Understanding the Protein Ladder and Molecular Interactions
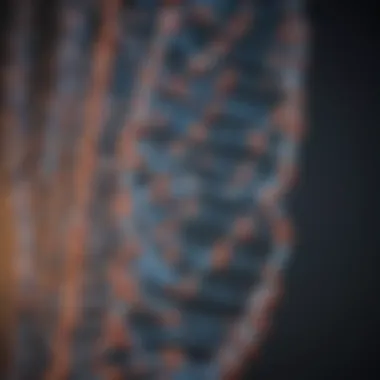
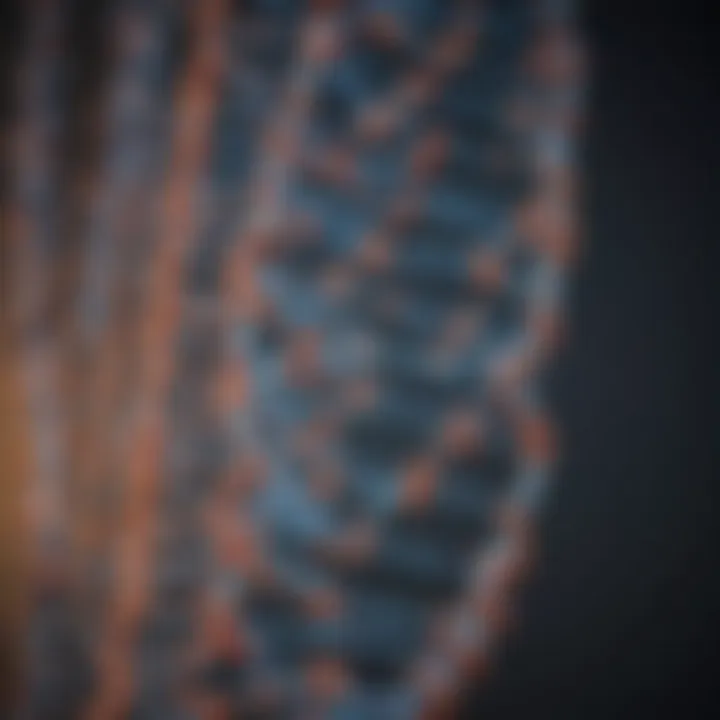
Intro
In the intricate realm of molecular biology, proteins serve as the essential building blocks of life. Whether we’re looking at enzymes facilitating metabolic processes or antibodies defending against pathogens, proteins are relentless workhorses in every living organism. Their significance cannot be overstated, as they participate in numerous biochemical interactions that sustain life. Understanding these interactions, particularly through the lens of the protein ladder, offers significant insights into the hierarchies and networks that govern cellular behavior.
The protein ladder provides a metaphorical framework illustrating the relationships and structures of proteins. As we explore this terrain, we will touch upon pivotal concepts such as protein folding and its implications for function, the experimental techniques employed to study these molecules, and the potential for novel drug development stemming from this knowledge. Ultimately, our journey seeks to paint a comprehensive picture of protein science, effectively bridging theoretical principles and cutting-edge research.
With this groundwork in place, let's delve into the key findings of recent studies that expand our understanding of protein interactions and their far-reaching implications.
Prelude to Proteins
In embarking on the journey of protein science, we must first grasp the essence and significance of proteins themselves. Proteins serve as the building blocks of life, playing multifaceted roles that extend into every biological process. These intricate molecules are crucial for a range of functions from catalyzing metabolic reactions to enabling cellular communication, thus underpinning the complexity and functionality of all living organisms. Without a doubt, understanding proteins takes center stage in our exploration of their interactions and structures within the protein ladder framework.
Proteins consist of long chains of amino acids, which fold into specific three-dimensional shapes that determine their function. Thus, the configurations they adopt are not merely arbitrary but intrinsic to their roles. A protein's structure significantly affects its behavior; for instance, enzymes require precise arrangements to efficiently catalyze reactions, while structural proteins, like collagen or keratin, provide stability and strength to tissues. These aspects make studying proteins an indispensable pursuit for researchers aiming to unlock the intricate workings of biological systems.
Moreover, the significance of proteins transcends individual functions; they work synergistically to form complex networks, akin to a well-orchestrated symphony where each musician, or in our case, each protein, plays their part. The ongoing research in protein interactions not only furthers our understanding of basic biology but also holds transformative implications for healthcare and therapeutics. As we delve further into the nuances of the protein ladder, a clearer picture of molecular interactions will surface, revealing how these connections can pave the way for advancements in drug development and treatment strategies.
Ultimately, exploring the realm of proteins equips us with tools to address real-world challenges, from disease treatment to bioengineering. By navigating through the components of the protein ladder, we set the groundwork for a more profound understanding, leading to potential innovations in various fields of scientific inquiry.
The Protein Ladder Concept
The concept of the protein ladder serves as an invaluable tool for researchers, educators, and students in dissecting the intricate world of protein interactions at a molecular level. By understanding how proteins are structured and how they influence biological functions, we gain critical insights that have far-reaching implications in fields such as biochemistry, molecular biology, and pharmacology.
In this framework, proteins can be seen as existing in different tiers or "rungs" of a ladder, each representing a specific level of complexity in their structure and functionality. This hierarchical concept brings forth several key benefits:
- Simplified Understanding: The protein ladder demystifies the complex relationships among proteins, aiding in the education of those who may be overwhelmed by the intricacies of molecular biology.
- Enhanced Research: By visualizing where a particular protein fits on the ladder, scientists can better predict interactions, fostering more informed hypotheses and research directions.
- Drug Development Insights: With the ladder as a guide, researchers can identify potential drug targets at specific levels of interaction, streamlining the drug discovery process.
"Understanding the structural hierarchy of proteins is akin to having a map in an uncharted territory; it guides us through a landscape filled with complexities."
As we delve deeper into the origins of this concept, its components, and effective visualization techniques, we'll see how the protein ladder not only elucidates molecular interactions but also paves the way for advancements in scientific research and practical applications.
Origins of the Idea
The idea of the protein ladder emerged from a growing realization of the significance of protein interactions within biological systems. Early studies focused on isolated proteins and their functions, but it soon became clear that proteins often work in concert, influencing cellular physiology in more complex ways.
One pivotal moment was the development of protein sequencing technologies in the 1950s, which allowed scientists to analyze and compare protein structures systematically. This opened the door to understanding how different proteins are related and how they interact in various biological pathways. Researchers began to visualize these relationships, leading to the ladder concept that represents the varying levels of interactions that shape cellular machinery and life itself.
Components of the Ladder
Each rung of the protein ladder encompasses distinct structural forms and interactions, all contributing to a grand tapestry of biological function. Here are the fundamental components:
- Primary Structure: This first rung represents the sequence of amino acids in a protein, akin to the alphabet in a language. The sequence dictates the protein's unique properties and eventual shape.
- Secondary Structure: Moving up, we encounter the formation of alpha helices and beta sheets. These structural motifs play a vital role in the overall folding and stability of proteins.
- Tertiary Structure: This level encompasses the three-dimensional arrangement of a single protein molecule. The interactions between various side chains lead to a well-defined shape critical for function.
- Quaternary Structure: At the top of the ladder lies the assembly of multiple protein subunits. This level is when proteins team up, forming larger complexes crucial for various cellular tasks.
Understanding these components aids in grasping how proteins not only function individually but also in harmony with other biomolecules.
Visualizing Protein Interactions
Visual representation of the protein ladder enhances comprehension significantly. A well-constructed diagram or model can shine a light on how different proteins fit together, reflecting their interactions and how these collaborations give rise to biological phenomena.
Various tools, such as molecular modeling software, allow for the creation of visual aids that can simulate these interactions. Here are some effective methods for visualizing protein interactions:
- Molecular Dynamics simulations: These provide a dynamic view of how proteins interact over time, offering insights into their behaviors in real-world conditions.
- Protein-protein interaction networks: Such network diagrams depict how different proteins interrelate, displaying a broader understanding of cellular processes.
- Hydrogen bond and hydrophobic interaction maps: Highlighting specific interactions gives a deeper insight into stability and function at various levels of the protein ladder.
The integration of visualization techniques not only aids in education but also fuels research by allowing scientists to hypothesize and understand protein behavior more clearly.
In essence, the protein ladder is much more than a theoretical construct; it is an essential framework that fosters a comprehensive understanding of molecular interactions, with implications extending into various disciplines ranging from fundamental research to drug discovery.
Protein Structure and Folding
In the realm of molecular biology, understanding protein structure and folding is pivotal to unraveling the intricate mechanisms that govern protein function. Proteins are not just linear chains of amino acids; they assume specific three-dimensional shapes that are crucial for their biological roles. The process of folding—a spontaneous event influenced by various factors—determines the functional capabilities of a protein and calls upon a wealth of interactions at the molecular level.
The importance of protein structure and folding extends into several core areas. For one, structural integrity is essential for enzymatic function. Enzymes, which facilitate biochemical reactions, rely heavily on having their active sites correctly positioned. Proteins that fold incorrectly may lead to loss of functionality and even disease. Consider the case of Alzheimer's disease where misfolded proteins aggregate and form plaques, disrupting cellular function.
Furthermore, the ability of proteins to interact with each other is dictated by their folding. The formation of complex quaternary structures often involves multiple polypeptide chains coming together, and this assembly can only occur if individual chains have folded properly. Protein interactions support cellular communication, immune responses, and a myriad of other biological processes. Thus, the study of protein structure and folding not only sheds light on basic life processes but informs practical applications in medicine and biotechnology.
Primary Structure
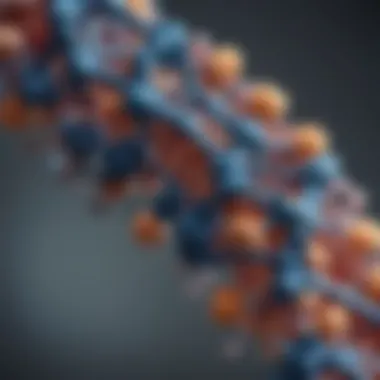
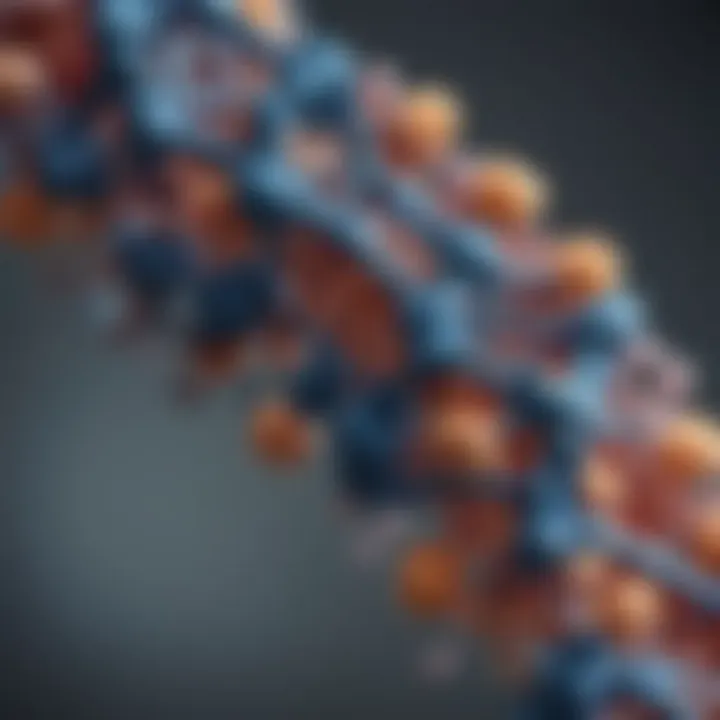
The primary structure of a protein is its linear sequence of amino acids. This sequence is a product of the genetic code, translating nucleotides into specific amino acids during protein synthesis. Each protein's identity and function stem directly from its primary structure, which dictates how the protein will fold into its three-dimensional form. The order of these amino acids matters significantly; even a single change can lead to profound effects on function.
For example, sickle cell anemia arises from a solitary substitution in the hemoglobin protein's primary structure. The altered amino acid leads to distinctive folding patterns that cause red blood cells to assume a sickle shape, which obstructs blood flow and results in numerous health complications.
"The primary structure is not merely a starting point; it is the very foundation upon which all other levels of protein architecture are built."
Secondary and Tertiary Structures
As a protein begins to fold, it generates secondary structures, which include alpha helices and beta sheets. These structures result from hydrogen bonds forming between nearby amino acids in the sequence. Alpha helices resemble spirals while beta sheets appear in pleated formations—both contribute to the protein's overall stability. These shapes help proteins maintain their functionality under various conditions.
The tertiary structure represents a further folding level, resulting from interactions between secondary structures. These interactions include hydrophobic forces, ionic bonds, and van der Waals forces, among others. It is this intricate three-dimensional conformation that allows proteins to interact with other molecules accurately. A crucial factor to note is the dynamic nature of these structures—many proteins can undergo conformational changes in response to environmental signals, thereby modulating their activities. This flexibility is critical for processes like allosteric regulation in enzymes.
Quaternary Structure
The quaternary structure is what occurs when multiple polypeptide chains come together to form a larger functional unit. Not all proteins achieve this level of complexity, but for those that do, it opens the door to a greater range of functions. Hemoglobin is an exemplary case, demonstrating how the quaternary structure enhances function. Made of four subunits, it uses cooperative binding to oxygen, a property that would be compromised if it were solely a monomer.
This cooperative behavior highlights the interplay between structure and function in proteins. The dynamics of how subunits come together not only affect oxygen transport but also how proteins interact with other cellular components, amplifying their roles in cellular machinery.
Biological Functions of Proteins
Biologically, proteins are the heavyweights of the molecular world; their functions are as diverse as they are critical to life. They serve multifaceted roles that are not only foundational to biological processes but also essential for cellular vitality. Understanding these functions offers insights into how proteins contribute to homeostasis in living organisms, their role in disease mechanisms, and their potential in biotechnological applications. Grasping the functions of proteins allows researchers to explore avenues such as targeted therapies, advanced drug design, and improved diagnostic techniques.
Enzymatic Activity
Proteins act as enzymes, catalyzing biochemical reactions that facilitate metabolic processes. They lower activation energy, enabling reactions to occur at a faster rate and under milder conditions than would be possible otherwise. Enzymatic activity is highly specific; each enzyme typically catalyzes a single type of reaction, influenced by its unique structure. An example of this is the enzyme lactase, which breaks down lactose into glucose and galactose. Individuals who produce insufficient amounts of lactase may develop lactose intolerance, highlighting how crucial enzymes are for metabolic health.
The efficiency and specificity of enzyme function can be affected by several factors, such as pH, temperature, and substrate concentration. Thus, the intricate balance within metabolic pathways relies heavily on the proper functioning of enzymes. Furthermore, researchers are harnessing this knowledge in biocatalysis for green chemistry—an approach that seeks to enhance reaction rates while minimizing environmental impact.
Structural Proteins
Not all proteins come to the party to react; some play the role of scaffolding, providing structural support to cells and tissues. Collagen, for instance, is the most abundant protein in the human body, making up a significant portion of our connective tissues, including skin, tendons, and cartilage. Its strength and flexibility allow tissues to withstand various stresses, thus contributing to overall structural integrity.
Structural proteins also facilitate cellular organization. For example, tubulin, a structural protein, forms microtubules that are essential for cell shape and transport within the cell. Actin, another key structural protein, plays a vital role in muscle contraction and cell motility. Together, these proteins not only sustain cellular architecture but also ensure that cells can respond appropriately to their environment. This aspect proves crucial in understanding conditions like muscular dystrophy, where structural proteins are impaired.
Signaling Molecules
In addition to their structural and catalytic roles, proteins are instrumental in communication within and between cells. Hormones, such as insulin, are proteins that regulate key physiological processes, including glucose metabolism. Insulin signals cells to absorb glucose, demonstrating how proteins can regulate energy balance and influence metabolic states.
Another critical aspect of protein signaling involves receptors, which bind specific signaling molecules. When a signaling molecule, like a hormone, binds to its receptor, it triggers a cascade of cellular responses. For instance, the binding of adrenaline to its receptor initiates a rapid increase in heart rate, preparing the body for a 'fight or flight' response. The intricate dance of protein interaction enables organisms to adapt to changes in their environment, underscoring the role of proteins in signaling pathways.
"Proteins are not just building blocks; they are also regulatory keys that unlock numerous biological mechanisms."
Epilogue
In summary, the biological functions of proteins are vital for life. From facilitating essential biochemical reactions to providing structural integrity and enabling communication between cells, proteins are at the forefront of molecular interactions that sustain biological processes. Understanding these functions leads to further discoveries in protein research, allowing for advancements in medicine, biotechnology, and our overall understanding of life at the molecular level.
By synthesizing our knowledge about enzymatic activity, structural roles, and signaling mechanisms, we can appreciate the intricate web of interactions that proteins partake in, illustrating their undeniable importance in the realm of biology.
Methods for Analyzing Proteins
The exploration of proteins, their structures, and functions is pivotal in the biological sciences. In this context, methods for protein analysis provide remarkable frameworks to dissect the complicated world of proteins. Grasping these methodologies equips researchers and practitioners with tools vital for understanding protein interactions and their implications in various biological processes. This section delves into specialized techniques that play a key role in protein analysis, illuminating their benefits and considerations.
Techniques for Protein Isolation
One of the first steps in any protein study is isolation, the process of separating proteins from other cellular components. Various strategies exist for achieving this, each with its own specific use and insights:
- Cell Lysis: This initial procedure involves breaking open cells to release proteins. Different lysis methods can be employed, depending on the cell type. For instance, mechanical disruption, like sonication and homogenization, can be used for tough tissues, while detergents may be ideal for softer cell lines.
- Centrifugation: After cell lysis, centrifugation is frequently used to separate proteins based on their mass and density. The result is a supernatant rich in protein components, ready for further purification.
- Precipitation Techniques: Ammonium sulfate precipitation is often utilized for an initial concentration of proteins. This method is particularly favorable due to its cost-effectiveness and simplicity.
- Affinity Chromatography: Tailored to bind specific proteins, usually through an antibody, this method enhances purity and specificity. Highly beneficial for targeting proteins of interest with minimal contamination from others.
While each method has its advantages, researchers must carefully consider which approach aligns best with their experimental goals. Different techniques come with varying degrees of yield, purity, and specificity, impacting downstream applications used in understanding the protein ladder.
Spectroscopy and Chromatography
Spectroscopy and chromatography serve as crucial methodologies for analyzing proteins, facilitating the determination of their concentration, purity, and structural properties. The synergy between these two approaches enhances the overall understanding of protein behaviors.
- UV-Visible Spectroscopy: Often employed for estimating protein concentration, UV-visible spectroscopy capitalizes on the absorbance of UV light by aromatic side chains of amino acids. It's straightforward but must be handled with care, as it doesn’t provide insight into structure or interactions directly.
- Fluorescence Spectroscopy: This advanced technique offers a wealth of information. By utilizing fluorescent tags, researchers can gain insights into protein folding, conformation, and interactions in live cells. The techniques allow for real-time observation, giving a dynamic view of proteins in action.
- High-Performance Liquid Chromatography (HPLC): When it comes to separating, identifying, and purifying proteins, HPLC shines. Its high resolutive power can distinguish between proteins that vary by just a few amino acids. It’s extensively used for both preparative and analytical purposes.
Combining these methodologies can significantly bolster research outcomes, helping delineate not just how proteins function individually but also how they interact within complex biological systems.
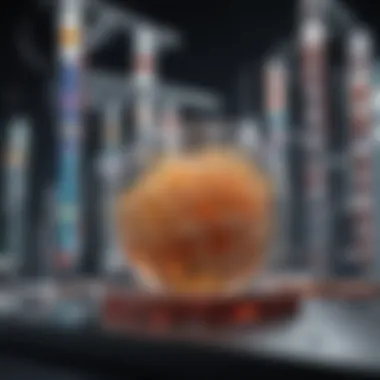
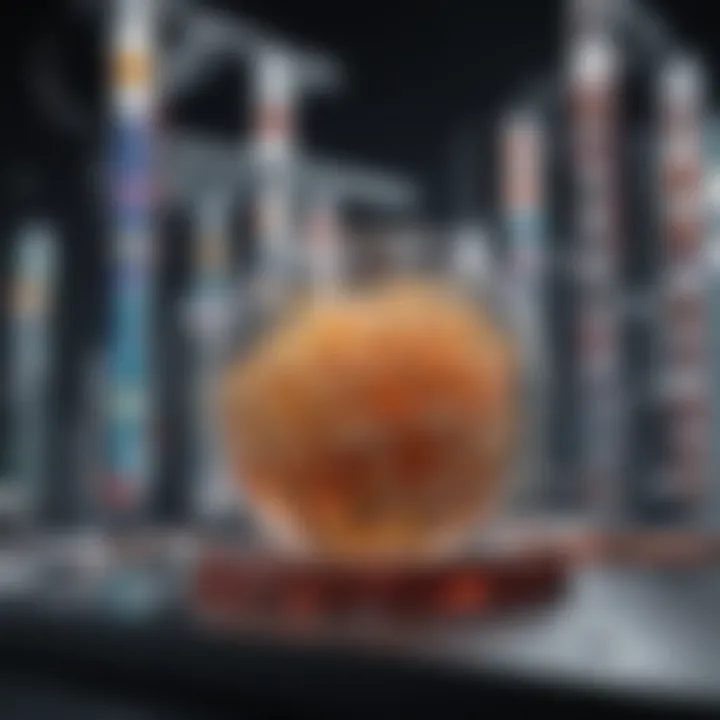
Crystallography in Protein Analysis
Crystallography stands out as a gold standard for determining the three-dimensional structures of proteins. Unlike other techniques, which might provide indirect measures, X-ray crystallography reveals atomic arrangements in exquisite detail. Key points to be noted include:
- Crystal Growth: The first hurdle often encountered is obtaining suitable protein crystals. Conditions must be meticulously optimized, striking a balance between promoting crystal growth and ensuring structural integrity.
- X-ray Diffraction: Once high-quality crystals are obtained, exposing them to X-ray beams allows for the collection of diffraction patterns. Interpreting these patterns provides insight into atomic positions within the protein structure.
- Data Interpretation: Utilizing complex computational algorithms, researchers can transform raw data from diffraction into real structural images. This step is crucial, revealing invaluable insights into how proteins function at a molecular level.
- Applications: Findings from crystallography can inform drug design and the development of therapeutics by elucidating target sites for binding interactions.
While successful crystallization and analysis are often seen as monumental feats, they unveil high-resolution structural details that open doors to broader scientific understanding, shaping not only basic biology but also translating into applied biomedical sciences.
Ultimately, the choice and implementation of analysis methods shape the breadth of knowledge gained from protein studies. With an arsenal of sophisticated techniques at their disposal, researchers are better equipped to tackle the complexities of protein interactions and their biological implications.
Implications for Drug Development
The study of proteins and their interactions holds significant value for drug development. Understanding the protein ladder allows researchers to comprehend how different proteins operate within biological systems. This can facilitate the design of therapies that target specific molecular interactions, ultimately leading to more effective treatments for various diseases. When drug developers grasp the nuances of protein behavior, they can manipulate these interactions to achieve desired therapeutic outcomes.
Targeting Protein Interactions
Targeting protein interactions is becoming a focal point in the design of modern drugs. By identifying protein-protein interactions, researchers can uncover potential drug targets that are critical for disease progression. For instance, in cancer research, certain proteins may interact abnormally, leading to uncontrolled cell growth. By inhibiting these interactions, drugs can effectively stop the growth signal. Moreover, protein interactions can also be modulated to improve drug efficacy.
Some specific approaches include:
- Competing Against Natural Ligands: Designing small molecules that fit into the same binding site as regulatory proteins can inhibit their activity.
- Allosteric Modulation: Targeting sites that are not the active site of a protein can regulate its activity. This allows for more nuanced control than straightforward inhibition.
- Biotherapeutics: Some therapies utilize engineered proteins to either enhance or disrupt specific interactions within cell signaling pathways.
This targeted approach not only has the potential to enhance drug specificity but also minimizes side effects, as it can reduce the chance of affecting other unrelated proteins.
Structure-Aided Drug Design
Structure-aided drug design capitalizes on the detailed understanding of protein structures to inform and guide the drug discovery process. Armed with knowledge about the 3D structure of proteins, scientists can design compounds that fit precisely within the protein's active sites or modulate its function.
The process generally follows a few key steps:
- Structural Characterization: Techniques such as X-ray crystallography and nuclear magnetic resonance (NMR) spectroscopy allow researchers to visualize protein structures in detail.
- Molecular Docking Studies: With structural data, scientists perform molecular docking studies to predict how small molecules might interact with a protein's active site. This provides a preliminary understanding of efficacy before synthesis.
- Combinatorial Chemistry: This method allows researchers to create libraries of compounds that can be screened against protein targets, optimizing the chances of finding effective drugs.
By considering not just the protein’s static structure but also dynamics—how that structure changes over time—researchers can better anticipate how a molecule will behave inside the body, leading to a more successful design process.
"As we unlock the complexities of protein interactions, we unlock the potential for groundbreaking therapeutic strategies that change lives."
Utilizing these methods can lead to the discovery of novel therapeutics that are tailored to the specific molecular machinery of diseases, enhancing both efficacy and safety profiles in drug candidates.
In summary, the implications for drug development stemming from understanding the protein ladder framework are profound. By honing in on protein interactions and leveraging structural insights, researchers are better equipped to develop innovative treatments that address unmet medical needs.
Proteins in Cellular Mechanisms
Proteins play an astonishingly intricate role in cellular mechanisms. Essentially, they are the workhorses of the cell, facilitating various processes that are crucial for life. Understanding how proteins contribute to cellular mechanisms allows for deeper insights into their functionality, efficiency, and potential implications in health and disease.
Cell Signaling Pathways
Cell signaling pathways are fundamental for communication within cells and between different cells. Proteins are crucial here, serving as messengers that transmit signals from the exterior environment to various internal processes. Think of it like navigating a bustling city where each intersection represents a signaling event, guiding the flow of traffic—except, in this case, it's about cellular responses.
The intricate dance of proteins in these pathways involves receptors, enzymes, and secondary messengers, each doing its part to ensure information reaches where it’s needed. For instance, when a hormone binds to its receptor, it triggers a cascade of events involving various proteins that can alter cellular behaviors, such as growth and metabolism.
Some key points to consider:
- Specificity: Each pathway is highly specific, meaning only certain proteins interact with each other.
- Modularity: Signaling pathways can be modular in nature, consisting of interchangeable components that allow cells to adapt to various stimuli.
- Temporal Dynamics: The timing of signaling events is critical. Proteins must be present at the right time and place to maintain coordinated responses.
"The role of proteins in cell signaling is akin to directors calling the shots in a complicated play, ensuring that every act unfolds at just the right moment."
Cell Cycle Regulation
In the realm of cell cycle regulation, proteins govern the intricate phases that each cell undergoes—essentially guiding the life cycle of the cell from division to death. This process is tightly controlled by a set of proteins known as cyclins and cyclin-dependent kinases (CDKs). Each combination serves as a checkpoint that determines whether a cell is ready to transition to the next phase, ensuring that only healthy cells replicate.
When the mechanisms governing these transitions falter, the consequences can be dire, potentially leading to uncontrolled growth and the formation of tumors. Hence, understanding the proteins involved in cell cycle regulation illuminates pathways to counteract diseases like cancer. Important aspects include:
- Checkpoints: Proteins monitor and regulate the cell cycle at checkpoints to prevent errors.
- Feedback Loops: Protein interactions often create feedback loops that can either promote or inhibit progression through the cycle.
- Repair Mechanisms: Certain proteins also play a role in repairing DNA damage that may arise during replication, ensuring cell integrity.
Apoptosis and Proteins
Apoptosis, or programmed cell death, is a vital process that removes unnecessary or damaged cells. Here, proteins play a two-edged sword role—they can promote cell survival or signal for destruction, maintaining the body's balance.
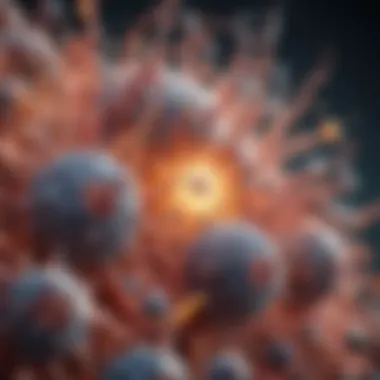
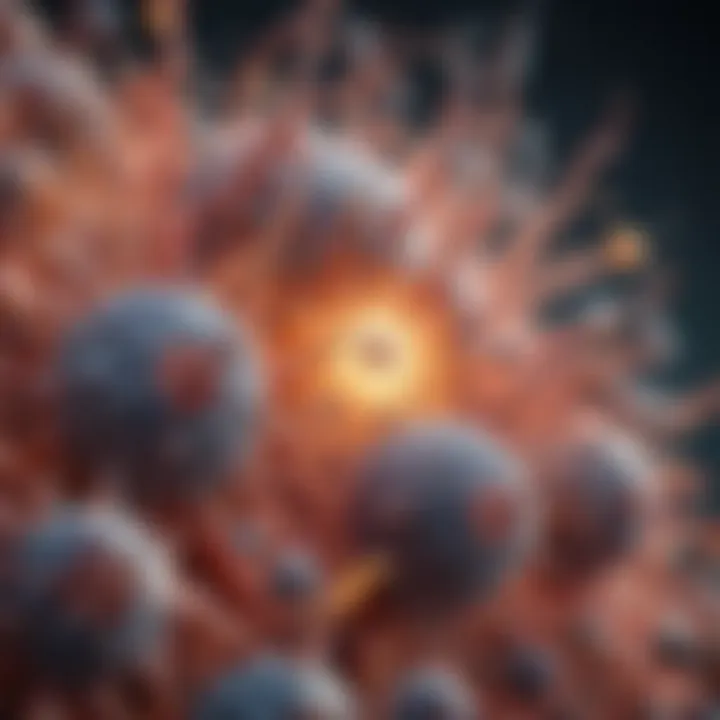
Key proteins involved in apoptosis include caspases, which act similarly to executioners, cleaving other proteins to execute cell death, and BCL-2 family proteins, responsible for feeding survival signals. This balancing act is crucial because a failure in apoptotic signaling can lead to diseases such as cancer or autoimmune disorders.
Considerations for apoptosis:
- Intrinsic vs. Extrinsic Pathways: Apoptosis can be triggered through internal (intrinsic) signals, often in response to cellular stress, or external (extrinsic) signals from other cells through receptor-ligand interactions.
- Importance of Homeostasis: The role of apoptosis in maintaining tissue homeostasis ensures that cell turnover occurs in a regulated manner, preventing disease manifestation.
- Potential Therapeutics: Understanding these pathways opens avenues for targeted therapies that might manipulate apoptosis—either to promote cell death in cancer cells or to rescue cells in degenerative diseases.
Challenges in Protein Research
The field of protein research, while replete with exciting possibilities, faces significant challenges that can complicate the understanding and application of protein science. Addressing these challenges is crucial for advancing our knowledge of molecular interactions, which in turn can impact everything from basic biology to drug development.
Protein Misfolding Disorders
Protein misfolding disorders represent a unique and critical challenge in the realm of protein research. At the heart of these disorders lies the failure of proteins to fold correctly, which can lead to disastrous consequences for cellular function. Conditions such as Alzheimer’s disease, Parkinson’s disease, and Huntington’s disease highlight just how catastrophic misfolding can be. In these diseases, misfolded proteins aggregate, forming toxic structures that disrupt normal cellular processes.
The study of these disorders not only broadens our understanding of protein functionality, but it also offers potential avenues for therapeutic intervention. For instance, understanding the molecular pathways leading to misfolding might enable scientists to design drugs that stabilize these proteins or facilitate their correct folding. Research on chaperone proteins—those that assist other proteins in achieving proper conformation—has also surged.
Despite these advances, several barriers persist. Research methodologies can be complex and costly, often requiring sophisticated techniques in cellular biology and biochemistry. Moreover, the variability between individual human genotypes further complicates the study of protein misfolding, as it may influence how proteins behave in different people, potentially skewing results and interpretations.
Experimental Limitations
While the exploration of protein dynamics and interactions has progressed, experimental limitations still pose significant hurdles. The intricate nature of protein structures makes it profoundly difficult to observe and analyze them in real-time. Techniques like X-ray crystallography and NMR spectroscopy, although valuable, come with their limitations. For example, X-ray crystallography requires crystallization, which can be a stumbling block for many proteins that refuse to form stable crystals. This limitation means that a wealth of proteins, crucial for understanding various biological processes, remain unexplored or inadequately characterized.
Another significant limitation is the scale of high-throughput screening methods. While these approaches have increased efficiency in identifying potential drug candidates, they often oversimplify the complex and dynamic environments in which proteins operate. Chemical libraries can contain thousands of compounds, but determining interactions and the functional relevance of each one in a cellular context requires a nuanced approach that high-throughput screening simply cannot provide.
Moreover, the reliance on in vitro studies has drawn some criticism. Many cellular mechanisms are context-dependent, and what works in a test tube may not have the same effects within living organisms. This disjunction leads to potential shortcomings in translating research findings into clinical applications.
"Understanding the limitations and challenges in protein research is not just an academic exercise; it is fundamental to addressing health issues on a global scale."
In summary, tackling the challenges in protein research is imperative for unlocking the full potential of protein science. As researchers navigate through the intricacies of protein misfolding disorders and experimental limitations, they pave the way for new insights and advancements that could impact how we understand, treat, and even prevent various diseases. This ongoing journey is essential for capitalizing on the promise that protein research holds for improving human health and advancing scientific knowledge.
Future Directions in Protein Study
As the realm of protein science continues to evolve, understanding future directions becomes paramount. The study of proteins is not merely an academic pursuit; it has significant implications across various fields such as medicine, biotechnology, and environmental science. This section articulates the ongoing trends and innovations that promise to reshape our comprehension of proteins, their functions, and their interactions.
Emerging Technologies
In recent years, technological advancements have been groundbreaking in protein research. The advent of high-throughput screening methods allows scientists to analyze numerous proteins simultaneously, leading to a better understanding of protein functions and interactions. Furthermore, techniques like CRISPR and genome editing have paved the way for precise modifications in protein coding, enabling researchers to explore the nuances of protein behavior.
- Cryo-electron microscopy (Cryo-EM) has gained traction. This method allows for the visualization of proteins in their native state, providing insights into their structure and dynamics without the need for crystallization.
- Mass spectrometry has also seen advancements, facilitating the detailed analysis of protein complexes, including post-translational modifications that influence protein function.
Moreover, the integration of AI and machine learning into protein research is a game changer. Algorithms can predict protein structure and stability based on sequence data, thus speeding up the research process. This computational power opens up new doors in drug design and understanding diseases linked to protein malfunctions. Researchers can simulate how new drugs will interact with target proteins, optimizing the development process significantly.
Interdisciplinary Approaches
The future of protein studies is not confined within the boundaries of biochemistry; rather, it's an interdisciplinary endeavor. Collaboration between biology, chemistry, physics, computer science, and even social sciences enriches the understanding of proteins.
- Biophysics provides deeper insights into the physical principles governing protein folding and stability, while computational biology facilitates predictive models that inform experimental strategies.
- Systems biology approaches encompass the complex interactions within cellular networks, accounting for various proteins' roles in these interactions.
- Furthermore, the incorporation of data science into protein research enhances the ability to interpret vast datasets generated by experimental techniques, allowing for more comprehensive insights.
In bringing these disciplines together, researchers are able to approach protein interactions from multiple angles, leading to holistic understanding. The synergy of interdisciplinary methods fosters innovation in treatments for diseases caused by dysfunctional proteins.
"As we bridge gaps between disciplines, our understanding of proteins and their roles in biological systems is becoming more coherent and multifaceted."
Finale
Bringing together the threads of discussion regarding the protein ladder, it’s crucial to recognize the implications this framework has on a multitude of scientific domains, particularly in understanding molecular interactions. The protein ladder serves not merely as an academic pursuit but as a pivotal tool that sheds light on the complexity of protein behavior in biological systems. At a glance, its significance can be boiled down to a few key aspects:
- Holistic Understanding: By visualizing proteins in a hierarchical structure, researchers gain a more comprehensive understanding of how proteins interact with one another. This understanding is fundamental to decoding cellular processes ranging from metabolism to signal transduction.
- Drug Development Applications: The insights gleaned from studying the dynamics of the protein ladder aid pharmaceutical companies immensely. Clarity about protein-protein, protein-ligand interactions helps in the design and optimization of drugs, enhancing their efficacy and reducing side-effects.
- Educational Framework: For students and educators in the field of biochemistry, the protein ladder is an invaluable educational tool. It encapsulates complex reactions and interactions simply yet effectively, making it accessible for learners at various levels.
Recap of Key Points
To summarize the key insights discussed in the article:
- Definition of Proteins: Proteins are intricate molecules essential for life, functioning in nearly every biological process.
- Protein Ladder Concept: This framework offers a structured way to understand molecular interactions, emphasizing the hierarchical nature of protein relationships.
- Structural Insights: The article highlights the various levels of protein structure, from primary to quaternary, and their impact on functionality.
- Biological Roles: Proteins serve multifaceted roles such as catalysts, structural components, and molecular signals in a cellular context.
- Analytical Techniques: Methods used to analyze protein interactions are critical for advancing our understanding of these biomolecules.
- Future Directions: Emerging technologies and interdisciplinary approaches promise great potential for further discoveries in protein research.
By synthesizing these elements, readers can appreciate not only the complexity of protein science but also the novel applications that stem from it.
Importance of Ongoing Research
Continued research into protein interactions remains vital as it fuels advancements across various disciplines. The nature of science is ever-evolving, and the need for deeper insights into molecular interactions is pressing. Here are a few considerations surrounding ongoing research:
- Addressing Global Health Issues: Research into proteins can directly impact our approach to diseases, including cancer and neurodegenerative disorders. Understanding protein dysfunction opens pathways for innovative therapies.
- Innovations in Biotechnology: With the rise of synthetic biology and biotechnology, research into proteins can yield synthetic proteins designed for specific functions, potentially revolutionizing industries such as agriculture and medicine.
- Collaborative Efforts Across Fields: As the boundaries between fields blur, interdisciplinary approaches help develop novel methodologies and insights. A collaborative environment encourages scientists from varied backgrounds to address complex biological questions collectively.
Advancing the study of the protein ladder and enhancing our understanding of protein interactions is not merely an academic exercise; it's a crucial endeavor that touches upon the very fabric of life and health.