MCF10 Cells in Breast Cancer Research: A Comprehensive Guide
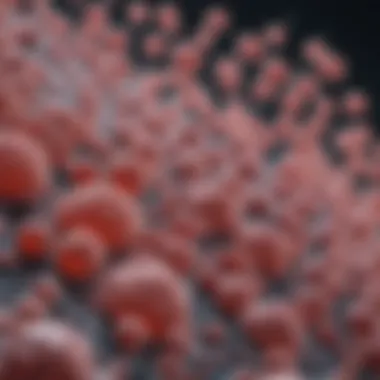
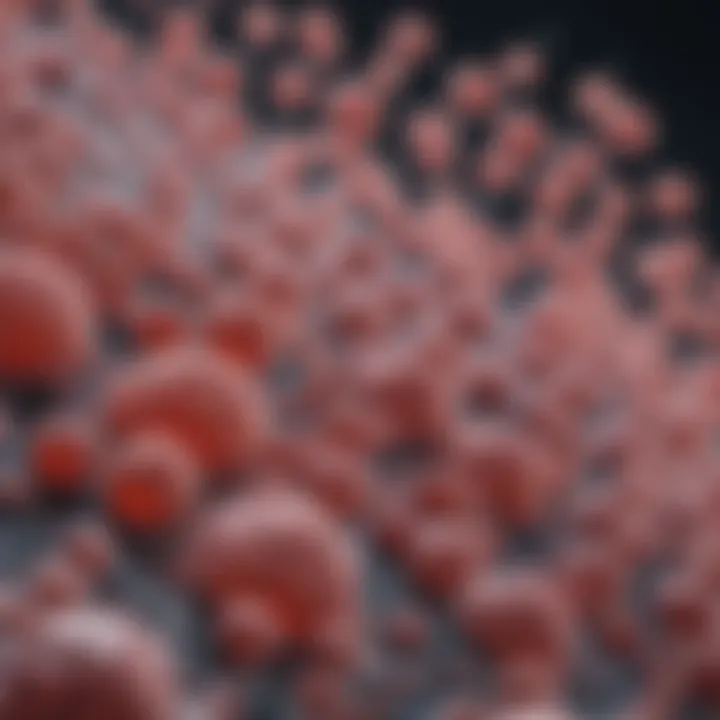
Intro
MCF10 cells have become a fundamental resource in cancer research, particularly in the context of breast cancer studies. Originally derived from human breast tissue, these cells are characterized by their non-tumorigenic properties, making them a suitable model for both in vitro and in vivo research. The significance of MCF10 cells extends beyond their biological characteristics; they serve as a bridge between normal breast epithelial behavior and malignant processes, which is crucial for understanding cancer biology more accurately.
The exploration of MCF10 cells allows researchers to investigate intricate biological mechanisms underlying breast cancer, including cellular differentiation, proliferation, and response to various treatments. By utilizing MCF10 cells, scientists can analyze cell signaling pathways, which are pivotal in tumor progression and metastasis. This article investigates their attributes, applications, and the implications these cells have in advancing current breast cancer research and therapeutic strategies.
MCF10 cells also present a practical advantage in research settings by offering a controllable environment for experimentation. With an extensive range of derived cell lines, including those modified to express oncogenes or silenced tumor suppressor genes, they provide a versatile platform for studying differing aspects of cancer dynamics. Therefore, this comprehensive examination aims to highlight the pivotal role MCF10 cells play in uncovering the complexities of cancer biology, ultimately contributing to scientific progress in developing new treatment modalities.
Preamble to MCF10 Cells
The introduction of MCF10 cells serves as a foundational aspect of understanding their role in cancer research. MCF10 cells represent a unique and significant model for the study of breast cancer biology because of their non-tumorigenic properties. Their ability to emulate normal breast epithelial cells makes them a valuable tool for a variety of scientific inquiries. In this article, we underscore the relevance of MCF10 cells, focusing on their origin, basic characteristics, and significance in the research field.
Origin and Development
MCF10 cells were derived from human breast tissue, specifically from a female patient undergoing reduction mammoplasty. The original cell line was established by Dr. H. R. J. G. Awad and his colleagues at the University of California, Los Angeles (UCLA) in the late 1980s. Unlike other cancer cell lines, MCF10 cells are non-tumorigenic, which means they do not form tumors when injected into immunodeficient mice. This characteristic ensures that the cells maintain a physiological profile similar to that of normal breast epithelial cells, allowing researchers to study under conditions that closely mimic in vivo environments. Such origins provide essential insights into the complexities of breast tissue and the differences between healthy and malignant cells.
Basic Characteristics
MCF10 cells possess several distinct characteristics that set them apart from other cell lines. They grow in a well-defined, serum-free medium, which is crucial for preserving their phenotype. The cells typically form acini-like structures when cultured in three-dimensional (3D) matrices, mirroring the architecture of breast tissue. Their growth is influenced by various factors such as hormonal signaling and extracellular matrix components. Additionally, MCF10 cells are known to express specific markers typical of normal luminal epithelial cells, like cytokeratin 18, which helps characterize cellular responses to stimuli in a controlled experimental setup.
Significance in Research
The use of MCF10 cells in research is profoundly significant due to their applications in the study of breast cancer mechanisms. Being non-tumorigenic allows researchers to explore how breast cells transition from a normal state to one characterized by malignancy. These cells serve as a benchmark for assessing drug efficacy and cellular behavior under various treatment scenarios. Moreover, they provide insights into critical pathways involved in tumor development, hormone responsiveness, and genetic expression. For example, studies using MCF10 cells have revealed information about oncogenes and tumor suppressor genes, which are essential for understanding cancer biology.
"MCF10 cells are crucial for deciphering breast cancer pathways, facilitating the development of targeted therapies that aim to improve patient outcomes."
Properties of MCF10 Cells
The properties of MCF10 cells play a pivotal role in understanding their utility in cancer research. These cells serve as a foundational model for studying breast epithelial biology and tumorigenesis. MCF10 cells are often viewed as a reference standard due to their non-tumorigenic nature and their ability to mimic normal breast epithelial cells, thus providing insights that are often impossible to achieve with tumor-derived cell lines. Exploring these properties not only aids in basic cancer biology research but also enhances the development of potential therapeutic strategies.
Cell Morphology
MCF10 cells exhibit distinct morphological characteristics that are essential for their classification and study. Typically, these cells are non-adherent and grow in a monolayer. They display a cuboidal shape with tight junctions present between neighboring cells, which is indicative of their epithelial origin. This particular morphology is crucial, as it allows them to closely resemble normal mammary epithelial cells.
The ability to form acini-like structures in three-dimensional cultures further exemplifies their usefulness. Such structures provide insights into cellular organization and the behavior of breast epithelial cells in a more physiologically relevant environment. Observing these morphologies under different experimental conditions can help researchers discern the effects of various stimuli or drugs on cell behavior and structure.
Growth Characteristics
The growth characteristics of MCF10 cells are another critical aspect to consider. These cells typically proliferate at a moderate rate, which is important for maintaining distinct phases of cell cycle studies. They rely on specific growth factors, including epidermal growth factor and insulin, to thrive in culture.
MCF10 cells exhibit contact inhibition, a normal cellular response where cell proliferation ceases when cells are densely packed. This characteristic is important for research, as it highlights the regulatory mechanisms that are often altered in cancerous cells. Moreover, the cells' ability to form monolayer cultures allows for standardized assays, essential for drug testing and toxicology studies.
Gene Expression Profiles
Gene expression profiling of MCF10 cells reveals a significant landscape that underscores their relevance in cancer research. The expression patterns of MCF10 cells can be compared to those of malignant counterparts to identify key differences that may contribute to tumorigenesis. Genes implicated in cell cycle regulation, apoptosis, and cell adhesion are particularly noteworthy.
High-throughput sequencing techniques have allowed for a deeper understanding of these expression profiles. Such analyses often highlight the cellular pathways that are upregulated or downregulated in response to various external factors. For instance, the expression of estrogen receptors can provide insights into how MCF10 cells respond to hormonal stimuli, which is a crucial aspect of breast tissue behavior and pathology.
Studies indicate that analyzing these gene expression profiles has the potential to identify novel targets for breast cancer therapies.
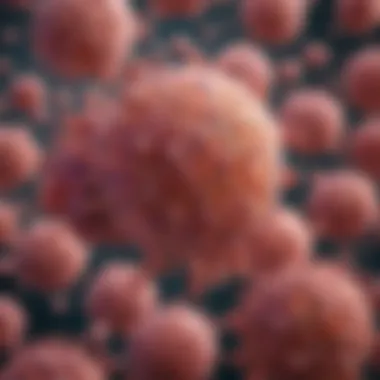
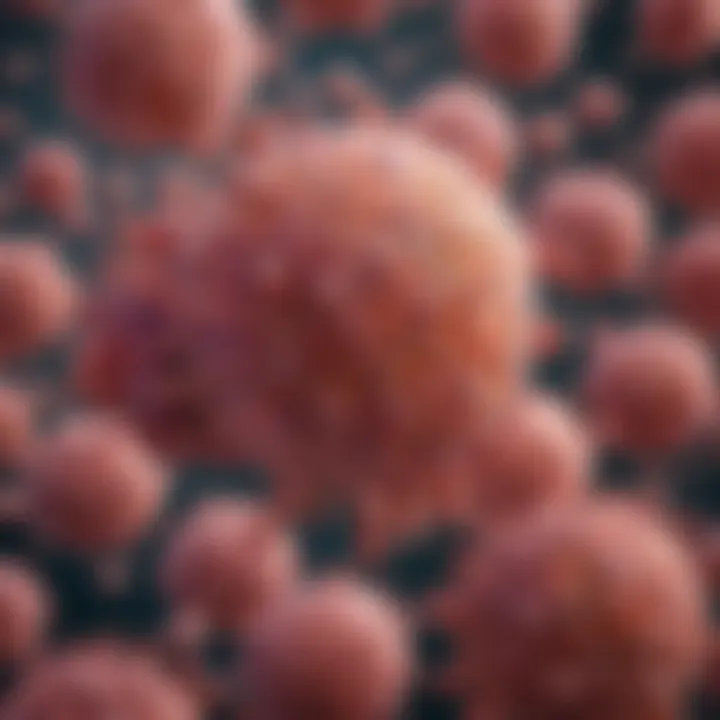
Applications of MCF10 Cells
The applications of MCF10 cells in cancer research are extensive and critical. Their unique characteristics make them indispensable for studying the mechanisms of breast cancer, drug responses, and toxicity. Research using MCF10 cells allows scientists to gain insights that can guide the development of innovative therapeutic strategies and improve our understanding of cancer biology. Below, we explore three significant applications: their roles in breast cancer research, drug discovery and testing, as well as toxicology assessments.
Breast Cancer Research
Breast cancer research leverages MCF10 cells due to their non-tumorigenic nature and ability to retain epithelial properties. MCF10A, the parental cell line, represents normal breast epithelial cells, making it an ideal model to study the transition from normalcy to malignancy. The advantages include:
- Ability to model the early stages of breast cancer.
- Use in studies of tumor microenvironments and cell signaling pathways.
- Insights into genetic alterations that lead to breast cancer progression.
Researchers can employ MCF10 cells to investigate the impacts of oncogenes and tumor suppressor genes, such as HER2 and p53. Furthermore, studies examining interactions with hormones, particularly estrogen, provide valuable understanding of how these factors influence breast cancer development.
Drug Discovery and Testing
In the realm of drug discovery, MCF10 cells serve as a platform for screening potential anti-cancer drugs. Their characteristics allow scientists to study drug efficacy in a controlled environment. The importance of using MCF10 cells includes:
- Evaluation of drug responses in a model that mimics normal breast tissue.
- Testing combinations of therapies to enhance effectiveness.
- Opportunity to develop personalized medicine approaches based on individual responses.
Moreover, researchers can utilize high-throughput screening methods to assess vast libraries of compounds, pinpointing candidates that show promising results against specific cancer targets. Drug tests conducted on MCF10 cells provide preliminary data necessary before moving to live tissues or clinical trials.
Toxicology Assessments
Toxicology assessments using MCF10 cells offer insights into the safety profile of chemicals and pharmaceuticals. These studies help determine how different substances impact normal breast epithelial cells compared to malignant cells. The relevance of MCF10 cells in toxicology includes:
- Understanding cytotoxicity and cell viability in response to various agents.
- Evaluating the potential harm of environmental toxins on breast tissue.
- Providing data for regulatory assessments regarding safety of new compounds.
By exposing MCF10 cells to potential toxins, researchers can conduct dose-response studies that inform both safety evaluations and possible therapeutic windows. These findings are crucial in ensuring that new drugs or chemicals do not adversely harm healthy cells while targeting disease.
MCF10 cells stand at the forefront of breast cancer research, offering essential insights into both oncogenic processes and the impact of therapeutic interventions.
Overall, MCF10 cells serve as a versatile tool in a variety of applications within cancer research. Their contributions extend from the foundational understanding of cancer biology to practical applications in drug development and safety assessment.
MCF10 Cell Variants
The variants of MCF10 cells are essential for understanding the nuances of breast cancer research. Studying these variants allows researchers to investigate distinct characteristics and behaviors that arise from different genetic modifications or environmental factors. These variants serve as critical tools for exploring the progression of cancer and also for testing potential therapeutic interventions. The significance of MCF10 cell variants in research cannot be underestimated; they provide a platform to study transformation and invasion relevant to human breast cancer.
MCF10A Cells
MCF10A cells are the parental line of the MCF10 cell series. This non-tumorigenic breast epithelial cell line retains many characteristics of normal breast tissue. They are often used as a baseline model for comparison against other variants when interpreting results. The growth of MCF10A cells is dependent on growth factors present in their culture medium, signifying their reliance on external signaling, much like normal tissue in vivo. They exhibit a notably stable karyotype which is crucial for experiments that demand consistent and reproducible cellular behavior. This stability enables researchers to explore mechanisms of cell cycle regulation and tissue organization within a controlled environment.
MCF10AT Cells
MCF10AT cells are an important derivative of MCF10A, engineered to express the oncogene HRAS. This variant undergoes transformation and exhibits characteristics akin to early-stage breast cancer cells. The progression from MCF10A to MCF10AT provides insights into the role of oncogenes in breast cancer initiation. Researchers utilize MCF10AT cells to assess cellular responses to various treatment modalities and to understand altered cell signaling pathways arising from oncogenic changes. Their ability to proliferate faster and invade surrounding tissue makes them a powerful model for studying tumor formation and metastasis in breast cancer.
MCF10CA1 Cells
MCF10CA1 cells represent a more advanced variant, demonstrating characteristics of late-stage breast cancer. This cell line was derived from MCF10AT cells and exhibits aggressive growth properties and a heightened invasive potential. MCF10CA1 cells are particularly valuable when investigating therapeutic resistance and the mechanisms that facilitate cancer progression. They allow scientists to probe the interactions between tumor cells and the tumor microenvironment. Their utility in drug screening is valuable for testing agents that target late-stage breast cancer behaviors. Understanding this variant contributes to identifying strategies for overcoming treatment resistance in clinical settings.
Methodologies for Studying MCF10 Cells
Understanding MCF10 cells necessitates the application of various methodologies that are pivotal in cancer research. Using precise and effective techniques enables researchers to decipher the intricate biological behaviors of these cells, which may replicate certain characteristics of breast cancer.
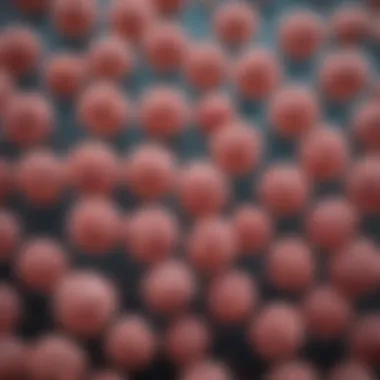
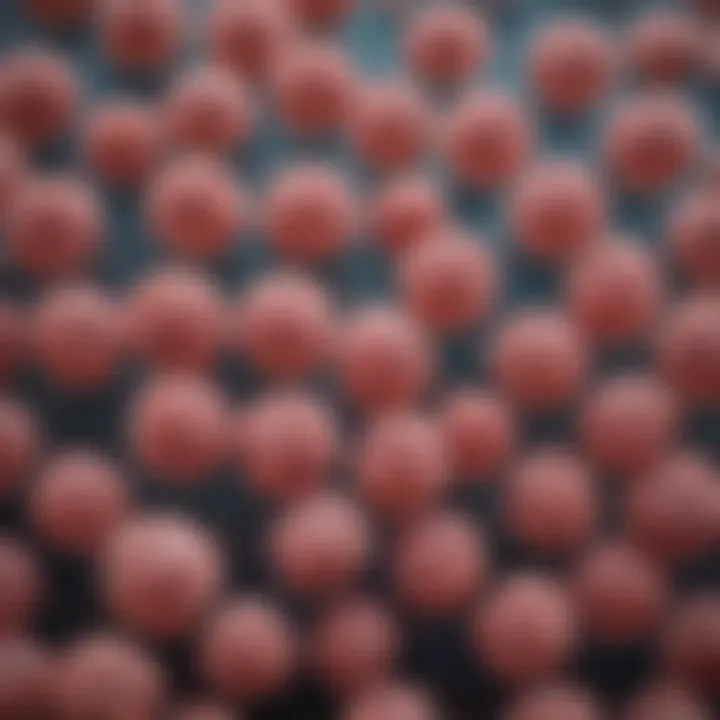
To ensure robust and reliable experimentation, methodologies must be adapted to suit the distinctive qualities of MCF10 cells. The following sections delve into the critical techniques involved in studying these cells, emphasizing the paramount importance of each methodology in illuminating the biological processes underpinning breast cancer.
Cell Culture Techniques
Cell culture is the foundational methodology in studying MCF10 cells. Mastery over culture techniques directly influences the reproducibility and reliability of results. To start, MCF10 cells require specific growth conditions that mimic their natural environment as closely as possible. This includes using appropriate media like DMEM/F12 supplemented with growth factors such as epidermal growth factor (EGF) and insulin. Such conditions foster the maintenance of their epithelial characteristics.
Moreover, it is crucial to monitor the confluence of the cell layer to avoid differentiation or overgrowth, which could alter experimental outcomes. Passaging methods, where cells are subcultured to maintain exponential growth, must also be carefully optimized. Practicing sterile techniques and controlling variables like temperature and CO2 levels are essential to minimize contamination and ensure cell viability.
Transfection Protocols
Transfection of MCF10 cells allows for the introduction of foreign DNA and RNA, enabling the analysis of gene function and protein expression. This process is vital for respiratory studies, gene editing and investigating cancer-related pathways. The choice of lipofection or electroporation methods often depends on the specific objectives of the research.
For example, lipofection is generally less invasive and thus tends to yield higher cell viability. Commercial kits like Lipofectamine and FuGENE are frequently employed to enhance the transfection efficiency. However, optimizing the ratio of DNA to transfection reagent is a critical step that can significantly affect outcomes. Post-transfection time frames should be adjusted to allow for proper expression of the transfected material, ensuring data accuracy.
Assays for Functional Studies
Conducting functional assays is imperative to elucidate the specific biological activities of MCF10 cells in a cancer context. Common assays include proliferation assays, migration and invasion assays, and apoptosis detection assays. Each of these provides insights into the behavior and characteristics of cells under study.
For proliferation studies, assays like MTT (tetrazolium salt) or BrdU incorporation can quantitatively measure cell growth. Migration assays, such as the Boyden chamber or wound healing assays, evaluate the invasive potential of transformed MCF10 variants. Lastly, apoptosis assays using Annexin V and propidium iodide staining allow researchers to assess the effects of treatments on cell survival.
Through these methodologies, researchers can systematically investigate and unravel the complexities of MCF10 cells, enhancing our understanding of breast cancer biology and potential therapeutic avenues. Each method serves as a vital tool in the intricate puzzle of cancer research.
Insights into Cancer Biology
Understanding cancer biology is crucial in the development of effective therapeutic strategies. MCF10 cells serve as an important tool in studying various aspects of breast cancer biology. Their non-tumorigenic characteristics make them ideal for uncovering mechanisms that contribute to tumor formation, growth, and maintenance. This section will discuss significant elements such as tumor suppression mechanisms, hormonal influences, and the role of the microenvironment. Each of these factors plays a vital part in the complex network of interactions that drive cancer progression.
Tumor Suppression Mechanisms
MCF10 cells allow researchers to explore tumor suppression mechanisms in a controlled setting. The study of these mechanisms is particularly relevant for understanding how normal cells evade the transformation into cancerous cells. Key proteins involved in tumor suppression, such as p53 and Rb, can be studied using these cells to determine how alterations in their activities lead to malignancy. Investigating genetic changes and signaling pathways offers insights helpful for developing targeted therapies. Furthermore, it appears that MCF10 cells retain certain pathways that remain active in normal breast epithelium. This indicates that, under certain conditions, normal breast epithelial cells can indeed suppress tumorigenic characteristics effectively.
Hormonal Influence on Cell Behavior
Hormones significantly affect cell behavior, particularly in breast epithelium. MCF10 cells respond to hormones like estrogen and progesterone, which is essential for studying hormone-related cancers. Research has shown that estrogen signaling pathways can promote cell proliferation and affect apoptosis, creating an environment conducive to breast cancer development. Understanding how these hormonal signals influence MCF10 cells enhances our comprehension of breast cancer's dependency on hormonal fluctuations. This knowledge may inform treatment strategies, especially in recurrent or metastasized hormone-sensitive breast cancer cases.
Role of the Microenvironment
The tumor microenvironment is critical in cancer biology. MCF10 cells provide a platform to examine interactions between tumor cells and their surrounding stroma. Studies focus on how extracellular matrix components and signaling molecules within this microenvironment dictate cellular behavior. For example, the presence of specific fibroblasts, immune cells, and matrix components can modulate MCF10 cell growth and differentiation. These interactions may promote tumor progression and chemoresistance. Understanding this dynamic emphasizes the need to consider therapeutic approaches that target both the tumor cells and their microenvironment.
Through these explorations, MCF10 cells continue to prove their value in dissecting the complexities of cancer biology, ultimately supporting advancements in treatment options.
Advancements in Techniques Involving MCF10 Cells
The developments in techniques involving MCF10 cells represent a crucial aspect in cancer biology research. These advancements not only enhance our understanding of breast cancer but also improve experimental methodologies. MCF10 cells provide a versatile background for testing genetic modifications, drug interactions, and other biological phenomena. When researchers employ advanced technologies, they can garner insights that were previously unattainable.
CRISPR-Cas9 Applications
CRISPR-Cas9 technology has revolutionized the field of genetics. It allows precise edits in the genome of MCF10 cells. By using this tool, scientists can knock out specific genes to study their roles in cancer progression. This approach enables the exploration of pathways that contribute to tumorigenesis and potentially identifies novel targets for therapy. Moreover, the efficiency of CRISPR in MCF10 cells means that researchers can generate various cell lines with specific genetic alterations. These cell lines can serve as essential models for drug testing and the investigation of cancer mechanisms.
High-Throughput Screening Methods
High-throughput screening is a vital method in modern research, particularly in biomedical fields. This technique allows researchers to analyze many compounds rapidly. When applied to MCF10 cells, high-throughput screening helps in identifying potential therapeutic agents with efficacy against breast cancer. Researchers can screen thousands of drugs simultaneously, enabling the identification of promising candidates much faster than traditional methods. This approach accelerates the drug discovery process, leading to quicker therapeutic options for patients. However, it requires careful optimization to ensure that results are reproducible and relevant.
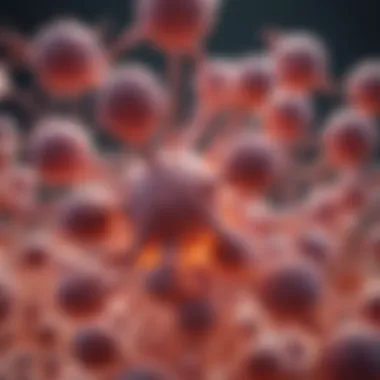
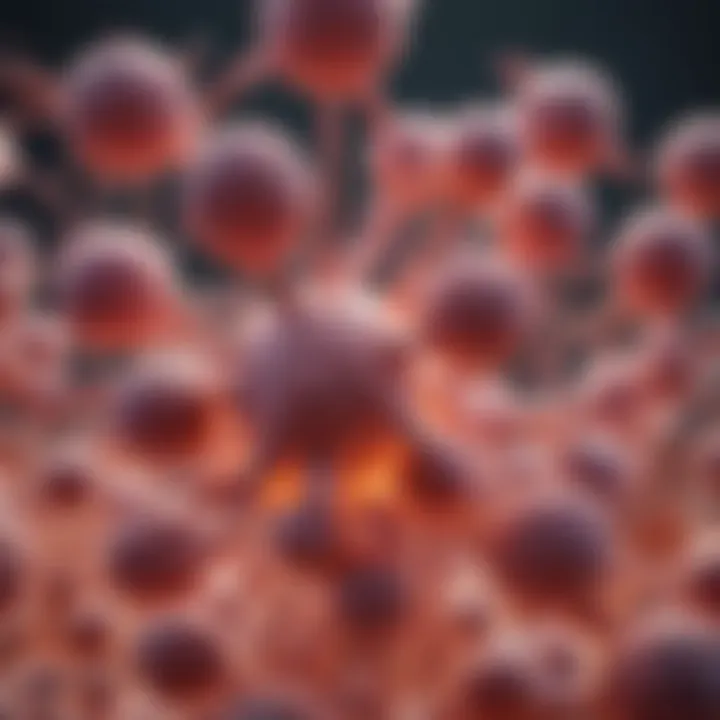
Omics Technologies
Omics technologies, such as genomics, proteomics, and metabolomics, have enhanced our understanding of MCF10 cells in cancer research. These technologies allow comprehensive analysis of cellular components. In the context of MCF10 cells, genomics can reveal mutations associated with breast cancer, while proteomics can identify the expression levels of critical proteins involved in cancer pathways. Metabolomics provides insights into the metabolic changes that occur in cancer cells compared to normal cells. Integrating multiple omics approaches can yield a holistic view of cancer biology and contribute to personalized medicine strategies.
The integration of advanced techniques in MCF10 research holds the potential to uncover previously unknown mechanisms of cancer, facilitating breakthroughs in treatment.
Limitations of MCF10 Cell Studies
Understanding the limitations of MCF10 cell studies is crucial in cancer research. While these cells provide a valuable model, acknowledging their constraints helps guide research design and interpretation of results. The limitations help inform researchers of the potential variances that may affect findings, ultimately leading to more robust conclusions.
Differences from In Vivo Systems
MCF10 cells are derived from human breast epithelial cells, providing a cell line that closely mimics normal breast tissue. However, their in vitro nature means that they cannot fully replicate the complex environment of a living organism.
Key differences include:
- Microenvironment: In vivo systems contain a dynamic microenvironment with various cell types, extracellular matrix components, and signaling molecules. MCF10 cells lack this multifaceted interaction, potentially skewing how cells respond to stimuli.
- Immune Response: Tumor microenvironments in living organisms also include immune cells that can influence tumor behavior. MCF10 cells do not exhibit an immune response, which may lead to inaccuracies in understanding immune-mediated effects on tumor development and treatment responses.
- Genetic Diversity: Each individual has unique genetic makeup. MCF10 cells lack the genetic diversity seen in human populations, which may limit applicability across different genetic backgrounds.
These differences underscore the necessity for careful extrapolation of in vitro findings to real-world scenarios.
Potential Artifacts in Experimental Results
While MCF10 cells have provided significant insights, researchers must be aware of potential artifacts that can arise during experimentation. Such artifacts may mislead conclusions or misrepresent biological realities.
Consider these common artifacts:
- Cell Line Variability: Culturing conditions, such as media compositions and passage numbers, can lead to variability in cell behavior. This inconsistency can affect gene expression and growth characteristics.
- Selective Pressure: Over time, prolonged culture of MCF10 cells may create selective pressures that induce genetic mutations or alter phenotypic traits. As a result, the behavior of these cells may deviate from their original characteristics.
- Lack of Cell-Cell Interactions: MCF10 cells grown in isolation do not experience the interactive influences from neighboring cells. This isolation can affect signaling pathways and cellular responses commonly observed in a multicellular environment.
These artifacts emphasize the need for integrative approaches, combining in vitro studies with in vivo models to enhance the validity of research findings.
Recognizing these limitations encourages critical evaluation of MCF10 cell studies, promoting a more comprehensive understanding of their role in cancer research.
Future Directions for MCF10 Cell Research
Future directions in MCF10 cell research are essential for advancing our understanding of breast cancer and improving therapeutic strategies. Research in this area paves the way for integrating innovative methodologies and investigating new treatment avenues. This section outlines two critical future directions: integrating new technologies and exploring combination therapies. Each is vital for extending the functional application of MCF10 cells in cancer research.
Integrating New Technologies
The integration of new technologies can significantly enhance the research capabilities involving MCF10 cells. Advanced methods like single-cell sequencing and multiplexed imaging allow for in-depth analysis of cell behavior and characteristics. These methodologies facilitate a better understanding of cellular heterogeneity in tumors, particularly the variegation observed in cancer cells. In addition, techniques such as CRISPR-Cas9 gene editing can be employed on MCF10 cells to elucidate the roles of specific genes in breast cancer progression. This approach leads to targeted interventions, allowing researchers to explore precise molecular mechanisms.
Another innovative move is the application of organ-on-a-chip technologies. MCF10 cells can be utilized in these platforms to create more realistic models of the human breast microenvironment. Such technologies enable researchers to study the dynamic interactions between cells and extracellular matrices under various conditions, providing deeper insights into tumor progression and response to therapies.
Moreover, high-throughput screening combined with MCF10 cells can expedite drug discovery processes. Researchers can utilize these cells to assess thousands of compounds rapidly, identifying potential therapeutic candidates more efficiently. Such advancements not only improve the efficiency of drug screening but also promote a more systematic approach towards the identification of new treatment modalities.
"The incorporation of new technologies will undoubtedly change the landscape of breast cancer research. It allows us to approach questions with unprecedented accuracy and depth."
Exploring Combination Therapies
Exploring combination therapies presents another promising direction for MCF10 cell research. Standalone treatments often fall short due to the complex nature of cancer. Thus, combination therapies can potentially enhance treatment efficacy and mitigate resistance in tumors.
MCF10 cells provide a platform for examining various drug combinations. By employing these cells, researchers can test different chemotherapeutics, targeted therapies, and adjunct treatments in tandem. For instance, combinations of HER2 inhibitors with immune checkpoint blockers can be evaluated efficiently using MCF10 models.
The concept of synergy among drugs is particularly noteworthy. Understanding how certain drugs interact can lead to more robust treatment plans. MCF10 cells can help elucidate the optimal ratios and timing for these therapies, leading to improved patient outcomes. Moreover, pinpointing specific pathways affected by drug combinations can also lead to more personalized treatment strategies.
In addition, exploring the effects of various combination therapies on tumor microenvironment interactions is crucial. MCF10 cells can be incorporated into studies examining how different treatments influence immune cell infiltration or angiogenesis. Such investigations can provide insights that will guide future clinical trials and therapeutic applications.
In summary, both integrating new technologies and exploring combination therapies are critical future directions for MCF10 cell research. These strategies not only promise to enhance our understanding of breast cancer but also facilitate the development of effective therapeutic innovations.