Exploring the Properties of Graphite
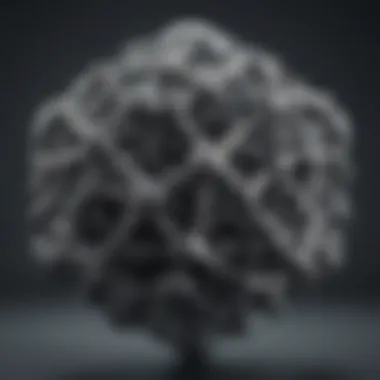
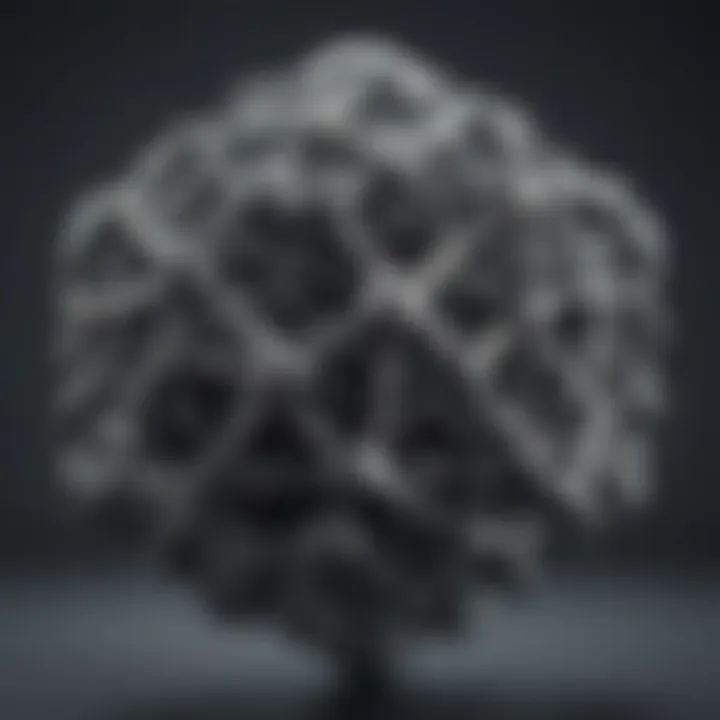
Intro
Graphite is often viewed as just another form of carbon. While that may be true on a surface level, diving deeper reveals a material of extraordinary complexity and versatility. From the electrodes in our smartphones to lubricants in machinery, graphite plays a pivotal role across various sectors. Understanding the properties of graphite is essential not only for scientists and researchers but also for those in technology and manufacturing industries.
Many people might love to use graphite pencils without knowing what lies behind that dark mark on paper. Unpacking the unique characteristics of graphite helps us appreciate its multifaceted uses. It’s time we take a closer look at what makes this material so special and indispensable in modern applications.
Research Overview
Summary of Key Findings
The examination of graphite has led to several key insights:
- Molecular Structure: Graphite has a layered structure made up of carbon atoms arranged in a hexagonal lattice. This specific arrangement contributes to its unique properties such as electrical conductivity and thermal resistance.
- Electrical Conductivity: Unlike most non-metals, graphite conducts electricity, making it valuable in various electrical applications, including batteries and electrodes.
- Thermal Conductivity: Graphite is also an excellent conductor of heat, which is why it is often employed in high-temperature applications.
- Mechanical Strength: Despite being relatively light, graphite possesses a good degree of strength, especially when utilized in composites and other structural applications.
- Reactivity: The chemical properties of graphite allow it to form compounds with various elements, which is significant in both industrial applications and research.
Research Objectives and Hypotheses
The primary aim of this exploration is to systematically break down graphite's properties and their applications. The specific objectives are:
- To analyze the molecular structure of graphite and how it affects its properties.
- To evaluate its conductivity and how it benefits multiple industries.
- To assess its mechanical properties and potential uses in advanced materials.
- To explore its reactivity and implications for chemical applications.
Hypothesis: It is postulated that the unique structural arrangement of carbon in graphite gives rise to its distinctive physical and chemical characteristics, leading to its varied applications across sectors.
Methodology
The analysis of graphite's properties was conducted through a combination of literature review, laboratory testing, and case study evaluations. This multi-faceted approach allowed for a comprehensive understanding of its characteristics and utilizations.
Study Design and Approach
- A qualitative study was employed to assess existing literature, focusing primarily on peer-reviewed articles, industry reports, and relevant case studies.
- A quantitative aspect was included through experimental testing, examining specific properties such as conductivity in controlled conditions.
Data Collection Techniques
Various techniques were utilized:
- Literature Review: Extracting data from databases like PubMed and Google Scholar for in-depth information.
- Laboratory Experiments: Conducting tests using equipment like conductivity meters and heat flow analyzers to measure specific properties accurately.
"Graphite serves as a linchpin in technological innovation, enabling advancements that may one day redefine how we think about materials."
This structured methodology ensures a robust analysis, paving the way for a deeper comprehension of graphite and its pivotal role in modern applications.
Prelude to Graphite
Graphite, a material often overlooked in daily conversations, holds a prominent place in the realm of materials science and technology. It is prevalent in numerous applications spanning from industrial use to cutting-edge technological implementations. Understanding graphite is akin to grasping the underpinnings of modern functionality in various devices and systems.
This segment sets the stage for a deep dive into the properties of graphite, emphasizing its significance as an all-around performer in diverse fields. The discussion will illuminate the structural components, unique characteristics, and outstanding applications of graphite, establishing a foundation for comprehending its role in technological advancements.
Enhancing one’s knowledge of graphite starts with recognizing its ubiquity. Whether in the pencils we use to jot down thoughts or in advanced batteries that power our electronics, graphite plays a pivotal role. It possesses qualities like conductivity and flexibility, making it indispensable in many sectors, including electronics, energy, and manufacturing.
In this article, we will not only explore these qualities but also the molecular structure and physical characteristics that contribute to its remarkable functionalities. Each layer of understanding builds upon the last, allowing readers to appreciate not just what graphite is, but how its properties correlate directly to its applications.
- Key Factors in Focus
- Molecular structure and its implications on properties.
- Physical traits that enable versatility across applications.
- The balance of chemical stability and reactivity, which tailors graphite for specific uses.
As we embark on this examination of graphite, keep in mind that this material is more than just a simple carbon allotrope. Its potential is vast, reflecting a complexity that merits a thorough exploration.
"Graphite’s true strength lies in its layered structure, a feature critical to its functionality."
The path ahead will guide you through an insightful analysis of graphite’s myriad properties, weaving an informative narrative that resonates across academic and industrial arenas alike.
Molecular Structure of Graphite
Understanding the molecular structure of graphite is crucial for appreciating its unique properties and applications. At its core, graphite consists of layers of carbon atoms arranged in a hexagonal lattice. This arrangement allows for a remarkable combination of strength and flexibility, making graphite a sought-after material in various industries. The significance lies not only in its physical characteristics but also in the implications those characteristics have for its usage in technology and material science.
Graphene Layers
Graphite can be visualized as a collection of graphene layers, each one atom thick. These layers are bonded together by weak van der Waals forces, which allows them to slide over one another with ease. This structure is responsible for graphite's well-known lubricating properties. When you think about it, this ability to slide reduces friction, making it ideal for use in applications such as lubricants and even in pencil lead.
The thickness of these graphene sheets is what dictates many of graphite's extraordinary characteristics. From electrical conductivity to heat resistance, the unique stacking of these layers plays a driving role. The ability to isolate a single layer of graphene has opened new avenues for research and application, highlighting its potential in nanotechnology and electronics.
- Key Characteristics of Graphene Layers:
- Made of carbon atoms arranged in a two-dimensional honeycomb lattice.
- Characterized by high thermal and electrical conductivity.
- Provides unique mechanical properties, including flexibility and strength.
Bonding and Hybridization
Examining the bonding within graphite uncovers its fascinating properties. Each carbon atom in graphite uses sp² hybridization, leading to a trigonal planar arrangement. This process involves the mixing of one s orbital and two p orbitals to form three equivalent orbitals. This hybridization accounts for the strength of the covalent bonds between carbon atoms, resulting in a sturdy yet versatile structure.
These sp² hybridized carbon atoms create sigma bonds in the plane of the graphene layers, while the remaining p orbitals create delocalized pi bonds above and below the plane. This delocalization is what facilitates graphite's impressive electrical conductivity, as electrons are not tied down to specific atoms, enabling them to flow freely.
- Important Aspects of Bonding and Hybridization:
- Covalent bonds formed between carbon atoms provide stability.
- Delocalized electrons allow efficient charge transport, crucial for electronic applications.
- This structure accounts for the unique anisotropic properties of graphite, meaning its behaviors are direction-dependent.
"Graphite's layered structure is a key player in its diverse functionality, from serving as a lubricant to enabling high-capacity storage in batteries."
Through this exploration of molecular structure, we lay a foundation for understanding how these fundamental characteristics underpin the many applications of graphite. From electronics to engineering, recognizing the importance of graphene layers and bonding mechanisms constantly drives innovation and research in this remarkable material.
Physical Properties of Graphite
When we talk about graphite, its physical properties often take center stage. These characteristics serve as the foundation of why graphite is so useful across various applications and industries. Understanding these properties helps us appreciate the material not just as a source for pencils but as a vital player in energy storage, lubrication, and much more.
Appearance and Color
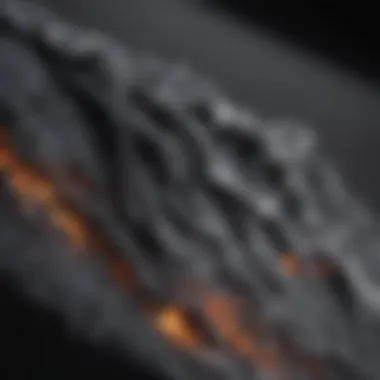
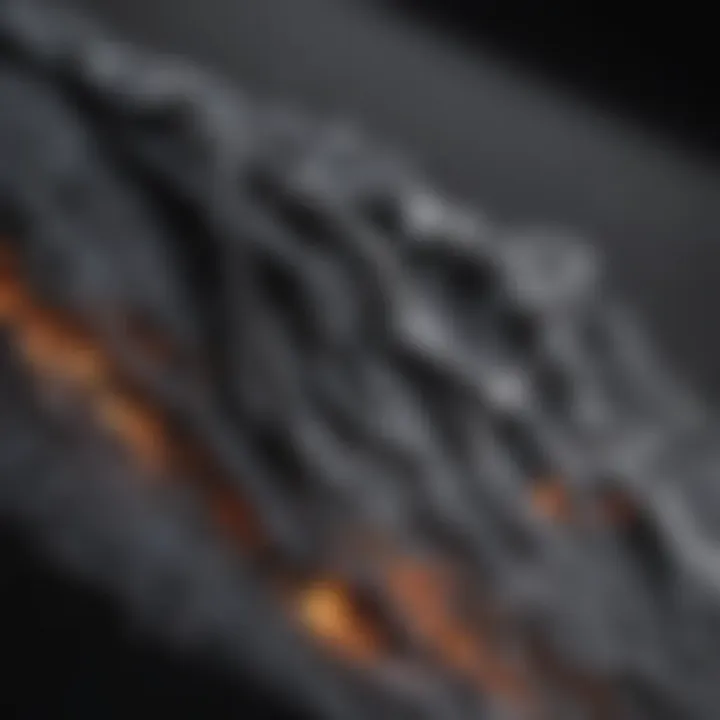
Graphite is usually seen as a gray-black material, which can make it appear rather unremarkable at first glance. However, nuances exist within its visual presentation. When examined closely, graphite can show a lustrous sheen, especially when in layered forms. This shine is what draws people in; it’s almost like a subtle invitation to learn more about its attributes. The color can sometimes vary based on its purity and source. For example, higher purity graphite can tend toward a more metallic luster, whereas lower purity options may look dull and ashy.
Additionally, the texture can range from smooth to somewhat gritty, depending upon its form—whether it's powder, flakes, or larger chunks. These visual cues are more than just skin-deep; they signal the quality and potential applications of graphite in industries such as electronics and aerospace.
Density and Hardness
Graphite possesses a relatively low density compared to metals like iron but has remarkable hardness that is noteworthy. The specific density of graphite can vary from about 1.5 to 2.3 g/cm³ based on the type and processing it has undergone. This makes it lightweight yet sturdy, a unique combination that is appealing in multiple industrial contexts.
The hardness of graphite can be misleading at first. It’s softer than diamond but harder than many other materials. This characteristic allows it to function effectively as a lubricant; it can endure high pressures without packing down. It can withstand significant wear and tear, so that's why it's often found in applications like brake linings and sealants, where durability is key.
Thermal Properties
Thermal Conductivity
One of the standout features of graphite is its high thermal conductivity. This means that it can effectively dissipate heat, making it ideal for applications that require the management of thermal energy. Graphite’s thermal conductivity is significantly greater than that of metals such as steel. This characteristic comes from the layer structure of graphene that provides high mobility for heat transfer. Because of this, it's a popular choice in heat sinks and thermal management systems in electronics and automotive industries.
Graphite's capacity to manage heat plays a critical role in its selection for demanding technological applications, ranging from aerospace to battery systems.
Thermal Expansion
Thermal expansion might not sound like the most exciting topic, but it’s crucial when discussing materials. Graphite exhibits anisotropic thermal expansion, meaning it expands differently based on the direction of heat application. In layman’s terms, this can be quite beneficial. For instance, in applications where precise tolerances need to be maintained despite temperature changes, graphite’s predictable expansion can prevent unwanted deformities.
So, while other materials might warp or produce stress under heat, graphite tends to keep its cool—literally and metaphorically. However, careful consideration must be taken in environments with extreme temperature fluctuations. The anisotropic nature could lead to complications if not accounted for in the design phase.
Ultimately, the thermal properties of graphite contribute substantially to its reputation as an invaluable material in modern industries, whether in electronics, engineering, or even renewable energy solutions.
Chemical Properties of Graphite
Understanding the chemical properties of graphite is essential for grasping its versatile roles across different sectors. These properties dictate how graphite behaves when interacting with various substances, thus shaping its applications in different fields, from batteries to lubricants.
Reactivity with Acids and Bases
Graphite showcases a unique behavior when exposed to both acids and bases. Unlike many materials, it is relatively inert in strongly acidic or basic environments. Typically, graphite does not react noticeably with hydrochloric or sulfuric acid, which makes it a suitable material for electrochemical cells. However, some more aggressive reagents, such as nitric acid, can cause oxidation, which modifies its structure and electrical properties.
Moreover, when graphite interacts with bases like sodium hydroxide, noteworthy transformations occur. The alkali can intercalate between graphene layers, causing an expansion of the structure. This characteristic becomes invaluable in applications such as catalysts and specialized electrodes.
"Graphite's resistance to chemical attack is a compelling reason for its use in a multitude of applications. Its stability serves as a foundational trait in diverse environments."
Oxidation and Reduction Reactions
Graphite's reactions involving oxidation and reduction are complex yet significant. The process of oxidation in graphite usually involves the incorporation of oxygen into its structure, which can lead to the creation of graphite oxides. These oxides hold particular importance in material science due to their enhanced reactivity compared to pure graphite.
Conversely, reduction reactions are pivotal in the regeneration of reduced graphite oxide back to its original form. This balance of oxidation and reduction is fundamental in numerous applications, especially in the realm of energy storage.
Here’s a concise overview of what oxidation and reduction mean for graphite:
- Oxidation:
- Reduction:
- Involves reaction with oxygen or other oxidizing substances.
- Alters structural properties and can lead to functionality fluctuations.
- Restores graphite oxide back to graphite.
- Essential in battery designs, improving charge retention.
Overall, the chemical properties of graphite play a pivotal role in its vast array of uses, affecting everything from durability to functionality in electronic applications. The interplay between these reactions not only highlights the material's resilience but also its adaptability, accommodating the demands of modern-day technology.
Electrical Conductivity of Graphite
Electrical conductivity is a cornerstone attribute of graphite, shaping its utility across numerous modern applications. Understanding how graphite behaves electrically often illuminates its significance in various fields ranging from electronics to renewable energy. The conductivity of graphite stems primarily from its unique molecular arrangement; the presence of delocalized electrons allows for efficient charge transport. To tease out more about this phenomenon, we must dive into the fundamentals of charge carrier mobility and its implications.
Mechanism of Charge Carrier Mobility
The mobility of charge carriers in graphite can be attributed to its structure. Graphite consists of layers of graphene, where carbon atoms form a hexagonal lattice. Within these layers, each carbon atom bonds with three others, leaving one electron essentially free, contributing to electrical conduction. These delocalized electrons can shift relatively freely within the plane of each graphene layer, facilitating conductivity.
The movement of charge carriers is not merely random; it is intricately linked to temperature and structural integrity. For instance, an increase in temperature can augment vibrational energy, potentially scattering these electrons and reducing their mobility. But, under optimal conditions, the conductivity in graphite can rival that of metals. This distinct mechanism gives graphite its edge in sensitive electronic applications.
Applications in Electronics
The impressive electrical properties of graphite open avenues for its application in a plethora of electronic devices. Here’s a closer look at its varied uses:
- Battery Technologies: Graphite serves as a vital component in lithium-ion batteries, providing a stable anode material that enhances energy density and cycle life.
- Conductive Coatings: Its excellent conductive features allow for its use in conductive paints and coatings, making surfaces electrically conductive without compromising on aesthetics.
- Electrodes: Graphite is widely used in electrodes for electrochemical processes, including fuel cells and supercapacitors.
- Transistors: In the realm of semiconductor technology, graphene derived from graphite is utilized to create faster, smaller transistors, reshaping the future of computing.
"Graphite not only demonstrates substantial electrical conductivity but also embodies versatility that continues to drive innovations across various sectors."
Thermal Conductivity of Graphite
Understanding the thermal conductivity of graphite is crucial for various applications, particularly where heat management is of utmost importance. Graphite boasts exceptional thermal conductivity, which means it can efficiently transfer heat. This property is largely due to its unique molecular structure – layers of graphene that allow for rapid energy dispersion. Such efficiency in thermal transfer can have direct implications across industries ranging from electronics to aerospace, where overheating can lead to component failure.
Comparison with Other Materials
Graphite's thermal conductivity is often compared against materials like metals and ceramics. To illustrate this comparison:
- Graphite vs. Copper: While copper is renowned for its thermal conductivity, graphite holds its own, especially in certain temperatures and conditions. Graphite's performance can surpass copper in specific applications where its lightweight nature provides an added benefit.
- Graphite vs. Aluminum: Aluminum is frequently used for heat dissipation, but graphite's ability to handle higher temperatures without losing structural integrity makes it a solid choice for demanding environments.
- Graphite vs. Ceramics: Typically, ceramics do not perform as well as graphite in thermal conductivity. However, they have their strengths in certain high-temperature applications. The choice between these materials often becomes a matter of balancing conductivity with physical properties needed for the application at hand.
Applications in Heat Management
The applications of graphite in heat management are as diverse as they are crucial. Some specific uses include:
- Heat Sinks: Graphite is used in heat sinks for electronic devices. Its ability to quickly disperse heat away from sensitive components makes it invaluable in preventing overheating and extending device lifespan.
- Thermal Interface Materials: In the realm of electronics, thermal interface materials utilize graphite to enhance cooling efficiency by facilitating better thermal contact between components.
- Aerospace and Automotive: In high-performance environments, graphite is found in heat shields and thermal blankets. Its lightweight and high conductivity ensure that energy is managed effectively, thus contributing to overall safety and performance.
- Industrial Processes: Many manufacturing processes, such as metal refining, require careful heat management. Graphite plays a vital role in maintaining appropriate temperatures for material manipulation, ensuring quality and safety in production.
"The ever-increasing demand for thermal management solutions highlights graphite's rise as a go-to material in cutting-edge technology."
Mechanical Properties of Graphite
The mechanical properties of graphite are fundamental to understanding its versatility across various industries. Graphite isn't just a pencil lead; it’s a material with a unique set of characteristics that make it valuable in applications requiring both strength and flexibility. By examining its compressive and tensile strength, along with its elasticity and ductility, we can appreciate how graphite stands out in the material world.
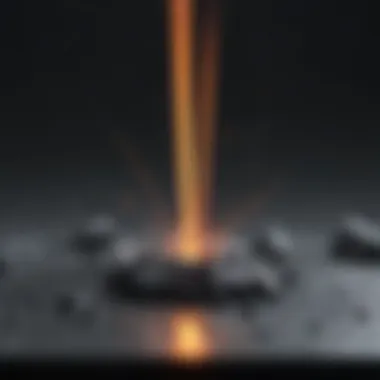
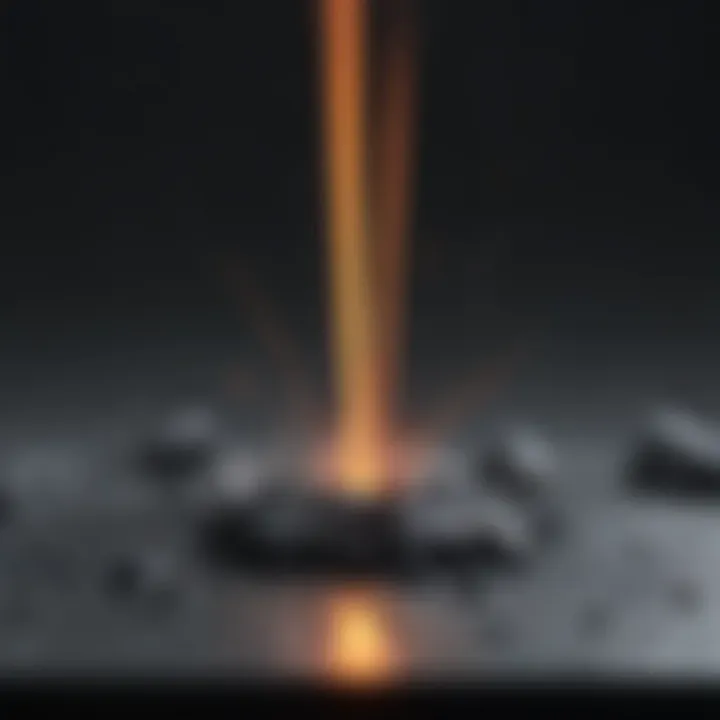
Compressive and Tensile Strength
When it comes to load-bearing applications, compressive and tensile strength are paramount. Compressive strength measures how much load a material can bear while being compressed, whereas tensile strength pertains to the resistance against being pulled apart.
For graphite, both of these properties are impressive but with a unique twist. While it exhibits good compressive strength, it's the tensile strength that is particularly notable. Graphite can withstand significant pulling forces without breaking, thanks to its layered structure, which allows the planes to slip past each other without major damage.
This quality makes graphite ideal for components such as seals, bushings, and gaskets. The high tensile strength helps keep these components intact, even under high-stress environments. In applications like aerospace and automotive, where every gram matters and performance is non-negotiable, graphite provides a lightweight yet strong option.
Moreover, its ability to maintain structural integrity at elevated temperatures further enhances its desirability in high-performance applications. For professionals in engineering or material science fields, understanding the precise compressive and tensile strengths in relation to their project requirements can significantly influence material selection.
Elasticity and Ductility
Elasticity refers to a material's capacity to return to its original shape after deformation, while ductility describes its ability to stretch without breaking. Graphite showcases interesting behavior in both aspects.
Graphite is generally considered brittle, yet its layered crystalline structure imparts a certain degree of elasticity. This means that while it may not 'bounce back' like rubber, it can endure some level of stress without permanent deformation. This property is crucial during manufacturing processes where alterations in shape are expected, such as during machining or molding.
Ductility is another defining characteristic. Although graphite doesn’t exhibit ductility in the same manner as metals, it can still be processed into fibers and sheets. This readies it for use in composite materials that can yield increased strength while maintaining lightweight advantages.
For industries looking to innovate, this mix of elastic behavior coupled with a controllable degree of ductility encourages experiments with synthetic and natural graphite composites. The flexibility of graphite extends to its ability to be used in a range of products, from advanced batteries to architectural materials, where unique mechanical properties are desired.
"The mechanical properties of graphite set it apart as a shining star within the realm of engineering materials, merging strength, flexibility, and performance seamlessly."
As the discussion unfolds, it becomes clear that when selecting materials for various applications, the mechanical properties of graphite ought to be at the forefront of consideration given their broad implications in terms of performance and efficiency.
Thermal Expansion Behavior
The study of thermal expansion behavior in graphite plays a pivotal role in understanding its physical characteristics and performance in various applications. Thermal expansion refers to the tendency of matter to change in shape, area, and volume in response to a change in temperature. For graphite, this behavior is particularly interesting due to its unique structure and properties. Understanding how graphite expands or contracts when subjected to temperature changes is critical for industries that utilize this material, ensuring the integrity and reliability of products.
One of the core aspects of thermal expansion behavior in graphite is that it exhibits anisotropic expansion. This means that the material expands at different rates along different axes. Such characteristics stem from graphite's layered structure, where graphene layers can slide over one another with relative ease. These layers, when heated, respond differently compared to their orientations when subjected to different thermal conditions. This property can lead to enhanced performance in applications that require materials to maintain structural integrity under varying thermal loads.
In addition to the anisotropic nature of graphite expansion, understanding its temperature dependence is equally critical. The rate at which graphite expands is influenced by temperature ranges. For instance, at lower temperatures, the expansion might be minimal, while exposure to elevated temperatures can result in considerable changes in dimensional stability. This behavior is significant for designers and engineers who work with graphite-based technologies, as it helps predict how a graphite component will perform under operational heat stresses.
Anisotropic Expansion
Graphite's anisotropic expansion can be vividly illustrated when comparing its behavior along the 'c-axis' and the 'a-axis.' When heating graphite, the dimensional change is greater in the perpendicular direction, showcasing how different axes respond uniquely to temperature changes. This knowledge is particularly advantageous in the manufacturing of components in heat-sensitive environments, such as engines or electronic devices. It helps inform design decisions—one must consider how the orientation of the graphite within a component could impact its overall performance.
Here are some key points regarding anisotropic expansion in graphite:
- Directionality: Expands more significantly in the plane of the graphene layers than perpendicular to them.
- Implications for Engineering: When incorporating graphite in multi-material assemblies, understanding the expansion differences can help mitigate internal stresses that may lead to failure.
Temperature Dependence
The temperature dependence of graphite's thermal expansion can be quantified using experimental data. Generally, as temperatures increase, graphite experiences a notable expansion. However, this does not happen at a uniform rate throughout its entire temperature range. Most importantly, this expansion behaves linearly at moderate temperatures, yet deviates at extreme temperatures, particularly when approaching or exceeding its thermal stability limits.
The situation becomes more complex at elevated temperatures:
- At around 1000 °C, significant degradation may occur, altering its properties and performance.
- The transition temperature at which the properties of graphite may change underscores the need for careful thermal management in applications that operate in extreme environments.
In sum, both anisotropic expansion and temperature dependence are essential to understanding the thermal expansion behavior of graphite. They provide valuable insights into how graphite can be effectively utilized in a variety of technological applications, contributing to safer and more efficient designs in advanced industries.
"The anisotropic nature of graphite's thermal expansion not only shapes its applications but also challenges engineers to think creatively about material use in diverse environments."
Applications of Graphite
Graphite's essential nature lends itself to numerous applications across various industries. The unique properties of this carbon allotrope make it an indispensable material in fields ranging from manufacturing to energy production. Understanding how graphite is utilized helps us appreciate its role in modern technology and innovation. This section highlights the significance of graphite's applications, focusing on its benefits and considerations in different fields.
In Industrial Applications
Graphite holds a prominent place in industrial applications due to its impressive properties such as high thermal conductivity, electrical conductivity, and chemical stability. Industries that require materials that can withstand high temperatures and pressures often turn to graphite. For example, in the steel industry, graphite serves as a vital component in the production of steel due to its ability to enhance the durability and overall quality of the metal.
Moreover, graphite is commonly used in lubricants, creating a friction-resistant barrier that supports mechanical efficiency. The automotive industry, in particular, relies on graphite for brake linings and gaskets, owing to its ability to resist heat and wear. The following are a few key industrial applications of graphite:
- Electrode manufacturing: Graphite is used to produce electrodes for electric arc furnaces.
- Mold releases: In the casting industry, graphite is applied as a release agent to allow easy removal of castings.
- Battery production: The lithium-ion battery sector heavily relies on graphite as an anode material, owing to its high intercalation capacity.
These examples underscore the multifaceted nature of graphite in industrial applications, where its unique properties make it a material of choice.
Use in Renewable Energy Technologies
The push for a more sustainable future has led to increased interest in renewable energy sources. Graphite plays a pivotal role in this transition, primarily as a key material in battery technology and energy storage systems. The surge in electric vehicles and renewable energy systems like solar and wind power highlights the need for effective energy storage solutions.
Graphite's suitability for batteries stems from its excellent conductivity and ability to accommodate lithium ions, which enables faster charging and longer battery life. Consequently, its application in advanced energy storage devices not only enhances performance but also promotes a greener planet. Here are some notable ways graphite contributes to renewable energy technologies:
- Solar panel production: Graphite is used in the production of electrodes for solar energy systems.
- Supercapacitors: The lightweight properties of graphite allow for the development of highly efficient supercapacitors with rapid charge-discharge capabilities.
- Fuel cells: Graphite is integral in fuel cell technology, providing a platform for reactions with minimal mass transport resistance.
Through these applications, graphite is establishing itself as a major component in fostering innovations for a sustainable energy landscape.
Role in Lubricants and Coatings
Graphite's application as a lubricant and in coatings speaks volumes about its versatility and unique properties. Traditional lubricants often break down under high pressure or heat, leading to increased friction and wear. Graphite, on the other hand, withstands extreme conditions without losing effectiveness, making it an ideal alternative.
For heavy machinery, graphite lubricants help to extend the lifespan of components and enhance performance, making them invaluable in industries such as construction and mining. The use of graphite in coatings also deserves a mention. Its ability to form a thin protective layer aids in preventing corrosion, which is crucial in environments that expose machinery to harsh conditions. Here are a few key points about graphite in lubricants and coatings:
- Dry lubricant: Graphite serves as a dry lubricant in applications where liquid lubricants might be unsuitable.
- Anti-corrosive properties: Coatings containing graphite provide resistance against corrosion, increasing the durability of surfaces.
- Heat resistance: Graphite's thermal properties lend themselves effectively to high-temperature applications.
Graphite in Advanced Technologies
Graphite plays a pivotal role in contemporary technology, which is often overlooked. Its unique structure and properties make it an indispensable material in various applications that stretch across many fields, from consumer electronics to energy storage solutions. This section will delve into two of its most significant applications: batteries and supercapacitors, as well as its role in nanotechnology.
Use in Batteries and Supercapacitors
Batteries and supercapacitors have become essential devices in today's world, especially with the rising demand for portable electronics and electric vehicles. Graphite is primarily used in the anodes of lithium-ion batteries, acting as a crucial component that stores lithium ions when the battery charges and releases them during discharge.
Its layered structure allows for efficient lithium ion diffusion, leading to improved energy density and charging rates. Not only does graphite contribute to the overall performance, but it also affects the battery’s lifespan.
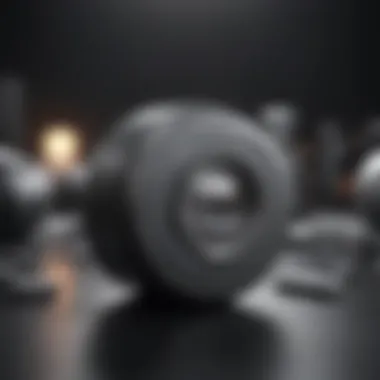
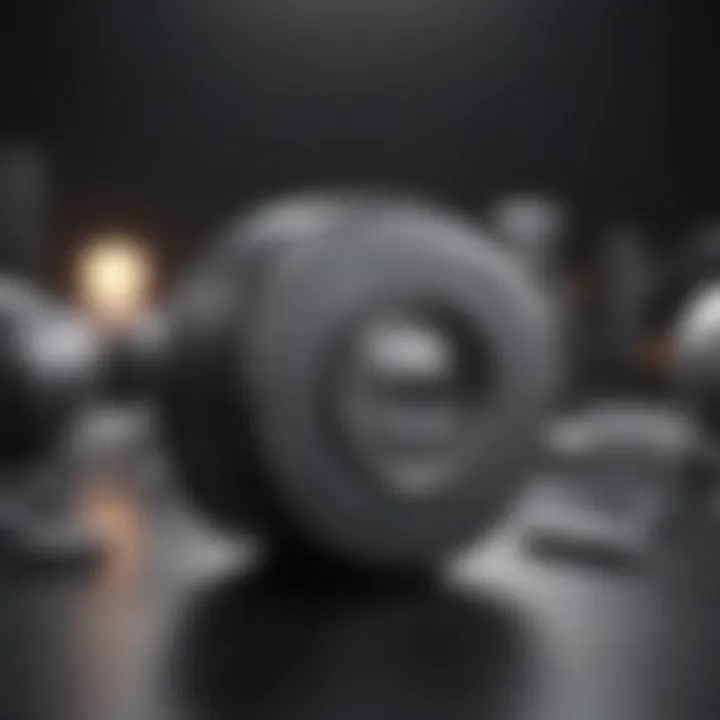
Some key advantages of using graphite in batteries include:
- High Electrochemical Stability: Graphite maintains its structure better than many alternatives, making it a reliable choice for consistent performance.
- Abundant and Cost-Effective: Given that graphite is readily available, it presents a cost-effective solution compared to other materials.
- Environmentally Friendly: Compared to some synthetic alternatives, natural graphite has a lower environmental impact in terms of sourcing and processing.
However, it’s not without challenges. As technology advances, the quest for higher energy densities implies a need for new materials, which could potentially replace or complement graphite in future battery technologies.
Application in Nanotechnology
Beyond its role in batteries, graphite, in the form of graphene, has carved a niche in the realm of nanotechnology. Graphene, a one-atom-thick layer of carbon atoms arranged in a hexagonal lattice, inherits the remarkable properties of graphite while enhancing them at the nanoscale.
- Exceptional Strength: Graphene is remarkably strong, offering a tensile strength much higher than steel, while remaining flexible and lightweight.
- High Electrical Conductivity: This property of graphene enables faster electron mobility, vital for applications in high-speed electronics.
- Versatility: The potential uses of graphene are staggering. It can be used in sensors, conductive coatings, and even new materials for aerospace technology.
"Graphene’s introduction altered the landscape of material science, providing avenues for innovations previously thought impossible."
Research continues to explore the integration of graphene into next-gen technologies, with a particular focus on how these materials could interact with existing systems in novel ways. This includes enhancing the efficiency of energy generation, storage devices, and even improving wearable technologies.
Environmental Considerations
Graphite, while a remarkable material for its myriad applications, poses significant environmental considerations that merit careful examination. As industries burgeon, it's imperative to look at how graphite sourcing, production, and disposal affect the ecosystem. This section will delve into sustainability and sourcing strategies, alongside examining the role recycling plays in mitigating negative environmental impacts.
Sustainability and Sourcing
Sustainability in graphite sourcing revolves around responsible practices that minimize environmental degradation. Extracting graphite, whether from natural mines or synthetic processes, can have far-reaching impacts. Natural graphite mining often leads to deforestation, soil erosion, and water pollution. Companies involved must adhere to rigorous environmental standards that not only aim to reduce these impacts but also promote the regeneration of local ecosystems.
- Key aspects of sustainable sourcing include:
- Ensuring land reclamation after mining operations, which encourages the recovery of native flora and fauna.
- Employing water-efficient techniques to limit usage and pollution in the surrounding areas.
- Supporting local communities through fair trade practices, ensuring the socio-economic benefits of mining are equitably shared.
Moreover, the emerging trend towards synthetic graphite production opens up avenues for sustainability. This approach typically uses less water and can incorporate recycled materials, thus reducing carbon footprints.
Recycling and Reuse
The importance of recycling and reuse in graphite cannot be overstated. As demand for graphite surges, especially in tech industries such as batteries, the potential to minimize raw material extraction through recycling becomes paramount. Rather than relying solely on virgin materials, repurposing graphite can lead to resource conservation and significant cost savings.
Recycling graphite involves retrieving this material from used products like batteries and lubricants. Through advanced processing technologies, these graphite materials can be reclaimed and reintegrated into new products. This not only reduces the strain on natural resources but also lowers environmental waste.
"As a result, effective recycling strategies for graphite help close the material loop, making it a cornerstone of sustainable practices in modern industries."
For instance:
- Applications of recycled graphite include:
- Battery production: Recycled graphite can be used in new lithium-ion batteries, thus powering electric vehicles and renewable energy storage.
- Lubricants and coatings: Utilizing recycled graphite in industrial applications decreases reliance on fresh resources, showcasing the practicality of reusing materials.
In summary, the path toward a sustainable future in graphite utilization hinges significantly on thoughtful sourcing practices and effective recycling methods. By addressing these elements, stakeholders can considerably lessen the environmental footprint associated with this versatile material.
Future Trends in Graphite Research
The exploration of future trends in graphite research is not just an academic exercise; it represents a critical crossroads for technology and material sciences. As industries evolve, the demand for advanced materials like graphite grows, propelling innovations that could markedly affect various sectors from electronics to renewable energy. This section will discuss these emerging trends, underscoring their importance for researchers, manufacturers, and environmentalists.
Emerging Applications in Technology
The versatility of graphite is poised to lead to its use in emerging technologies that capitalize on its unique properties. Several key areas stand out:
- Energy Storage Solutions
- Wearable Technology
- 3D Printing
- Graphite is seeing increased integration in battery technology, particularly in lithium-ion batteries, where it serves as an essential anode material. Recent research indicates that enhanced graphene-based contributions can yield batteries with faster charging times and improved longevity.
- With the rise of smart textiles and wearable devices, graphite's conductive properties may play a role in developing flexible electronics. Potential applications include sensors that monitor biometric data or fabrics that can change in response to environmental stimuli.
- In additive manufacturing, graphite's conductivity makes it an attractive option for printing components that require electrostatic discharge protection. As this technology evolves, the demand for graphene-infused materials is expected to surge, particularly in the realm of aerospace and automotive parts.
These applications not only showcase graphite's adaptability but also highlight its potential in shaping future technologies. With ongoing research, we can anticipate fine-tuning of these applications to achieve higher efficiency and functionality.
Innovations in Material Synthesis
The future of graphite research also lies significantly in innovations surrounding material synthesis. Advancements are critical as they can result in:
- Enhanced Graphene Production
- Composite Materials
- Sustainable Synthesis Techniques
- Traditional methods of graphene production are time-consuming and costly. New techniques are emerging, including chemical vapor deposition and liquid-phase exfoliation, that promise quicker production rates with superior material quality. Such innovations open doors for broader commercial applications of graphene.
- Researchers are focusing on developing composite materials that blend graphite with polymers or metals to achieve a synergy of properties. These composites can lead to lightweight, strong materials ideal for use in transportation and aerospace sectors, contributing to fuel efficiency and performance.
- With an eye toward environmental impacts, green chemistry approaches are being explored for graphite synthesis. Innovations may include utilization of renewable resources or less environmentally damaging processing methods, making the entire lifecycle of the material more sustainable.
As these innovative synthesis methods gain traction, they could also reduce production costs and enable wider adoption of graphite-based technologies across various industries.
"The future research landscape of graphite is not purely academic; it is a significant player in our technological advancement and sustainability goals."
In summary, the exploration of future trends in graphite research sets the stage for breakthroughs in diverse applications and enhanced material synthesis methods. By embracing these trends, industries can harness graphite's potential, paving the way for innovations that are not only smart but also sustainable.
Finale: The Significance of Graphite
In this examination of graphite, it becomes increasingly clear that this material is not just a simple carbon allotrope. Its multifaceted properties make it a cornerstone in various fields, from basic science to advanced technology. The significance of graphite cannot be overstated; it underpins many modern innovations and has far-reaching implications across a myriad of industries.
Key Takeaways from the Examination
Understanding the properties of graphite shines a light on several core aspects:
- Unique Molecular Structure: The layered arrangement of graphene sheets provides graphite with exceptional electrical and thermal conductivity. This is vital in electronics, where materials with high conductivity are essential for efficiency.
- Versatile Applications: Graphite’s function spans countless applications, including its role in batteries and renewable energy technologies. By harnessing its qualities, industries can develop more efficient energy storage and transfer solutions.
- Environmental Relevance: Attention to sustainability and responsible sourcing of graphite is crucial as demand continues to grow. Conscious efforts toward recycling and reuse can significantly mitigate environmental impacts resulting from extraction and production processes.
- Innovations on the Horizon: Ongoing research and emerging technologies suggest that the functionality of graphite will only expand. New synthesis methods hold promise for enhanced properties, which could lead to breakthroughs in nanotechnology and material sciences.
Considerations About the Importance of Graphite
Graphite is a material that connects several domains of science and technology, solidifying its relevance. Each section of our exploration has revealed something distinctive about graphite's contributions:
- In Industrial Settings: Its mechanical properties ensure durability and dependability, making it the go-to material in many industrial applications.
- In Energy Solutions: As the world shifts toward more sustainable practices, the role of graphite—before overlooked—is coming back to the forefront, mainly due to its use in clean energy technologies.
- In Research and Development: Current trends point to innovations in graphite synthesis, which are poised to unlock new capabilities in various fields of study.
"The story of graphite is still being written; its pages continue to turn as research unveils new potentials far beyond conventional applications."
This insight into the significance of graphite emphasizes that it is more than a mere material used in pencils or batteries. Its implications stretch across everyday life and into cutting-edge research. By understanding its properties and potential, we equip ourselves to contribute more meaningfully to advancements in science, industry, and sustainability. As we move forward, a nuanced appreciation of graphite will be critical in leveraging its advantages to meet the challenges of tomorrow.