Exploring Techniques in Single Cell Sequencing
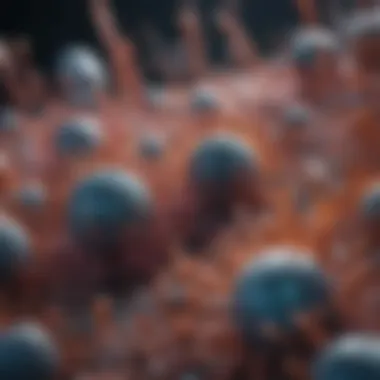
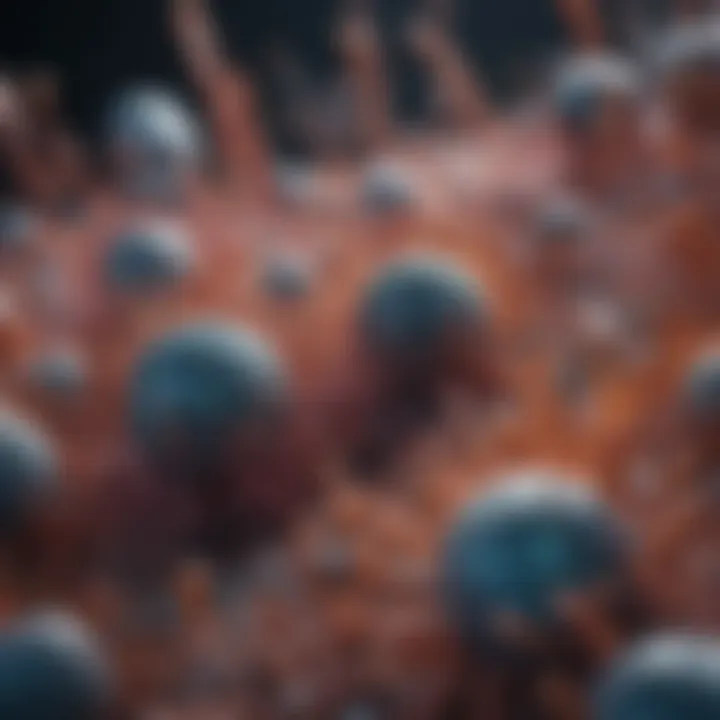
Intro
Single cell sequencing is becoming a pivotal method in modern biological research. This innovation allows researchers to dissect complex biological systems at an unprecedented resolution. By examining individual cells, scientists can understand gene expression patterns, genomic variations, and how these factors contribute to health and disease.
The capability to analyze genetic data from single cells provides insights that bulk sequencing methods simply cannot deliver. As we look forward to the potential of these techniques, it is crucial to appreciate the complexities and nuances involved.
Research Overview
Summary of Key Findings
Through extensive studies in the field, several key findings have emerged:
- Enhanced Resolution: Single cell sequencing allows for a more detailed view of cellular heterogeneity compared to traditional methods.
- Applications Across Fields: This technique is not confined to genomics alone; its applications extend to cancer research, immunology, and developmental biology.
- Technological Advances: Innovative platforms such as 10x Genomics' Chromium and Fluidigm’s C1 have streamlined workflows, enabling more comprehensive analyses.
Research Objectives and Hypotheses
This article aims to:
- Evaluate the different methodologies employed in single cell sequencing.
- Investigate how these methods can be applied in various biological contexts.
- Discuss challenges such as data complexity, cost-effectiveness, and reproducibility.
The central hypothesis is that advancements in single cell sequencing technologies will continue to enhance our understanding of biological processes, thus providing pathways for novel therapeutic strategies.
Methodology
Study Design and Approach
A systematic examination of the current methodologies in single cell sequencing is necessary to understand its potential. The research encompasses:
- Literature Review: Analyzing existing publications on methodologies and applications of single cell sequencing helps frame the current state of the field.
- Comparative Analysis: Evaluating the strengths and weaknesses of different platforms will be central to identifying best practices.
Data Collection Techniques
Data collection employs various techniques:
- Cell Isolation Methods: Techniques such as microfluidics and laser capture microdissection are pivotal.
- Sequencing Technologies: Next-generation sequencing (NGS) plays a critical role. Technologies like Illumina and PacBio are commonly utilized.
- Data Processing: Bioinformatics tools, including Seurat and Scanpy, enable handling and analysis of the complex datasets generated.
The results obtained from these methodologies provide a nuanced understanding of cellular function and variability, a necessity for tackling contemporary bioscience challenges.
Prelude to Single Cell Sequencing
In the landscape of biological research, single cell sequencing has emerged as a pivotal advance. It allows researchers to dissect the complexities of cellular behavior in unprecedented detail. Past methods primarily offered insights into populations of cells. However, this approach often masked the rich heterogeneity present within these populations. Single cell sequencing, in contrast, focuses on individual cells, unveiling the subtle distinctions in gene expression and genomic variations that are often overlooked.
This capability is not merely a refinement of existing methods; it represents a paradigm shift. By enabling the understanding of cell-to-cell variations, scientists can uncover new insights into cellular functions, disease mechanisms, and developmental processes. It opens doors to explore the unique identities of cells in diverse contexts, ranging from cancer biology to neuroscience.
Moreover, the importance of this technique transcends its technical prowess. It serves as a powerful tool that augments our capacity to construct comprehensive cellular maps of organisms. This aids in understanding the architecture of tissues and organs.
There are several key benefits inherent in single cell sequencing:
- Customized Approaches: Different cells may respond uniquely to stimuli. Single cell sequencing allows for tailored analyses according to the specific needs of research objectives.
- High-resolution Insights: Fine details about cellular states and transitions can be explored, which is crucial for advancing personalized medicine.
- Discovery Potential: Researchers may identify novel cellular subtypes and interactions, which can lead to breakthrough findings in various fields.
However, engaging with single cell sequencing is not without its considerations. Technical challenges such as low yield of genetic material and data complexity can pose hurdles. Additionally, proper interpretation of the data is essential to derive meaningful conclusions.
"Single cell sequencing can transform our understanding of biology, but it requires careful application and thoughtful interpretation."
As we delve deeper into this article, we will explore various methodologies, illuminate the applications across disciplines, and discuss the limitations that come with this powerful technology. Single cell sequencing thus stands at the cusp of revolutionizing how we view biological systems, paving the way for novel discoveries and innovations in science.
Historical Context
The history of sequencing technologies provides a crucial backdrop for understanding single cell sequencing. This exploration is essential because it highlights the evolution of methodologies that have revolutionized biological research. Recognizing the historical context allows researchers to appreciate the advancements leading to current practices and the potential future developments.
Evolution of Sequencing Technologies
Sequencing technologies began to take shape in the late 1970s with the introduction of methods like Sanger sequencing. This basic technique set the stage for more complex technologies in the years that followed, including the development of next-generation sequencing (NGS). NGS provided a parallelized approach, allowing for the rapid sequencing of entire genomes. The transition from Sanger sequencing to NGS marked a significant shift in genomics, enabling the analysis of large datasets efficiently.
The drive for faster, cheaper, and more accurate sequencing led to the refinement of these technologies. Advances such as massively parallel sequencing have introduced remarkable speed and cost-effectiveness in the sequencing workflow. Today, these innovations support the intricate demands of single cell sequencing. As sequencing has become more accessible, the ability to analyze individual cells rather than bulk populations has opened new avenues in various disciplines.
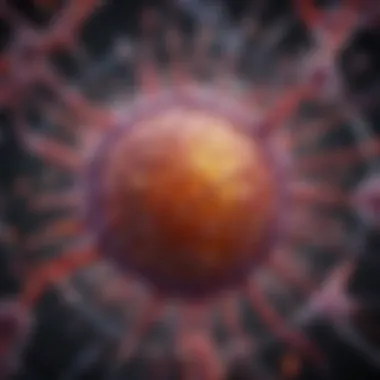
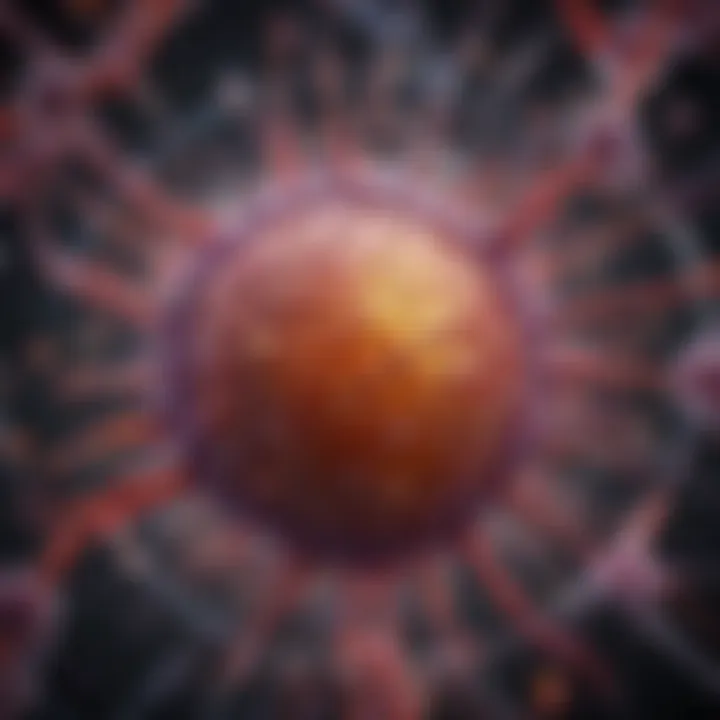
The Emergence of Single Cell Analysis
The concept of single cell analysis emerged as researchers recognized cellular heterogeneity as a vital aspect of biological systems. Earlier genomic techniques assessed average profiles—obscuring the variability present within cell populations. This lack of resolution could mask critical biological insights.
The introduction of single cell sequencing was a pivotal moment. This approach allows scientists to dissect complex tissues and understand cellular diversity at an unprecedented resolution. Single cell RNA sequencing, for example, enables the study of transcriptomic differences between cells, leading to discoveries in fields such as cancer research and developmental biology.
This analytical capability has shifted perspectives in biomedical research. It underscores the importance of individual cellular context, reinforcing the notion that cellular environments significantly impact gene expression and cellular fate.
Fundamental Concepts in Single Cell Sequencing
Single cell sequencing is a revolutionary approach in genomics and molecular biology that allows researchers to investigate cellular diversity in great detail. The fundamental concepts underpinning this area are crucial for understanding its impact on contemporary bioscience. This section emphasizes two primary aspects: cell heterogeneity and the importance of single cell resolution.
Cell Heterogeneity
Cell heterogeneity refers to the differences between individual cells within a population. It plays an essential role in various biological processes and diseases. Traditional bulk sequencing methods average the genetic information from a mix of cells. Consequently, important details regarding distinct cell types can be masked.
Studies have shown that even genetically identical cells can exhibit variability in gene expression, protein levels, and cellular responses. Recognizing this heterogeneity is vital in contexts such as cancer research, where distinct tumor cell populations may respond differently to treatments.
Understanding cell heterogeneity involves several layers:
- Diverse Cell Types: Different cell types possess unique functions that contribute to the overall organism.
- Microenvironment Influence: The local cellular environment can impact how individual cells behave or respond.
- Cellular States and Dynamics: Cells can exist in various states, influenced by developmental stages or stress responses.
With single cell sequencing, researchers can dissect these differences. This technique provides insights into how individual cells contribute to broader biological phenomena.
Importance of Single Cell Resolution
Single cell resolution is a key advantage of single cell sequencing techniques. Achieving this resolution allows scientists to observe and quantify gene expression or genomic variations at the level of individual cells. In systems where cellular dynamics are complex, such as the immune system or developing embryos, the ability to analyze single cells offers several benefits:
- Refinement of Data: Single cell data is richer. It enables researchers to identify subpopulations and understand their specific roles.
- Improved Diagnostic Potential: In medical applications, assessing individual cell behavior can lead to earlier and more accurate disease detection.
- Targeted Therapy Development: Understanding which cell types respond to specific treatments can enhance therapeutic personalization.
As the field progresses, the techniques that achieve this resolution continue to evolve. The combination of single cell resolution and the awareness of cell heterogeneity creates a comprehensive framework for both basic research and clinical applications.
By examining individual cells, we unlock data that traditional methods often overlook, revealing intricate details essential for understanding biological systems.
Thus, grasping these fundamental concepts is indispensable for comprehending the scope and implications of single cell sequencing in advancing knowledge within biosciences.
Major Techniques in Single Cell Sequencing
Single cell sequencing has transformed our understanding of biological systems. This section will explore key techniques used in this realm. Each methodology has its own distinct advantages. Understanding these techniques is crucial for researchers aiming to harness the full potential of single cell analysis in their studies.
Single Cell RNA Sequencing (scRNA-seq)
Single Cell RNA Sequencing, or scRNA-seq, is a predominant technique for analyzing gene expression at the single-cell level. This approach provides insights into cellular functions and heterogeneity. Unlike bulk RNA sequencing, which averages gene expression across many cells, scRNA-seq uncovers the variation in gene expression among individual cells.
The workflow typically begins with the isolation of individual cells, often done through microfluidics or manual pipetting. After isolation, mRNA from each cell is captured and reversed transcribed into cDNA. The library is then prepared for sequencing. Data from this process reveal the transcriptome profile of each cell. One key advantage of scRNA-seq is its ability to identify rare cell populations that might be overlooked in bulk analyses.
However, it is not without limitations. scRNA-seq can be sensitive to the quality of RNA, and technical variability may influence results. Despite these challenges, its application spans many disciplines, including developmental biology and oncology.
Single Cell DNA Sequencing (scDNA-seq)
Single Cell DNA Sequencing, or scDNA-seq, focuses on genomic variations at the cellular level. This technique is critical for studying tumor heterogeneity and evolutionary dynamics. By analyzing the DNA of individual cells, researchers can gain insights into mutations, copy number variations, and structural alterations.
The process involves similar steps to scRNA-seq. After isolating individual cells, genomic DNA is extracted. Amplification techniques like whole-genome amplification are commonly used, as the initial DNA amount is low. Following amplification, libraries are prepared, and high throughput sequencing is performed.
A significant benefit of scDNA-seq is its capacity to elucidate genetic diversity within a population. In cancer research, this helps in understanding how different clones evolve. Nonetheless, challenges include bias in amplification techniques, which might skew results.
Single Cell ATAC Sequencing (scATAC-seq)
Single Cell ATAC Sequencing, abbreviated as scATAC-seq, provides unique insights into chromatin accessibility at the single-cell level. This technique is pivotal in understanding gene regulation, as it detects open chromatin regions associated with active enhancer and promoter elements.
The workflow begins with nuclei isolation. Once isolated, the ATAC-seq protocol uses Tn5 transposase to integrate sequencing adapters into accessible regions of the genome. Afterward, the sample undergoes sequencing. The resulting data reveal not just which genes might be expressed, but also the regulatory landscape governing gene expression.
A key advantage of scATAC-seq is its ability to complement scRNA-seq, providing a more comprehensive view of cellular function. Nevertheless, scATAC-seq is sensitive to the quality of the starting material, which can affect reproducibility and reliability.
Spatially Resolved Sequencing Approaches
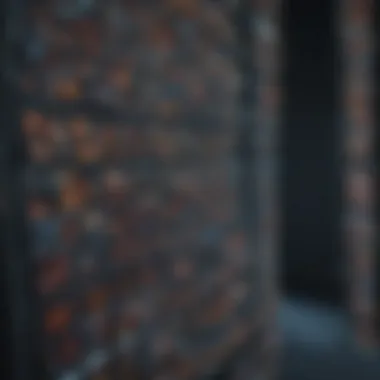
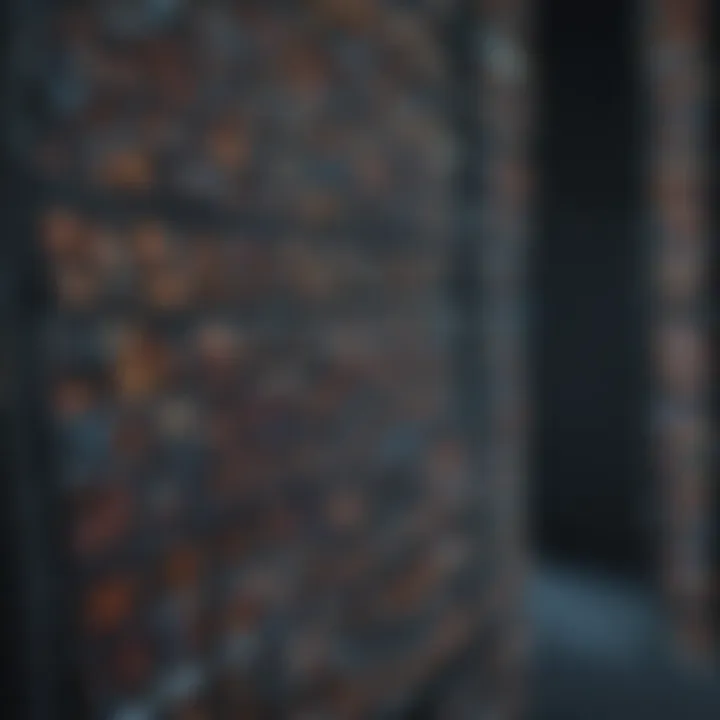
Spatially resolved sequencing approaches combine the power of high-resolution imaging with sequencing techniques. Methods such as Spatial Transcriptomics allow for the analysis of gene expression in the context of tissue architecture. This approach is crucial for understanding the microenvironment and interactions between cells.
The core concept involves capturing transcriptomic data while preserving the spatial context of the tissue. Typically, tissue sections are placed onto a slide with spatially barcoded capture oligonucleotides. After hybridization of RNA molecules, sequencing allows for a spatial readout of gene expression.
This technique provides an unparalleled view of cellular interactions and spatial dynamics, offering insight particularly relevant in tumor microenvironments and developmental biology. However, high costs and complex data analysis can pose limitations for broader adoption.
Understanding the diverse major techniques in single cell sequencing is essential for harnessing their potential in research across various fields.
In summary, each technique contributes uniquely to single cell sequencing. scRNA-seq excels in gene expression analysis, scDNA-seq uncovers genomic variations, scATAC-seq reveals regulatory elements, and spatially resolved approaches help visualize gene expression in the context of tissue architecture. The continued development and integration of these methodologies will further enhance our understanding of complex biological systems.
Workflow of Single Cell Sequencing
The workflow of single cell sequencing is an essential component of this technique, serving as the backbone for ensuring accuracy and reliability in the analysis of individual cells. The meticulous organization of this process allows researchers to uncover the remarkable diversity present in populations of cells. Each step, from sample preparation to data analysis, requires precision and careful execution. It highlights the significance of maintaining the integrity of delicate single cell material while also ensuring that the resultant data is robust for interpretation.
A structured approach to this workflow helps in addressing key elements such as the efficiency of cell isolation, the quality of sequencing libraries, the choice of sequencing techniques, and the methods of data interpretation. Below, each critical phase of the workflow is explored in detail:
Sample Preparation
Sample preparation is the foundational step in single cell sequencing. It involves isolating individual cells from a tissue or culture, which can be challenging due to the need to discern cells from their environment without altering their properties. Improper sample preparation may lead to contamination, loss of cell viability, or an inaccurate representation of the cellular composition:
- Cell Isolation Methods: Techniques such as microfluidics, laser capture microdissection, or manual pipetting are commonly employed. Each method has its advantages depending on the source material.
- Considerations for Viability: It is crucial to minimize cell stress and maintain viability throughout handling, as damaged cells may lead to misleading expression profiles.
Library Construction
Library construction is the subsequent phase, where the isolated RNA or DNA from each single cell is converted into a library suitable for sequencing. This process is pivotal as it determines the efficacy of the sequencing run:
- Protocols and Kits: Here, researchers may opt for specialized kits tailored for single cell applications, which may simplify the workflow while ensuring high yield.
- Duplication and Bias: Implementing appropriate techniques reduces biases that might distort gene expression data, allowing for a more accurate narrative of the transcriptome or genome of each cell.
Sequencing Techniques
The choice of sequencing technique directly impacts the quality of the data obtained from single-cell analyses. Current technologies cater to both RNA and DNA sequencing:
- Next-Generation Sequencing (NGS): NGS is the predominant method used, offering the ability to process thousands of cells in parallel, generating extensive data rapidly.
- Targeted Sequencing Approaches: In some scenarios, targeted sequencing of specific regions might be viable, particularly in applications that aim to resolve particular gene mutations in diseases like cancer.
Data Analysis and Interpretation
Data analysis is often regarded as one of the most complex aspects of the workflow. This phase transforms raw data into biologically meaningful information:
- Bioinformatics Tools: Various software platforms exist for analyzing single cell sequencing data, such as Seurat or Scanpy. These tools provide powerful functionalities for clustering, differential expression analysis, and visualization.
- Interpretative Challenges: Interpreting the results requires a nuanced understanding of both biological context and statistical methods. High complexity in the data can lead to information overload unless carefully parsed.
The streamlined workflow of single cell sequencing is fundamental for advancing biological insights, allowing researchers to dive deeper into the cellular complexity unseen in bulk analysis.
In summary, a thorough understanding of the workflow of single cell sequencing is vital for leveraging its full potential. Each stage, from sample preparation to data analysis, plays a significant role in shaping the outcomes of research studies and enriching our knowledge about cellular behaviors.
Applications of Single Cell Sequencing
Single cell sequencing stands as a pivotal tool in contemporary bioscience. Its ability to provide insights at the cellular level has transformed various fields of research. The understanding of complex biological systems is enhanced significantly by analyzing the unique characteristics of individual cells. While many sequencing techniques examine tissues or bulk samples, analyzing single cells reveals heterogeneity that is often masked. This precision allows researchers to discern subtle biological variations and functions.
Cancer Research
In cancer research, single cell sequencing plays a crucial role in decoding tumor complexity. Tumors are not composed of uniform cells; they exhibit significant cellular diversity. By applying single cell RNA sequencing (scRNA-seq), researchers can identify distinct cell populations within tumors. This enables the study of tumor microenvironments and the interactions between cancer cells and surrounding normal cells.
Furthermore, scDNA-seq helps to understand genetic variations present in individual tumor cells. This is vital for comprehending mechanisms that drive cancer progression and resistance to treatment. The identification of unique mutational signatures can inform therapeutic strategies and personalized medicine approaches.
Developmental Biology
Single cell sequencing enhances our understanding of developmental processes. During embryogenesis, cells undergo extensive differentiation. By studying single cells from various stages of development, researchers can map cell lineage relationships and track gene expression changes over time. This is essential for identifying key regulatory genes and pathways involved in normal development.
Moreover, insights gained from single cell analysis can also shed light on developmental disorders. By comparing normal and affected tissues at a single cell level, differences can be pinpointed, leading to a better understanding of underlying mechanisms.
Immunology
In immunology, the application of single cell sequencing has opened new avenues in understanding the immune response. The immune system comprises diverse cell types, each with distinct functions. scRNA-seq facilitates the identification and characterization of individual immune cells, revealing functional states during infections or diseases.
This detailed analysis can inform vaccine development and therapeutic interventions. For example, understanding T cell responses in infections or tumors can lead to improved strategies in immunotherapy. The ability to profile immune cells at a single cell level also aids in identifying biomarkers for autoimmune diseases.
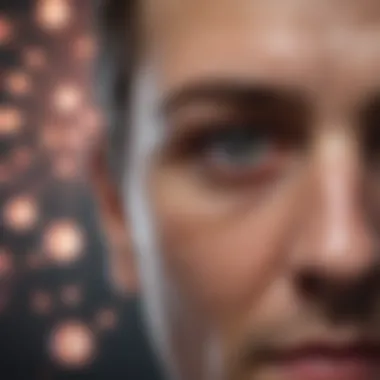
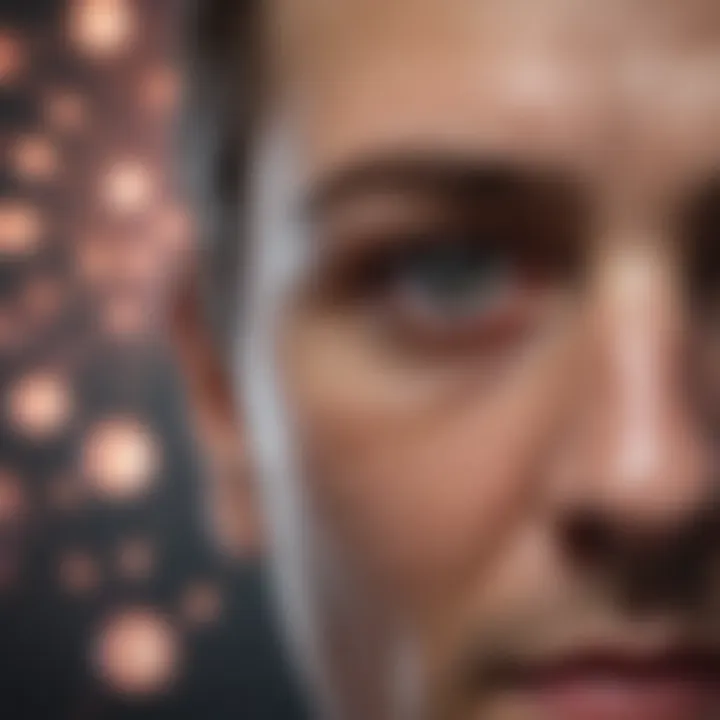
Neuroscience
The field of neuroscience benefits greatly from single cell sequencing as it allows researchers to explore the complexity of the brain. Neuronal diversity is critical for understanding brain function and disorders. Single cell RNA sequencing can uncover different neuron types and their specific gene expression profiles.
Additionally, this approach aids in identifying changes in the brain related to neurological diseases, such as Alzheimer’s or Parkinson’s. By examining the spatial distribution and functional states of neurons, scientists can better understand disease mechanisms. The potential for developing targeted therapies is enhanced through these insights, contributing to the advancement of personalized approaches in treating neurological disorders.
Challenges in Single Cell Sequencing
The field of single cell sequencing (SCS) has made significant strides in unlocking the cellular mysteries central to biological research. However, it is not without its challenges. Understanding these challenges is crucial for researchers and practitioners looking to utilize these techniques effectively. They encompass a variety of technical, data-related, and ethical considerations that can influence the success and reliability of single cell studies.
Technical Limitations
One of the foremost challenges in single cell sequencing is the technical limitations inherent to the methods used. Early single cell techniques suffered from low sensitivity and high background noise, leading to unreliable data. Although advancements have been made, such as the development of microfluidics and droplet-based platforms, some limitations persist. For instance, single cell RNA sequencing (scRNA-seq) can miss lowly expressed transcripts due to amplification biases during library preparation. This can result in incomplete or misleading representations of the transcriptome.
Moreover, the efficiency of cell capture has a direct impact on the quality of sequencing data. Loss of cells during the process can lead to a biased sample that fails to accurately represent the original population. Variability in cell isolation techniques, such as laser capture microdissection or fluidic devices, also presents challenges that can affect reproducibility across studies.
Data Complexity and Interpretation Issues
Single cell sequencing generates vast amounts of data, posing significant complexities in data management and analysis. The high-dimensional nature of this data complicates straightforward interpretation. Researchers must navigate through noise and biological variability, which can obscure meaningful signals.
To address these issues, advanced computational methods, like dimensionality reduction techniques, are often employed. However, these methods may introduce their own biases or assumptions about the data structure. For example, clustering algorithms are used to identify distinct cell populations, but their effectiveness greatly depends on the input parameters and the original dataset quality.
Additionally, integrating data from various sources or types—such as RNA and spatial transcriptomics—adds another layer of complexity. This necessitates sophisticated algorithms capable of harmonizing different datasets, reinforcing the importance of expertise in bioinformatics.
Ethical Considerations
As with any scientific advance, single cell sequencing raises ethical considerations that cannot be ignored. The ability to analyze individual cells poses risks concerning privacy, especially in human studies. Potential misuse of genetic information could lead to discrimination in areas such as employment or insurance.
Furthermore, there are concerns related to consent. Participants may not fully understand the extent of data that will be generated or how it might be used. Hence, obtaining informed consent becomes more complex in these scenarios. Researchers must ensure transparency regarding the implications of sequencing data gathering and storage.
Importantly, regulations and guidelines governing the use of genetic information in research are still evolving. This means that ethical compliance must be continually assessed and updated to protect subjects involved in research.
The challenges in single cell sequencing require an interdisciplinary approach, combining insights from biology, technology, and ethics to navigate the future of this promising field.
These elements collectively highlight the importance of continuing education, rigorous methodology, and ethical oversight in the application of single cell sequencing techniques. Addressing these challenges is crucial for the growth of the field, allowing researchers to unlock its full potential.
Future Directions in Single Cell Sequencing
The exploration into future directions in single cell sequencing offers a glimpse into how advancements will significantly refine and extend our understanding of biological systems. This area is essential, given the evolving nature of scientific inquiry, which increasingly demands fine-resolution insights into cellular heterogeneity, disease mechanisms, and treatment responses. The capabilities afforded by single cell technologies are reshaping the landscape of research, every advancing step enhancing both academic studies and clinical applications.
Integrative Approaches
Integrative approaches refer to the combination of different single cell sequencing methodologies to obtain more comprehensive data. By merging single cell RNA sequencing with single cell ATAC sequencing, for instance, researchers can analyze gene expression alongside chromatin accessibility within the same cells. This correlation can yield critical insights into gene regulation at a single-cell level, leading to improved understanding of disease mechanisms. Additionally, leveraging multi-omics strategies, which combine transcriptomics, proteomics, and metabolomics, offers an enriched view of complex biological phenomena. The integration of spatial data, such as from spatial transcriptomics, is also being explored to provide context to single cell analyses, allowing researchers to maintain the spatial information of cells in tissue samples.
Advancements in Technology
The technology underpinning single cell sequencing is rapidly improving. Innovations like the development of high-throughput sequencing platforms significantly reduce costs and increase the speed of data generation. Companies such as 10x Genomics and Illumina are pushing the boundaries of what is possible. Moreover, enhancements in microfluidics and automation streamline sample preparation, making it more accessible for laboratories with varying levels of technical expertise. Another remarkable advancement includes the introduction of scalable single-cell isolation techniques, allowing scientists to analyze thousands of cells simultaneously without losing resolution. These technologies not only improve the efficiency of obtaining single cell data but also enhance the quality, paving the way for more detailed investigations into cell behavior and properties.
Impact on Personalized Medicine
The implications of single cell sequencing extend into the realm of personalized medicine, wherein treatments can be tailored to the genetic and epigenetic landscape of an individual's cells. Understanding cellular variations assists in identifying biomarkers for diseases, leading to improved diagnostic and therapeutic strategies. For patients with cancer, single cell analyses can reveal tumor heterogeneity and enable the development of more targeted therapies. Furthermore, tracking cellular responses to treatments at a granular level can help in personalizing follow-up care. By providing insights into how specific cells react to clinical interventions, single cell sequencing stands to bridge the gap between laboratory findings and practical applications in patient care, ultimately enhancing outcomes and quality of life.
"The future of single cell sequencing holds the potential to revolutionize our understanding of biology and disease, making it a cornerstone of modern biomedical research."
As single cell sequencing technologies evolve, the integration of diverse methodologies, technological advancements, and the focus on personalized medicine will undoubtedly drive the next wave of discoveries. This progression not only emphasizes the illumination of cellular dynamics but also reinforces the significance of continuous innovation within this groundbreaking field.
The End
The conclusion of this article plays a pivotal role in encapsulating the intricacies of single cell sequencing techniques discussed. This segment synthesizes insights from various sections, emphasizing why this field has gained such prominence in contemporary bioscience.
Single cell sequencing is impactful because it allows for the dissection of cellular heterogeneity, which is vital in understanding complex biological systems. The ability to analyze gene expression and variations at the individual cell level unlocks new dimensions of research, offering a clearer view of biological processes that bulk analysis methods often obscure.
Summary of Key Insights
The key insights highlighted throughout this article serve to reinforce the significance of single cell sequencing:
- Technological Advances: Various methodologies like Single Cell RNA Sequencing (scRNA-seq) and Single Cell DNA Sequencing (scDNA-seq) illustrate the technological evolution that enables accurate data collection.
- Broad Applications: Single cell techniques have transformed fields like cancer research, immunology, and developmental biology. Each domain benefits uniquely from the capability to analyze cells individually, leading to more precise and targeted therapies.
- Challenges and Limitations: While the advantages are substantial, the hurdles such as technical limitations, ethical considerations, and the complexity of data are noteworthy. Awareness of these challenges is crucial for responsible application in research and clinical settings.
Implications for Future Research
Looking ahead, the implications for future research in single cell sequencing are profound. As the field continues to advance, several trajectories stand out:
- Integrative Approaches: Combining single cell sequencing with other omics technologies will enhance our understanding of cellular mechanisms.
- Personalized Medicine: The potential for tailored therapies based on individual cellular profiles could revolutionize treatment strategies in various diseases.
- Ethical Frameworks: Establishing solid ethical guidelines will be essential to navigate the complexities associated with genomic data derived from single cell analysis.