Mechanisms and Implications of Protease Digestion
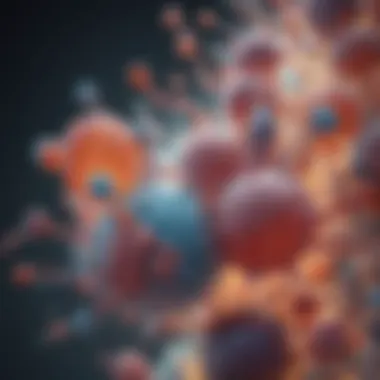
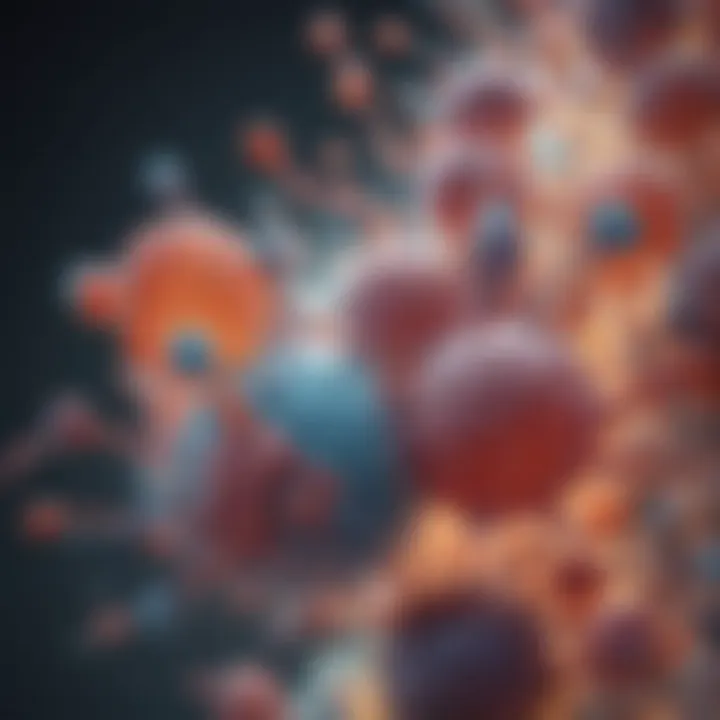
Intro
Proteins are the workhorses of the cellular machinery, playing a pivotal role in various biological processes. A key player in the lifecycle of proteins is proteases, enzymes responsible for the breakdown of proteins into peptides and amino acids. Understanding protease digestion is crucial, not just for biology students, but also for researchers, clinicians, and professionals who recognize the implications of these biochemical reactions in health and disease.
Protease digestion isn't just a simple process of chopping proteins into smaller pieces. It's an intricate dance of enzyme-substrate interactions influenced by many factors. Different types of proteases operate under varying conditions, with some thriving in acidic environments, like pepsin in the stomach, while others function best in neutral pH, like trypsin in the small intestine. This specificity is fundamental to the digestion of dietary proteins as well as the regulatory mechanisms in cellular metabolism.
In a world where advances in biochemistry and molecular biology are accelerating, the implications of protease activity span beyond mere digestion. Recent research is shedding light on protease inhibitors, which are being examined for their potential in therapeutic contexts, particularly in diseases where protease malfunction can lead to severe consequences.
As we delve deeper into protease digestion, it becomes clear that this topic has multiple layers. From fundamental mechanisms to the latest research trends, this comprehensive examination aims to educate and inform all who are curious about the biochemical processes that help sustain life.
Understanding Proteases
Proteases are crucial enzymes that catalyze the breakdown of proteins into peptides or amino acids. Understanding them is fundamental in both biological research and therapeutic applications. When we delve into the mechanisms of proteolysis, we unveil the intricate processes that sustain life, encompassing digestion, metabolism, and even pathological conditions. With a detailed look into the characteristics and functions of different proteases, this article aims to provide a roadmap for grasping the biochemical landscape of these enzymes.
Definition and Function
A protease, also known as a proteinase or peptidase, is an enzyme that performs proteolysis; that is, it cuts proteins into smaller polypeptides or single amino acids. These enzymes play a significant role in various biological processes. For instance, they are indispensable in digestion where they help break down dietary proteins into amino acids, which are then absorbed by the body for various functions, such as tissue repair and hormone production.
Moreover, proteases are involved in cellular regulation, cell signaling, and the immune response. They can be specific, targeting certain bonds in a protein, or broad-spectrum, digesting a wide range of substrates.
Classification of Proteases
Proteases can be classified based on various criteria such as site of action, catalytic mechanism, and specific amino acid residues involved in catalysis. This classification helps in understanding their function and application better. Below are five primary types of proteases:
Endopeptidases
Endopeptidases are proteases that cleave peptide bonds within the protein chain, as opposed to cutting off amino acids from the ends. This characteristic makes them essential for preliminary digestion. One key aspect of endopeptidases is their ability to generate peptide fragments, which can further be acted upon by other enzymes, such as exopeptidases. Their capability to process proteins efficiently positions them as a beneficial choice in protease digestion, making them crucial in various biological contexts.
Unique to endopeptidases is their active site arrangement, typically involving a catalytic triad that enhances hydrolytic activity. However, their activity can sometimes lead to excessive protein degradation if not properly regulated, making them a double-edged sword in biological systems.
Exopeptidases
Exopeptidases, in contrast, act on the terminal bonds of polypeptides, releasing free amino acids. They are critical in the finishing stages of protein digestion. Their defining characteristic is specificity towards either amino acids or carboxyl terminals. This makes them particularly valuable in refining the products of protein catabolism. By trimming down peptide fragments to single amino acids, they ensure that the body can absorb these essential nutrients effectively.
The unique feature of exopeptidases is their ability to act quickly on short peptides, which can be advantageous for rapid amino acid absorption. Nonetheless, if overactive, they might inhibit effective digestion by limiting the variety of peptides available for bodily functions.
Cysteine Proteases
Cysteine proteases have a cysteine residue at their active site that plays a crucial role in catalysis. This class of protease is involved in various physiological processes including apoptosis and protein turnover. Their unique feature lies in their mechanism, which often involves the formation of a thiolate anion that attacks the carbonyl carbon of a peptide bond, leading to proteolysis.
Their importance in health can not be overstated as they participate in key processes. For example, they are involved in immune responses. However, these proteases can also contribute to pathologies such as cancer and neurodegenerative diseases when misregulated.
Serine Proteases
These proteases feature a serine residue at their active site and are known for their broad substrate specificity. This class includes some well-known enzymes like trypsin and chymotrypsin, which are pivotal during protein digestion in the small intestine. Serine proteases are characterized by a catalytic triad of serine, histidine, and aspartate, enabling a distinctive mechanism of action that promotes efficient protein cleavage.
Although they are hailed for their efficiency in digestion, their dysregulation can lead to diseases such as pancreatitis, highlighting the importance of balanced proteolytic activity in the body.
Aspartic Acid Proteases
Aspartic acid proteases, characterized by two aspartate residues at their active site, are known for their action in acidic environments. An excellent example can be found in pepsin, an enzyme that operates in the stomach to facilitate protein digestion amidst a low pH. The unique aspect of aspartic acid proteases is their activation in acidic conditions, making them indispensable for initial digestive processes in organisms that consume protein-rich diets.
Their role is crucial as they break down complex proteins into more manageable forms for further digestion. However, excessive activity can lead to digestive disorders or gastric inflammation, showcasing yet another aspect of the importance of regulation in protease activity.
Mechanism of Protease Digestion
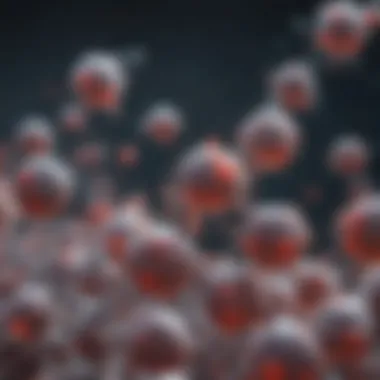
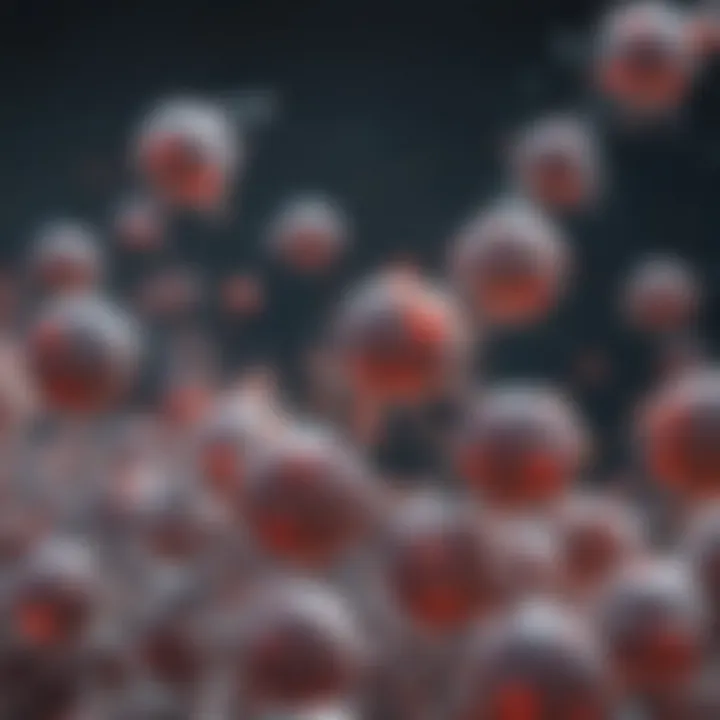
Understanding the mechanisms of protease digestion is fundamental to grasping how proteins are broken down in various biological systems. Each protease operates with precision, ensuring that proteins are meticulously processed to release amino acids vital for numerous cellular functions. This section unpacks different aspects of protease digestion, focusing on activation, substrate recognition, and the catalytic mechanisms involved. Recognizing these elements sheds light on their significance in digestion and potential implications for health and industry.
Activation of Proteases
The journey of protease digestion begins with the activation of proteases. Many proteases are synthesized in an inactive form known as zymogens. This precautionary measure prevents premature degradation of proteins within the tissue where they are produced. Activation occurs typically in response to specific stimuli. For instance, gastric proteases like pepsinogen convert to active pepsin in the acidic environment of the stomach. This transition not only catalyzes the breakdown of proteins but also signifies a crucial step in the digestive process, emphasizing how tightly regulated protease functions are within biological systems. The correct activation of these enzymes is essential; any misstep can lead to various digestive disorders or impaired nutritional absorption.
Substrate Recognition
Proteases do not act blindly; they exhibit a keen ability to recognize specific substrate proteins. This recognition is driven by the unique structure of the enzyme and the target substrate. The binding of a substrate involves intricate interactions between the amino acids on the protease and those on the target protein. This selectivity not only determines which proteins will be digested but also influences the overall efficiency of the digestion process. For example, the protease trypsin specifically targets the carboxyl side of lysine and arginine residues, showcasing the fit between enzyme and substrateβmuch like a key in a lock. This precise matching fortifies the importance of substrate recognition in ensuring that proteolysis occurs at the right sites and times.
Catalytic Mechanisms
The catalytic mechanisms employed by proteases further illustrate their complex functionality. Different classes of proteases utilize varying mechanisms which can be classified into three primary types: Dual Aspartate Mechanism, Cysteine Catalysis, and Serine Catalysis. Understanding how these mechanisms function not only enhances our grasp of protease activity but also points to their implications in various fields.
Dual Aspartate Mechanism
The Dual Aspartate Mechanism is notable for its efficiency in hydrolyzing peptide bonds. The unique characteristic of this mechanism lies in the utilization of two aspartate residues which operate in concert to facilitate the cleavage of peptide bonds. This mechanism is particularly beneficial due to its ability to stabilize the transition state, thus allowing the reaction to proceed more swiftly than those mechanisms reliant on a single acidic group. The advantages of this approach in protease digestion include improved speed and specificity, positioning it as a cornerstone in enzymatic function during protein breakdown.
Cysteine Catalysis
Next is Cysteine Catalysis, a mechanism recognized for its versatility across different biological contexts. The hallmark of this mechanism is the nucleophilic attack carried out by a cysteine residue. What's intriguing here is that it allows for a wide range of substrates to be processed, making it adaptable and vital in various physiological processes. While beneficial, one must consider the potential limitationsβmany cysteine proteases can be inhibited by oxidative modifications, which may impact their functionality in a biological setting.
Serine Catalysis
Finally, Serine Catalysis stands out for its contribution to processes ranging from digestion to signaling. The key characteristic of this mechanism involves a serine residue acting as a nucleophile, similar to that observed in cysteine catalysis, but here, serineβs hydroxyl group plays a pivotal role. This mechanism is a popular choice in protease catalysis owing to its robustness and efficiency. However, it also faces the challenge of regulation; serine proteases can be easily inhibited by protein inhibitors, which, while essential for control in various physiological pathways, can complicate therapeutic approaches where proteolysis needs to be precisely managed.
Understanding these catalytic mechanisms is crucial for developing therapeutic agents aimed at targeting specific proteases, which can be a double-edged sword in treating diseases involving dysregulated protein metabolism.
Physiological Role in Digestion
The physiological role of protease digestion is foundational to understanding how living organisms break down proteins into usable forms. By dissecting complex proteins into smaller peptides and amino acids, proteases facilitate nutrient absorption, crucial for energy production and cellular repair. This section delves into the distinct enzymatic actions that occur in different parts of the digestive system and highlights the significance of each step.
Protein Digestion in the Stomach
Gastric Proteases
Gastric proteases, the enzymes active in the acidic environment of the stomach, like pepsin, take center stage in protein digestion. The primary role of gastric proteases is to initiate the breakdown of proteins into smaller peptides. What sets them apart is their activation from precursor forms, known as zymogens, which prevents digestive enzymes from prematurely breaking down proteins in the stomach wall.
In their active form, gastric proteases exhibit remarkable stability under harsh acidic conditions, enabling efficient protein degradation.
The unique feature of gastric proteases is their ability to function optimally at a low pH, around 1.5-3.5. This harsh acidity not only enhances the enzymeβs activity but also denatures proteins, making them more accessible for enzymatic action. A key characteristic that makes these proteases beneficial is their efficiency in beginning the digestive process, paving the way for further breakdown in the small intestine. However, the disadvantage is that excessive activity might lead to gastric ulcers, indicating a delicate balance must be maintained in protease regulation.
Role of pH in Activation
The activation of proteases is heavily influenced by the pH level in various parts of the digestive tract. In the stomach, the acidic environment creates conditions conducive for the activation of gastric proteases. This aspect is pivotal because the enzymes need the proper pH to catalyze reactions effectively.
The crucial characteristic of pH in enzyme activation is its ability to confer specificity; that is, not every protease operates well in the same pH. For example, gastric proteases thrive in low pH, while pancreatic enzymes prefer neutral to slightly basic conditions when they enter the small intestine.
The unique feature of pH's role in enzyme activation is its influence on the structural configuration of proteases. A favorable pH alters the ionization states of amino acid residues at the active site, enhancing enzyme functionality. Yet, while being a beneficial parameter for activation, the challenge lies in the potential denaturing of enzymes if the pH strays too far from the optimal range.
Protein Digestion in the Small Intestine
Pancreatic Proteases
Once the partially digested proteins exit the stomach, pancreatic proteases take charge in the small intestine. Enzymes like trypsin and chymotrypsin, produced in the pancreas and activated in the intestine, extend the protein digestion process, converting peptides into even smaller units. These enzymes are secreted as inactive precursors, which are activated in the intestinal lumen to avoid damaging the pancreas itself.
What characterizes pancreatic proteases is their adaptability to function in a neutral pH, approximately 7-8, which mirrors the alkaline secretions that enter the small intestine. This unique feature enables a more refined level of protein digestion compared to gastric proteases. Their ability to target specific peptide bonds depending upon their amino acid context makes them a reliable choice throughout this article.
However, a disadvantage could be the relative specificity of some pancreatic proteases. Their targeted action, while effective, may lead to incomplete digestion if substrates contain unusual amino acid sequences not recognized by these enzymes.
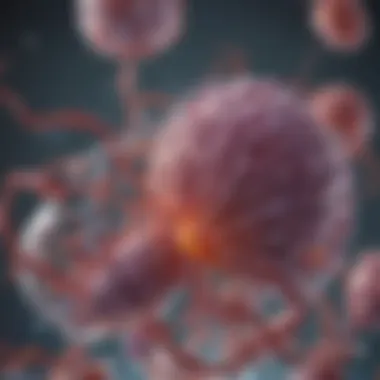
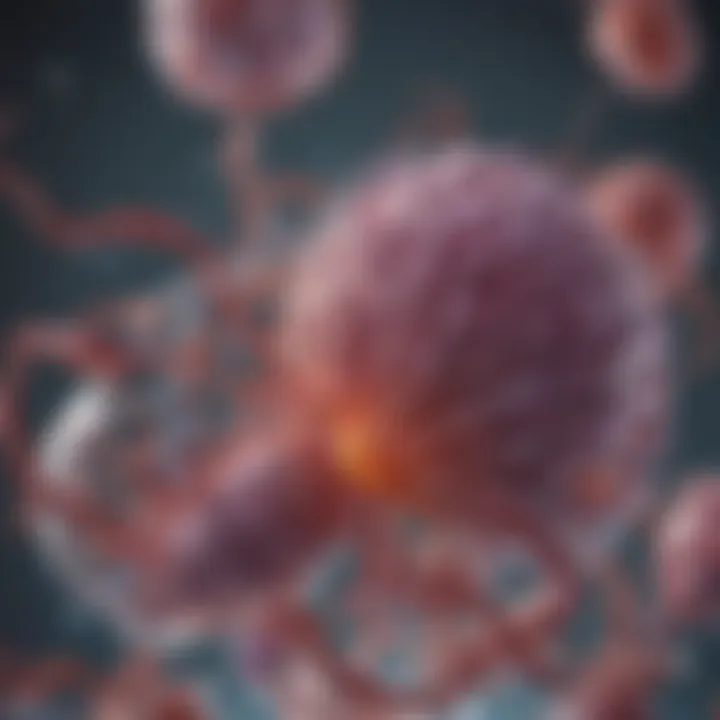
Enteropeptidases
Enteropeptidases, largely unrecognized heroes of the small intestine, initiate the cascade of pancreatic enzyme activation. Secreted by the intestinal mucosa, they play a crucial role in activating trypsinogen to trypsin. This activation is a critical step in the overall process of digestion, as activated trypsin subsequently activates other pancreatic proteases.
The vital characteristic of enteropeptidases is their ability to act specifically and efficiently in a very short time frame, thus amplifying the digestive process. Their unique feature lies in their requirement for an alkaline environment for optimal activity, coinciding with the transitional properties of the small intestine
However, one must consider the implications of enteropeptidase deficiencies. Insufficient levels can lead to malabsorption syndromes due to decreased pancreatic enzyme activation, showcasing their integral role in effective digestion.
The composition and functionality of digestive proteases are essential for transforming dietary proteins into usable nutrients, impacting overall health and metabolic efficiency.
Protease Regulation
Protease regulation is a critical aspect of maintaining homeostasis in biological systems. These enzymes, responsible for protein breakdown, must operate within tightly controlled parameters; otherwise, improper regulation can lead to detrimental effects, including disease development and cellular malfunction. Regulatory mechanisms ensure that proteases are activated only when needed and that their activity is modulated appropriately. This regulation is essential not just in digestion but also in various physiological processes such as blood coagulation, immune responses, and tissue remodeling.
Zymogen Activation
Proteases are usually synthesized as inactive precursors known as zymogens. This initial state prevents unwanted degradation of proteins. The activation of zymogens into their active forms, often through a specific cleavage process, is a finely tuned mechanism. When the conditions are right, such as a certain pH level or the presence of other enzymes, zymogens undergo conformational changes that expose their active sites. For instance, pepsinogen, a zymogen produced in the stomach, is activated to pepsin in the acidic environment, showcasing how the context of a biological system can dictate enzymatic activity.
Inhibitory Mechanisms
The inhibition of proteases is equally important in regulation. There are various types of inhibitors that help modulate protease activity to prevent excessive or inappropriate protein breakdown. Understanding these inhibitors offers insight into maintaining a balanced proteolytic environment in the body.
Serpins
Serpins, or serine protease inhibitors, are a prominent class of protease inhibitors known for their role in controlling serine proteases. These molecules are unique; they function by undergoing a significant conformational change upon binding to the active site of a protease, which effectively inactivates it. One key characteristic of serpins is their ability to inhibit multiple serine proteases, making them versatile regulators in various biological processes, including blood clotting and inflammation. Their structure allows for the rapid formation of stable complexes with their target proteases, providing a fast response to changes in the bodyβs needs, hence being beneficial in therapeutic contexts.
Other Inhibitors
Beyond serpins, there are numerous other inhibitors that play crucial roles in protease regulation. These include macromolecular inhibitors and cysteine protease inhibitors, which target various classes of proteases through distinct mechanisms. The versatility of these inhibitors lies in their specific binding interactions, which can block protease access to substrates. This specificity is a double-edged sword. On one hand, it affords fine-tuned control over proteolytic activity, which can be advantageous in therapeutic applications. On the other, it can lead to complications if the inhibitor's target is misregulated, resulting in conditions like autoimmune diseases.
"The regulation of proteases through various inhibitors showcases the intricate balance necessary for maintaining cellular health. Without this balance, pathways could spiral out of control, leading to severe consequences."
Overall, the regulation of proteases through mechanisms like zymogen activation and inhibition is essential for the proper functioning of biological systems. Understanding these processes provides valuable insights into disease mechanisms and therapeutic approaches.
Impact on Health and Disease
Understanding the role of proteases in human health and disease is crucial for various fields, from medical research to biotechnology. This section delves into the significant implications of protease activity, linking it directly to metabolic disorders, cancer, and autoimmune diseases. The enzymes that break down proteins are not only essential for digestion; they play pivotal roles in numerous physiological processes and pathological conditions. Consequently, comprehending how they function, and how they can be regulated or inhibited, becomes vital for developing effective therapeutic strategies.
Role in Metabolic Disorders
Proteases are involved not just in breaking down dietary proteins but also in regulating numerous metabolic pathways. They are indispensable for processing proteins that are crucial for energy balance and cellular communication. For instance, dysfunction in proteolytic activity can lead to metabolic disorders like obesity or diabetes.
- Insulin Regulation: Insulin is a peptide hormone that is vital for glucose metabolism. The proper proteolysis of preproinsulin into its active form requires a precise action of specific proteases. Disruption in this process could lead to insulin resistance or abnormal glucose levels.
- Hormonal Imbalances: Many hormones, including those related to metabolism such as leptin and ghrelin, need proteolytic cleavage to achieve their active forms. If proteases are not working optimally, this can cause significant disruptions in appetite regulation, energy expenditure, and ultimately, body weight management.
The key takeaway is that proteases serve as molecular scissors, slicing through peptide bonds to activate hormones and enzymes. Thus, any malfunction can cascade into metabolic dysregulation, exacerbating conditions like obesity, insulin resistance, or other metabolic syndromes.
Implications in Cancer
The connection between proteases and cancer is multi-faceted. On one hand, proteases are essential for normal cell growth and division. On the other hand, certain proteases, often termed "cancer-associated proteases," can promote tumor progression and metastasis. Understanding their dual role is imperative for cancer research.
- Tumor Microenvironment: Proteases like matrix metalloproteinases (MMPs) modify the extracellular matrix, facilitating tumor growth and invasion. They enable cancer cells to breach the surrounding tissue and spread to other organs, essentially paving the way for metastasis.
- Therapeutic Targets: Researchers are exploring protease inhibitors as potential cancer therapies. By targeting these enzymes, itβs possible to inhibit cancer cell invasion and metastasis effectively. Ongoing studies aim to determine the best ways to leverage protease inhibition to improve patient outcomes in various cancer types.
To sum it up, while proteases are essential for numerous physiological roles, their dysregulation or over-activation can lead to severe consequences, including the advancement of malignancies.
Autoimmune Diseases
In autoimmune diseases, the body's immune system mistakenly attacks its own tissues. The role of proteases in this process is gaining attention, particularly how they can modulate immune responses. Uncontrolled proteolytic activity can result in inflammation and tissue damage, illustrating why understanding protease function is increasingly important.
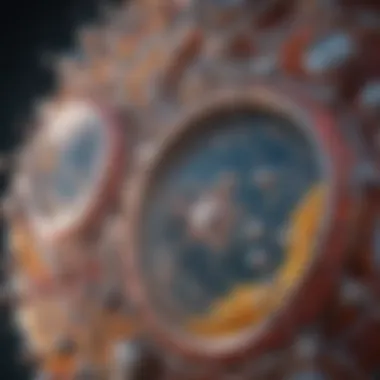
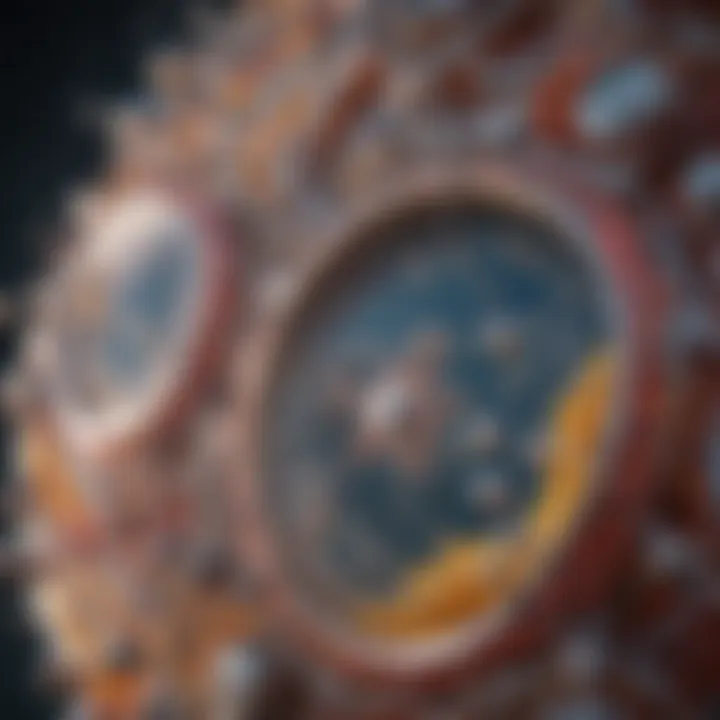
- Inflammatory Responses: Certain proteases can activate pro-inflammatory cytokines, heightening immune responses and exacerbating autoimmune conditions such as rheumatoid arthritis and lupus. For instance, the action of cysteine proteases is often elevated during these states, aggravating inflammation.
- Therapeutic Insights: There is potential for developing protease inhibitors to manage autoimmune diseases. By inhibiting specific proteases, it is conceivable to temper the excessive immune responses that characteristically accompany these disorders. Ongoing research is essential to pinpoint which proteases are most critical in these processes and how best to target them.
In overview, proteases are not merely digestive enzymes; they are pivotal players in the roles of health and illness. By informing our understanding of metabolic disorders, cancer progression, and autoimmune responses, proteases emerge as significant targets for therapeutic intervention, underscoring their integral place in both health and disease.
Applications in Biotechnology
Biotechnology extensively leverages protease digestion, intertwining fundamental biological processes with innovative applications. Understanding how proteases operate allows industries to enhance their products and develop new methodologies; from creating more efficient detergents to pioneering pharmaceuticals. The versatile nature of proteases brings forth numerous benefits across various sectors, raising opportunities for improved product quality and efficiency.
Industrial Uses
Food Industry
Proteases play a vital role in the food industry, chiefly through their action in tenderizing meat and improving protein digestibility. These enzymes enhance flavor and texture, making foods more palatable. For example, enzymes like papain from papaya and bromelain from pineapple are widely used. The key characteristic of the food industry is its adaptability to consumer preferences, and employing proteases is a winning strategy.
- Advantages: Proteases reduce cooking time and improve texture by breaking down protein structures. This not only enhances the culinary experience but also provides health benefits by making proteins easier to digest.
- Disadvantages: However, there can be over-tenderization with excessive use, potentially ruining the texture of certain dishes. Proper dosage is crucial to balance efficiency and quality.
Detergents
In detergents, proteases are game changers. They break down protein stains effectively, proving useful in laundry and dishwashing products. Specific proteases such as subtilisin are designed to target stains from blood, grass, and food, making them highly effective. The key characteristic of detergent applications is their efficiency in varied conditions; proteases work at different temperatures and pH levels.
- Advantages: This allows for lower temperature washes, saving energy and reducing wear on fabrics.
- Disadvantages: One downside is the potential for allergic reactions in some sensitive individuals, which may limit marketability in certain regions.
Pharmaceuticals
Proteases find critical applications in pharmaceuticals, particularly in drug development and production processes. They can be used to produce peptide drugs, making their functionality crucial in large-scale pharmaceutical synthesis. The characteristic that stands out in this area is their ability to catalyze reactions under mild conditions, providing better yields.
- Advantages: Their precision in hydrolyzing specific peptide bonds leads to the formation of therapeutically relevant compounds efficiently.
- Disadvantages: Nonetheless, protease inhibitors may be necessary to control their activity during drug formulation, adding layers of complexity to their usage.
Research Applications
Protein Sequencing
Protein sequencing heavily relies on protease digestion to accurately identify and map amino acid sequences. The process breaks proteins into manageable peptide fragments, facilitating analysis. The key feature of protein sequencing is its ability to provide detailed insights into the protein structure, making it invaluable in various research arenas.
- Advantages: This offers a better understanding of protein function and interactions, aiding drug design and discovery processes.
- Disadvantages: One possible drawback is the requirement of advanced technology and expertise, which may not be universally accessible, thus limiting its widespread adoption in certain research settings.
Gene Expression Studies
Proteases play a supportive role in gene expression studies, where their digestion of protein samples allows for accurate assessments of gene function and regulation. This application highlights another facet of protease utility. The unique aspect here is their influence on post-translational modifications, revealing how these changes can affect gene expression.
- Advantages: The ability to correlate gene expression with protein activity holds potential for breakthroughs in understanding diseases and developing targeted therapies.
- Disadvantages: However, the need for specificity in protease selection to avoid unwanted digestions can complicate experimental designs, requiring more resources and careful planning.
Recent Advances and Future Directions
The landscape of protease research is continually evolving, unveiling a realm of advances that hold promise for the future of biotechnology and medicine. As scientists explore the intricate roles of proteases in various biological processes, the potential applications become clearer. Understanding recent advancements not only sheds light on protease functions but also offers insights into therapeutic prospects and genetic manipulation techniques.
Protease Inhibitors in Therapy
Protease inhibitors have emerged as a focal point in the development of therapeutic strategies for various diseases. These molecules intercede in the protein digestion process by inhibiting the action of specific proteases, thus controlling the breakdown of proteins within the bodyβan approach that has proven beneficial in treating conditions like HIV and certain types of cancer.
The integration of protease inhibitors is particularly noteworthy in antiviral therapies. For example, the use of ritonavir and lopinavir has drastically changed the management of HIV. Their mechanism involves blocking the viral protease, thereby halting viral replication. These treatments exemplify the intersection of biotechnology and therapeutic intervention, emphasizing the role of proteases beyond mere digestion.
Moreover, protease inhibitors have gained traction in oncology. This is largely due to their potential to modulate the tumor microenvironment. By allowing proteins that suppress cell death to accumulate, these inhibitors can enhance the efficacy of existing cancer treatments. Thus, research is focusing on understanding the specificity of these inhibitors to refine their application further.
"The design of selective protease inhibitors holds the key to advancing targeted therapies, reducing side effects, and improving patient outcomes."
Applications in Genetic Engineering
In the domain of genetic engineering, proteases play a vital role. Their ability to modify peptides allows for advancements in precision editing techniques. For example, researchers leverage specific protease activities to remove unwanted fusion tags from recombinant proteins. This facilitates the study of protein functions without interference from additional sequences.
Another exciting application is the utilization of proteases in creating genetically modified organisms (GMOs). By enabling targeted protein degradation, researchers can understand gene functions better and create crops with enhanced traits like increased resistance to pests or improved nutritional profiles.
Further, the CRISPR-Cas9 system is also seeing integration with protease technology. The ability of certain proteases to cleave specific peptide bonds presents new avenues for enhancing the efficiency and specificity of gene-editing tools. With the ever-growing demands for efficient plant and animal breeding, such approaches are likely to gain momentum.