Exploring Microscope Objectives in Science and Education
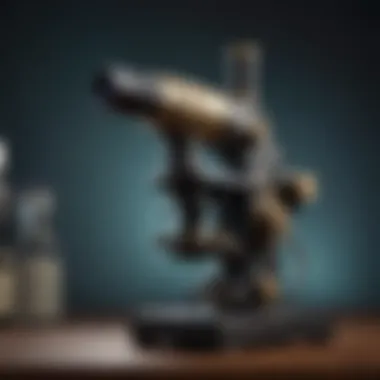
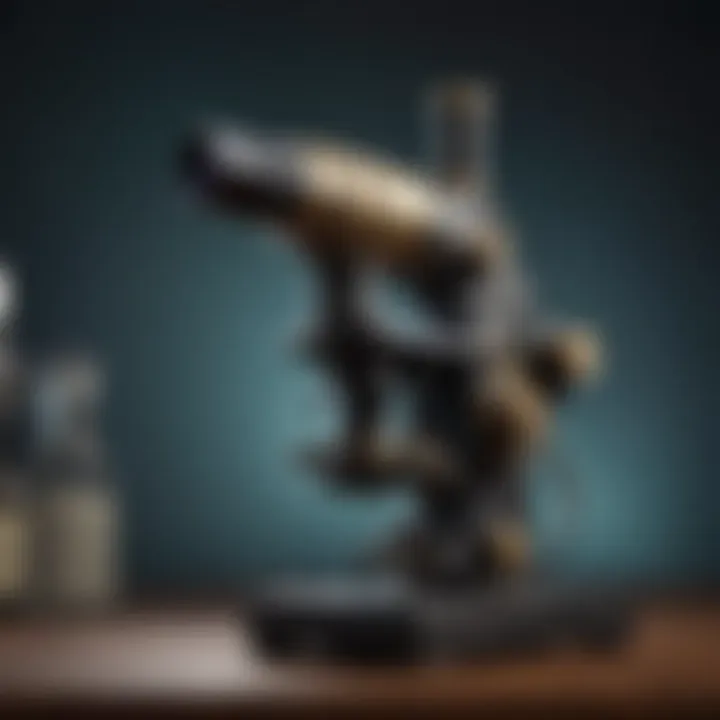
Intro
Microscopes have long been pivotal tools in the realm of scientific inquiry. Their primary purpose is to magnify objects too small for the naked eye, allowing researchers and students alike to explore the microscopic world. This article delves into the diverse objectives of microscopes, highlighting their importance in research and education. It will also examine the evolution of these instruments, the varied types available, and their operational principles. Furthermore, advancements in technology that enhance the capabilities of microscopes will be discussed.
This exploration aims to provide readers with a comprehensive understanding of microscopes, showcasing how these instruments are fundamental to the advancement of various scientific disciplines.
Research Overview
Summary of Key Findings
This article presents several key findings regarding the objectives and applications of microscopes. Firstly, it emphasizes that microscopes facilitate breakthroughs in biology, medicine, geology, and materials science. The capacity to scrutinize details such as cell structures or mineral compositions reveals crucial information that furthers academic and practical knowledge.
Secondly, the document outlines the historical development of microscopes, tracing their journey from simple lenses to sophisticated digital devices. This evolution underscores the continuous drive for enhanced precision and clarity in scientific observation.
Research Objectives and Hypotheses
The main objectives of this research include:
- To explore the variety of microscope types and their specific uses within different fields.
- To analyze the principles of microscope operation, showing how magnification and resolution contribute to effective observation.
- To assess the technological advancements in microscopy, including digital imaging and automation.
These objectives support the hypothesis that understanding the functionality and objectives of microscopes is essential for maximizing their use in research and education.
Methodology
Study Design and Approach
The research adopts a comprehensive literature review approach. It examines academic texts, articles, and recent advancements in microscopy to construct a detailed narrative. This design allows for a thorough investigation of both the historical context and contemporary applications.
Data Collection Techniques
Data used in the article are collected primarily from reputable sources such as journals, encyclopedias, and educational platforms. The integration of this information fosters a rich understanding of the various dimensions of microscopy. In addition, firsthand accounts from researchers utilizing microscopes add depth to the analysis.
"The microscope is a window to the unseen world that is vital for scientific understanding." - Anonymous
Through this structured methodology, the article reveals not only how microscopes function but also their significance in unlocking mysteries of the microscopic universe.
Preamble to Microscopes
Microscopes play a crucial role in the realm of scientific inquiry and education. They are not merely tools for observation; they transform our understanding of the microscopic world. This section aims to establish foundational knowledge and highlight the significant benefits of microscopes in various fields. A clear definition, along with a historical context, provides insight into how this tool has shaped scientific methods and academic curricula.
The narratives surrounding microscopes reveal how they unlock the mysteries of the small. From biology to materials science, they facilitate scientific discovery and promote educational engagement. Through their capabilities, researchers and students can observe phenomena that would otherwise remain invisible. This section sets the stage for a detailed exploration of the multifaceted objectives of microscopes.
Definition of a Microscope
A microscope is an optical instrument designed to magnify small objects and provide visible images of them. Most commonly, it employs lenses to focus light onto a specimen. The basic function of a microscope remains simple: to enhance visibility beyond the limits of the naked eye. Technically, this instrument allows for magnification and resolution, which are key in distinguishing minute details of an object.
Different types of microscopes serve diverse functions. For example, a compound light microscope can magnify objects at very high resolutions, making it ideal for examining cells. Furthermore, an electron microscope utilizes electron beams instead of light, allowing for incredibly detailed imagery at even higher magnifications. By broadening the scope of observation, microscopes enable a deeper understanding of our world at a fundamental level.
Historical Context
The history of microscopy dates back to the late 16th century. The first compound microscope was developed by Zacharias Janssen, a Dutch spectacle maker. His early work laid the groundwork for future advancements in the field. Subsequently, Antonie van Leeuwenhoek, in the 17th century, used simple microscopes to observe bacteria and sperm cells, which further demonstrated the power of the microscope in expanding human knowledge.
During the 19th century, significant improvements were made, particularly with the introduction of achromatic lenses. These lenses reduced chromatic aberration, improving image clarity. Advances in technology continued into the 20th century with the invention of the electron microscope, which revolutionized our ability to examine materials at atomic levels. Throughout history, the microscope has evolved profoundly, embodying the spirit of scientific exploration.
Understanding the evolution of microscopes and their definitions offers a lens through which we can appreciate their importance in both research and education. These instruments continuously serve as essential tools for advancing knowledge across disciplines, underscoring their objective to unveil the complexities of the microscopic realm.
Fundamental Objectives of Microscopes
Microscopes hold a pivotal role in scientific exploration and education. Understanding their fundamental objectives helps one grasp their significance in various disciplines. The essential functions can be categorized into three core areas: enhancing visibility, facilitating discovery, and promoting comprehension.
Enhancing Visibility of Small Objects
One of the primary objectives of a microscope is to enhance the visibility of small objects. Human vision has limitations when it comes to perceiving fine details. Microscopes bridge this gap by magnifying objects, enabling researchers to observe structures that are otherwise invisible to the naked eye.
Microscopes, like the light microscope and electron microscope, employ different techniques to increase detail visibility. Light microscopes utilize lenses and illumination to magnify specimens up to 1000 times their original size. In contrast, electron microscopes achieve higher resolutions, allowing for the observation at the nanometer scale, making them crucial for cellular and molecular biology research.
This ability to magnify minute structures is vital in various fields. For instance, biologists study the cellular architecture, while materials scientists analyze the microstructure of metals and polymers. Enhancing visibility not only aids in research but also in quality control for industries where precision is essential.
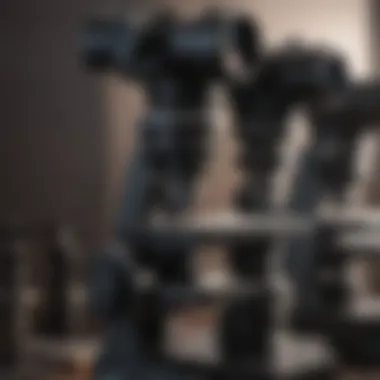
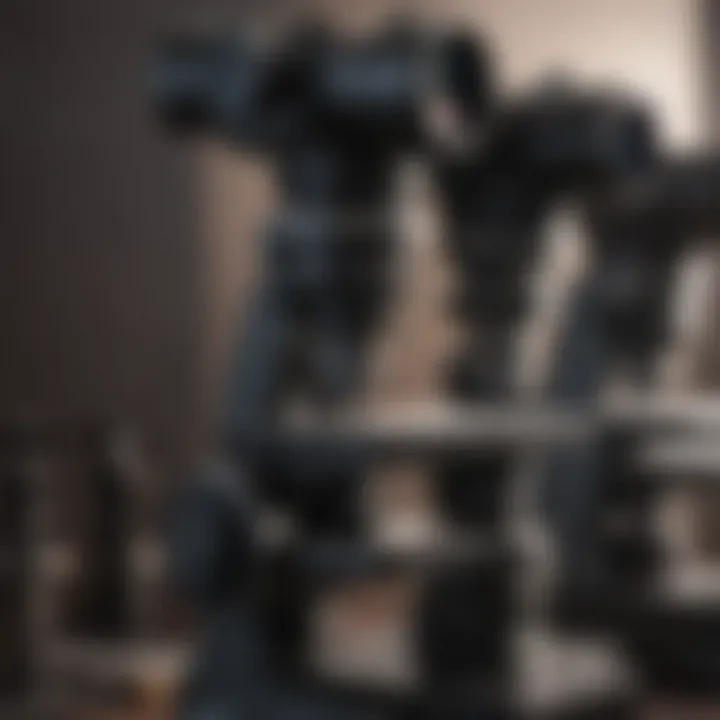
Facilitating Scientific Discovery
Microscopes serve as tools that facilitate scientific discovery by opening new avenues for investigation. They allow researchers to explore previously uncharted territories in science. The insights gained from microscopic analysis lead to breakthroughs in knowledge and understanding.
Through microscopy, scientists have made significant discoveries, such as the identification of microorganisms by Anton van Leeuwenhoek in the 17th century. This discovery laid the groundwork for microbiology. More recently, advancements in fluorescence microscopy have enabled researchers to study processes within live cells, leading to improved understanding in fields like cancer research and drug development.
The ability to visualize interactions at a micro or nanoscale has profound implications in developing novel materials and therapeutic approaches. It encourages innovation and enhances the pursuit of knowledge through various scientific inquiries.
Promoting Educational Understanding
Microscopes also play a fundamental role in education. They are not just instruments for scientists. In schools and universities, they serve as teaching tools that foster a deeper understanding of scientific concepts. The hands-on experience of using microscopes helps students observe biological specimens and mineral structures directly. This interaction enhances learning engagement and curiosity.
Educational curricula frequently incorporate microscope work to illustrate theoretical principles. Students can relate abstract concepts to tangible observations, grounding their knowledge in practical examples. Such experiences encourage critical thinking and inspire future scientific endeavors.
Furthermore, modern educational approaches often integrate digital microscopy, offering more versatile opportunities for learning. Students can analyze images and data through software that enhances their investigative skills.
"The role of a microscope extends beyond mere observation; it is an essential catalyst for discovery and understanding in science."
Types of Microscopes and Their Objectives
The exploration of microscopes can be deepened by understanding the various types that exist, each with their unique objectives and applications. This section discusses the importance of these different types, whether in research or educational contexts. By identifying their specific features and uses, we can appreciate their role in scientific advancements.
Light Microscopes
Light microscopes are fundamental tools in both laboratory settings and classrooms. They utilize visible light to magnify specimens. These microscopes can be classified into two main types: compound light microscopes and dissecting microscopes.
Compound Light Microscopes
Compound light microscopes are notable for their capability to magnify samples using a combination of optical lenses. The primary advantage of these microscopes is their ability to achieve high magnification and resolution of small specimens. This aspect makes them exceptionally valuable in biological research for observing cellular structures.
One key characteristic of compound light microscopes is their multi-lens system, which can provide up to 1000x magnification. This design allows for a detailed examination of thinly sliced specimens, making them widely utilized in educational settings.
A unique feature of compound microscopes is their ability to observe prepared slides. However, they require careful sample preparation, which can be seen as a disadvantage. Sometimes, the technique of preparing samples might result in the loss of information about the specimen's three-dimensional structure.
Dissecting Microscopes
Dissecting microscopes serve a different purpose compared to their compound counterparts. They provide lower magnification but allow users to see the surface details of larger specimens, making them ideal for dissection and manipulation.
The key characteristic of dissecting microscopes lies in their use of stereoscopic vision. This capability gives users a three-dimensional view of the sample. It is a popular choice for educational applications, especially in biology laboratories.
A unique feature is the ability to work with larger or thicker samples without the need for extensive preparation. However, their lower magnification limits the detailed observation of cells or finer structures, which can be seen as their main disadvantage.
Electron Microscopes
Electron microscopes represent a significant evolution in microscopy, using electron beams instead of light to achieve much higher magnifications. They include Transmission Electron Microscopes and Scanning Electron Microscopes.
Transmission Electron Microscopes
Transmission Electron Microscopes (TEM) are designed to transmit electrons through a specimen. This type offers high resolution that allows visualization of internal cellular structures at the molecular level.
The primary characteristic of TEM is its ability to produce images that can show details at resolutions down to a few nanometers. This is beneficial in fields like virology and materials science, where detailed internal structures matter.
A notable feature is that TEM requires very thin samples. This necessity might present preparation difficulties, highlighting a significant disadvantage; thicker samples cannot be adequately analyzed through this method.
Scanning Electron Microscopes
Scanning Electron Microscopes (SEM) function differently by scanning a focused electron beam across the surface of a specimen. They generate detailed three-dimensional images of the surface topology.
One key characteristic of SEM is its high depth of field, which provides a comprehensive view of the specimen's surface features. This microscope is important for applications in materials science and electronics.
A unique feature is the ability to analyze larger samples without extensive preparation. Nevertheless, sample fixation and coating are usually required, which might limit the analysis of certain biological specimens.
Other Specialized Microscopes
The field of microscopy extends into other specialized types that cater to specific research needs. Three notable examples are Fluorescence Microscopes and Confocal Microscopes.
Fluorescence Microscopes
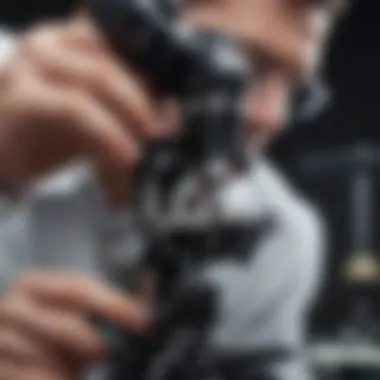
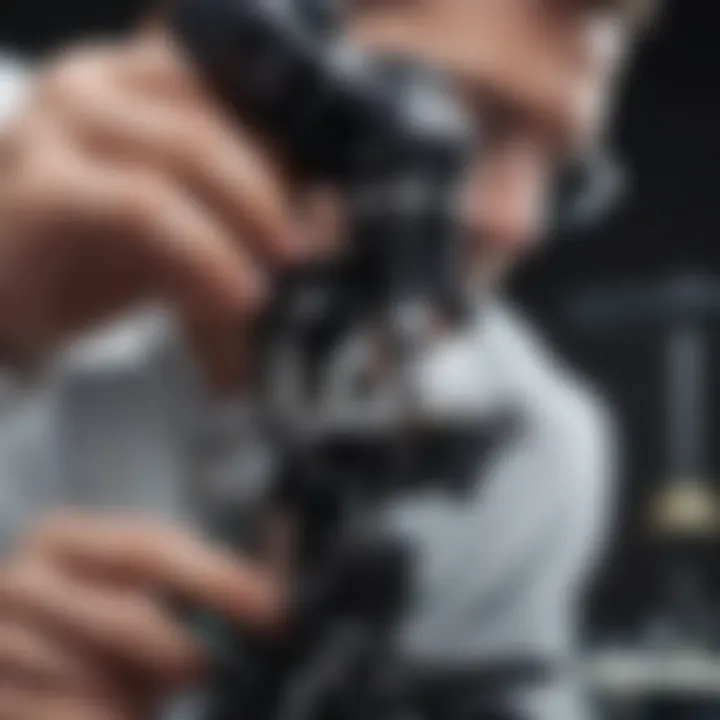
Fluorescence Microscopes are essential in biological research, allowing for the visualization of fluorescently labeled samples. Their ability to detect specific biological markers contributes greatly to cellular biology and pathology.
The key characteristic of these microscopes lies in their use of fluorescence to illuminate the specimen. This allows researchers to study specific cell components or proteins in great detail.
A unique feature of fluorescence microscopes is their capability to conduct multiplex studies, where several markers are observed simultaneously. However, a drawback is that autofluorescence from samples can interfere with results, potentially complicating image interpretation.
Confocal Microscopes
Confocal Microscopes represent an advanced form of optical microscopy that provides high-resolution images with improved contrast. They achieve this by using a pinhole to eliminate out-of-focus light.
One significant characteristic is their ability to create three-dimensional reconstructions of a specimen by taking multiple two-dimensional slices. This feature is beneficial in studying complex structures within cells.
A unique aspect of confocal microscopy is its increased imaging speed. This rapid imaging can be a crucial advantage during time-lapse studies. However, the complexity of the setup and higher costs can limit accessibility for some research environments.
Principles of Microscopy
The principles of microscopy are fundamental to understanding how microscopes function and what objectives they serve in scientific research and education. These principles not only define the limitations and capabilities of different microscope types but also enhance our comprehension of how they contribute to advancements in various fields. In this section, we will delve into optical principles and electron wave interferenceโtwo critical components that underpin the operation of microscopes.
Optical Principles
Optical principles revolve around the behavior of light when interacting with objects. Light microscopes rely on these principles to magnify small specimens. Key elements include refraction, reflection, and diffraction, which manipulate light to produce an enlarged image of the sample. The quality of the optical elementsโsuch as lensesโaffects clarity and resolution.
- Refraction: This is the bending of light as it passes through different mediums. Lenses are designed to exploit refraction to converge light rays, producing a magnified image.
- Resolution: Defined as the ability to distinguish between two points, resolution is crucial for clarity. It is influenced by the wavelength of light and the numerical aperture of the lens.
- Contrast: To make certain features visible, contrast must be enhanced. Techniques such as staining can improve the visibility of transparent specimens.
Optical principles provide the groundwork for various types of light microscopy, highlighting their role in biological and educational settings. The understanding of how light works allows researchers to adapt microscopy techniques for specific applications, leading to improved image quality and accurate results.
Electron Wave Interference
The principles of electron wave interference play a crucial role in electron microscopy, which allows scientists to observe materials at nanometer scales. Unlike light, electrons exhibit wave-like properties, leading to a different approach in imaging. This principle stems from quantum mechanics, where electrons are treated as waves that can interfere with one another.
When electrons are emitted from a source, they travel through the microscopeโs column, encountering electromagnetic fields that focus and direct their paths. Interference occurs when these electron waves overlap, enhancing certain structures and reducing noise, ultimately improving image clarity. Some key points to note:
- Wavelength: The shorter wavelength of electrons compared to visible light results in higher resolution capabilities, making it possible to view structures at the atomic level.
- Phase Contrast: Similar to light microscopy, phase contrast techniques can be applied to electron waves, improving image details without damaging delicate specimens.
- Sample Preparation: Special techniques are required for preparing samples, as they need to be very thin for electrons to pass effectively, which presents unique challenges compared to light microscopy.
Understanding electron wave interference is essential for future innovations in microscopy, as it paves the way for discovering new materials and biological mechanisms. Thus, mastering these principles enhances the scope of research possibilities in science.
"The understanding of microscopy principles not only expands the horizons of what can be seen but also enriches our approach to scientific inquiry."
By grasping these principles, researchers and students can begin to appreciate the powerful tools at their disposal and how they can be employed to further knowledge across diverse scientific disciplines.
Technological Advancements in Microscopy
Technological advancements in microscopy have significantly transformed the landscape of scientific inquiry. The continuous evolution of this tool has enhanced its precision, efficiency, and scope of application. These innovations are vital for researchers and educators alike, allowing for deeper insights into microscopic worlds that were previously inaccessible. The benefits are manifold, ranging from increased resolution and digital capabilities to the integration of advanced imaging techniques.
Improvement in microscopy technology not only facilitates more detailed observation but has also widened the scope of disciplines that rely on microscopes. In the modern age, the demand for accurate data has propelled the development of various advanced techniques, each building upon the findings and limitations of their predecessors.
Digital Microscopy
Digital microscopy represents a significant leap forward in the field. Unlike traditional methods that rely on optical lenses, digital microscopy utilizes sensors to capture images. This means that images can be processed and analyzed using software, which allows for precise measurements and sophisticated image manipulations. The ability to record high-definition images digitally ensures that data can be shared easily within research communities.
One of the central advantages of digital microscopy is its capacity for real-time imaging. This facilitates dynamic observation of specimens, an advantage notable in fields such as biology and material science where time-sensitive changes are critical. Researchers can easily document their findings and compare results without compromising the integrity of the sample.
3D Imaging in Microscopy
3D imaging in microscopy is another revolutionary advancement that offers a comprehensive view of specimens. This technique enables the reconstruction of three-dimensional images from two-dimensional data collected by the microscope. As a result, researchers gain essential insights into the spatial organization and relationships among different components of a sample.
By using techniques like optical sectioning or confocal microscopy, scientists can delve deeper into the complexities of structures at a micro scale. This is especially crucial in biological sciences, where understanding the morphology and spatial distribution of cells and tissues is vital for the study of health and disease.
In summary, technological advancements in microscopy, including digital and 3D imaging techniques, not only enhance the effectiveness of the microscope but also expand its potential applications across various scientific domains.
Applications of Microscopes in Scientific Disciplines
The application of microscopes across various scientific fields represents a cornerstone of modern inquiry and understanding. Their ability to magnify and analyze minute details transforms our comprehension of countless subjects. The significance lies in how microscopes facilitate concrete discoveries, enable effective study, and advance the boundaries of knowledge. Through careful examination, it becomes clear that microscopy is not limited to a singular function; its versatility enhances research and education.
Biological Research
Microscopy plays an essential role in biological research. Biologists utilize microscopes to examine cellular structures, observe organizational patterns, and discern interactions among microscopic organisms. Light microscopes allow for a detailed study of living cells, capturing real-time processes like mitosis and movement. Every observation leads to insights about cellular functions and potential abnormalities that may indicate disease.
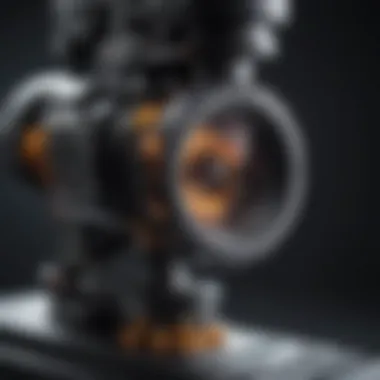
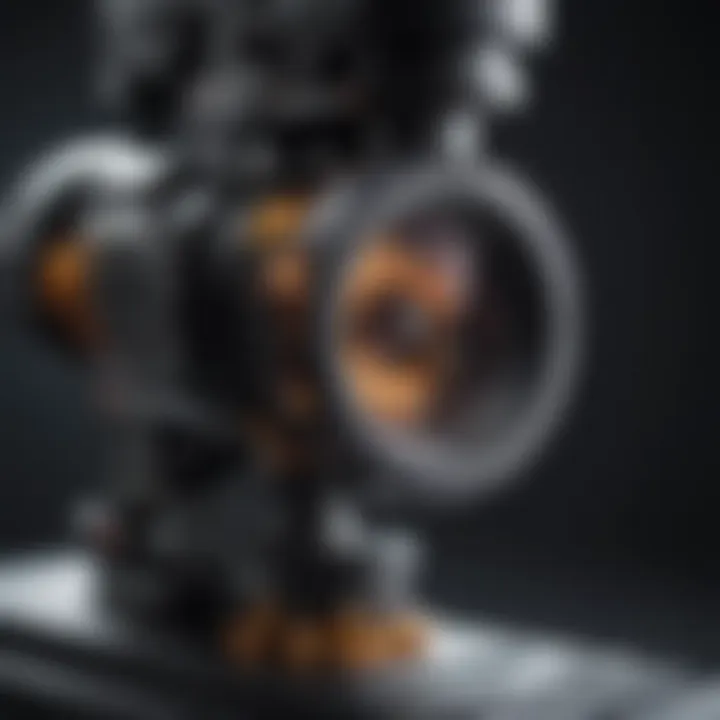
Electron microscopes, particularly transmission and scanning types, offer even higher resolution. They reveal intricate details about cellular components, such as organelles, viruses, and other nano-sized structures. This level of knowledge is critical in medical research, where understanding pathology can directly influence treatment methodologies. The insights gained from microscopic analysis pave the way for innovations in fields such as genetics, virology, and biotechnology.
Material Science
In material science, microscopes serve as pivotal tools for characterizing the physical and chemical properties of materials. Researchers rely on various types of microscopes to investigate metal alloys, polymers, ceramics, and nanomaterials. The ability to visualize microstructural features aids in understanding materials' behaviors, mechanical properties, and potential failures.
Scanning electron microscopy (SEM) is especially beneficial here. It provides detailed images of the surface structure and composition of materials. Such knowledge supports the development of stronger, more adaptable materials, thus advancing industries like construction, aerospace, and electronics. Comprehending how materials behave at a micro level ensures safer, more efficient designs and applications, ultimately benefiting society at large.
Nanotechnology
The field of nanotechnology heavily relies on microscopy for both research and application purposes. At the nanoscale, traditional methods of analysis often fall short. Microscopy enables researchers to manipulate materials on an atomic or molecular level, leading to discoveries that would otherwise remain elusive. Techniques such as atomic force microscopy (AFM) allow scientists to engage with nanostructures directly, examining their properties, interactions, and functionalities.
Moreover, the development of new nanomaterials has implications across many sectors, including medicine, electronics, and environmental science. These advancements highlight the importance of microscopy in ensuring quality and performance in the final products. Insights derived from nanoscale observations lead to innovative solutions for complex challenges, demonstrating the profound impact of microscopes in extending the frontiers of science.
"Microscopes are not just instruments but gateways to unseen dimensions of knowledge. Through them, we witness the intricacies of life and matter, thus deepening our understanding of the universe around us."
Limitations of Microscopy
Understanding the limitations of microscopy is critical when evaluating its effectiveness and applicability in various scientific fields. While microscopes play an essential role in advancing our knowledge, recognizing their constraints helps researchers to make informed decisions about their use. Limitations can arise from several factors, which can affect both the quality of the images produced and the interpretability of the data obtained.
Resolution Limits
One of the most significant limitations in microscopy is the issue of resolution. Resolution refers to the microscope's ability to distinguish between two close points as separate entities. In optical microscopes, the resolution is fundamentally limited by the wavelength of light. Typical microscopes may not resolve objects closer than about 200 nanometers, a restriction imposed by the diffraction of light. This limitation can obscure small structures like organelles in cells, thereby hindering a detailed understanding of biological processes.
Modern techniques such as super-resolution microscopy have emerged, focusing on overcoming these limits. For instance, STED (Stimulated Emission Depletion) microscopy can achieve resolutions down to 20 nanometers. However, these advanced methods usually come with increased costs and complexities, which may not be feasible in all laboratory settings.
Sample Preparation Challenges
Another critical limitation stems from the challenges associated with sample preparation. Preparing a specimen for microscopic examination can introduce artifacts, which may distort the observed structure. These artifacts can arise from fixation methods, staining procedures, or even slicing samples thinly enough for light to pass through.
Additionally, some materials might not be compatible with certain types of microscopy. For example, biological samples are often gold-coated for electron microscopy, but this alteration might change their natural appearance or chemical properties. Furthermore, there is the question of viability. Biological specimens can be rendered non-viable during preparation, which may limit the types of studies that can be performed.
Ultimately, while microscopy remains a powerful tool, its effectiveness is mitigated by resolution limits and preparation challenges. A nuanced understanding of these limitations allows researchers to interpret their findings with greater accuracy and to seek alternative methods when necessary.
Understanding the limitations of microscopy is essential to make informed decisions about how to collect and interpret data effectively.
With ongoing advancements in technology and methodologies, the challenge of overcoming these limitations continues to be a vital area for research, ensuring that microscopy remains an integral part of scientific inquiry.
Future Directions in Microscopy Research
Research in microscopy continues to evolve, driven by the demands of scientific inquiry and technological advancements. Future directions in this field hold significance for enhancing the capabilities of microscopes, particularly in terms of resolution, speed, and the ability to visualize dynamic processes in real time. As scientists explore innovative methodologies, they unlock new potential for discoveries across various disciplines, from biology to material science.
Potential Innovations
Future microscopy innovations aim to redefine how we observe and analyze samples. A few areas of focus include:
- Super-resolution Techniques: Methods like STED (Stimulated Emission Depletion) and PALM (Photo-Activated Localization Microscopy) are being refined. They exceed traditional optical limits, allowing for imaging at the nanoscale. Such innovation is vital for understanding intricate cellular structures.
- Live Cell Imaging: Advancements in fluorescent probes and imaging software will enable researchers to observe live cells in their natural environments. This will allow for a better understanding of cellular processes, which is crucial for therapeutic developments.
- Machine Learning Algorithms: Integrating artificial intelligence in image analysis can significantly enhance automation in data interpretation. This can lead to quicker analyses and increased accuracy in identifying subtle variations in samples.
- Hybrid Microscopy Techniques: Combining techniques, such as light and electron microscopy, can provide complementary data. Such approaches can elucidate both the structural and functional aspects of specimens.
Integration with Other Technologies
The integration of microscopy with other emerging technologies promises to broaden the scope of research and application. Key integrations include:
- Nanotechnology: The use of nanoparticles in microscopy can improve contrast and resolution. For example, gold nanoparticles enhance imaging capabilities, which is essential in fields like drug delivery and diagnostics.
- 3D Printing: Customization of microscopy components, like lenses or stages, through 3D printing can tailor tools to specific research needs. This accessibility could democratize advanced microscopy techniques for laboratories worldwide.
- Data Sharing Platforms: Leveraging cloud technology allows researchers to collaborate across geographical boundaries. Platforms can store and share microscopy data efficiently, facilitating global cooperation in scientific research.
"Future directions in microscopy research are pivotal for scientific progress, enabling us to visualize what was once unseen."
- Biotechnology: The integration of microscopy with biotechnological innovations can enhance the study of genetic materials. Techniques like CRISPR can be visualized within cellular environments, offering insights into gene function and regulation.
Continuous exploration in these areas promises not only increased understanding but also opens new avenues for technological applications, impacting various fields of research and education.
Culmination
The conclusion of this article encapsulates the critical discussions surrounding the objectives of microscopes. Microscopes serve as fundamental instruments in many fields, especially in scientific research and education. The understanding of their multifaceted goals can lead to a deeper appreciation of their functions and potential.
Summation of Objectives
Microscopes enhance our ability to observe details that are invisible to the human eye. The range of objectives can be summarized as follows:
- Visibility Improvement: Microscopes magnify small objects, revealing intricate structures. This capability is essential in disciplines like biology, where cellular details can be crucial for understanding life processes.
- Scientific Exploration: They are vital in facilitating discoveries, allowing researchers to explore materials and biological specimens in depth. The advancement of scientific knowledge often relies on the insights gained from microscopic observations.
- Educational Value: Microscopes serve as learning tools, enabling students and educators to engage with scientific principles interactively. Visualizing microscopic specimens makes abstract concepts more tangible, fostering a deeper understanding of science.
Microscopes as Tools for Advancement
Microscopes are more than just instruments; they are gateways to scientific advancement. Their role includes:
- Research Development: By providing a closer look at materials, microscopes enable innovations in various fields, including materials science and nanotechnology. Understanding the microstructure of materials can lead to the development of stronger and more efficient substances.
- Interdisciplinary Applications: The use of microscopes transcends traditional boundaries, connecting biology, chemistry, and physics. This interdisciplinary approach fosters a collaborative environment for research, driving innovation.
- Future Innovations: Emerging technologies in microscopy, such as Digital Microscopy and 3D Imaging, hold promise for even greater advancements. As these technologies develop, they may redefine how microscopic imaging is utilized in research and education.