Crispr-Cas9: A New Era in Genetic Engineering
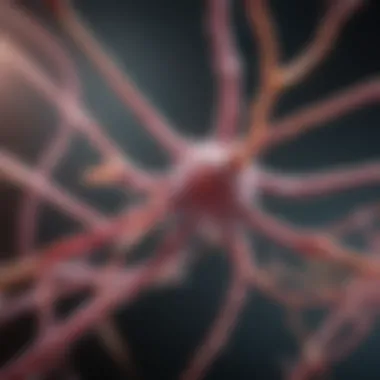
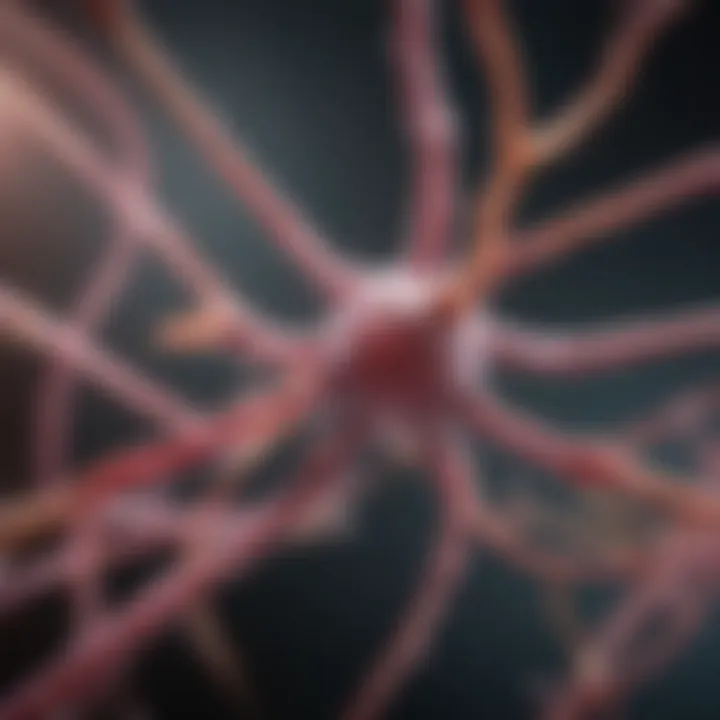
Intro
Crispr-Cas9 emerges as a pivotal breakthrough in genetic engineering, offering unprecedented precision in genetic modifications. This technology, which builds on the natural defense mechanisms of certain bacteria, allows for targeted alterations in DNA. It is more than just a scientific marvel; it carries implications across various fields including medicine, agriculture, and biotechnology.
Understanding this technology requires exploring its origins and mechanisms. The Crispr system works by utilizing guide RNA to direct the Cas9 enzyme to specific DNA sequences. Once there, it can cut the DNA, allowing scientists to add or remove genes effectively. This has made Crispr-Cas9 a vital tool in research labs worldwide.
There are many applications worth mentioning, from developing treatments for genetic disorders to engineering crops that can withstand pests or climate challenges. However, its transformative potential is overshadowed by ethical dilemmas. Questions regarding genetic privacy, ecological risks, and possible misuse of the technology necessitate careful consideration as the field advances.
The subsequent sections will provide a detailed review of the research landscape, including its findings and objectives, followed by a discussion of the methodology used in studies related to Crispr-Cas9.
One must recognize that while Crispr technology holds great promise, it also demands responsible stewardship. Proper discourse will set the stage for innovations that are not only effective but also ethically sound.
Preamble to Crispr-Cas9
Understanding Crispr-Cas9 is pivotal for grasping the current landscape of genetic engineering. This advanced technology stands out due to its efficiency in altering DNA. Researchers and professionals are increasingly drawn to its applications across various domains, from medicine to agriculture. The ability to precisely edit genes has opened new avenues for innovation. However, with great power comes significant responsibility. Exploring the implications and operational mechanics of Crispr-Cas9 will shed light on both its promise and the challenges it presents.
Historical Context
The journey of Crispr-Cas9 began long before it reached laboratories and clinics. The roots trace back to the early 1980s, when scientists first stumbled upon repetitive DNA sequences in E. coli. Initially, their function remained a mystery. It was in 2000 that researchers recognized these sequences as part of a bacterial immune system, protecting against viral infections. This discovery paved the way for harnessing Crispr technology in a scientific context. Subsequent studies illuminated how these sequences work in conjunction with Cas proteins, particularly the Cas9 enzyme, culminating in a powerful tool for gene editing.
Discovery of Crispr Sequences
The identification of Crispr sequences marked a significant breakthrough. These unique patterns consist of short segments of DNA repeated in a specific order. Known as "clustered regularly interspaced short palindromic repeats," Cristprs were found interspersed within bacterial genomes. In 2007, a pivotal study revealed that bacterial cells utilize these sequences to remember viruses they have encountered. Each time a virus attacks, the bacterial immune system stores part of the viral DNA. This discovery not only unveiled a natural defense mechanism but also provided a framework for developing gene editing technologies. By 2012, the first applications of Crispr-Cas9 in targeted gene modification were reported, demonstrating its potential in a wide array of fields. This trajectory marks the beginning of a new era in genetic manipulation.
The Mechanisms of Crispr-Cas9
Understanding the mechanisms behind Crispr-Cas9 is essential to grasping its role in genetic engineering. The potential of this tool lies in its ability to precisely edit DNA sequences, offering revolutionary approaches in various fields. The mechanisms involve different components that work in concert and a well-defined process that enables gene editing. Each of these elements not only contributes to the overall effectiveness of Crispr-Cas9 but also sheds light on the practical applications and ethical considerations surrounding its use.
Components of the Crispr System
Guide RNA
Guide RNA is a crucial component in the Crispr-Cas9 system. It serves as a template that directs the Crispr-associated protein 9 to the specific location in the DNA that needs to be modified. The key characteristic of guide RNA is its designed sequence, which can be tailored to match any target DNA sequence. This flexibility makes guide RNA a beneficial choice in many applications.
A unique feature of guide RNA is its adaptability. Researchers can create custom guide RNAs for various genes, allowing for precise targeting of specific sequences. This ability is particularly advantageous in medical research, where targeting genes associated with disorders can lead to effective therapies. However, the challenge lies in ensuring the accuracy of the guide RNA so that it only binds to the intended target without affecting other critical genes, which may cause undesirable outcomes.
Crispr-associated protein
Crispr-associated protein 9 (Cas9) is another vital element in the Crispr system. This protein acts as the molecular scissors that cut the DNA strands at the specified location. The key characteristic of Cas9 is its precision in generating double-strand breaks in the target DNA, allowing for the subsequent insertion or deletion of genetic material. This precision solidifies its status as a preferred tool for genetic editing.
One unique feature of Cas9 is its ability to function in a range of organisms, from bacteria to plants to animals, which has broad implications for biotechnological applications. However, over time, there's growing concern about potential off-target effects. It is vital to fine-tune the delivery of Cas9 to minimize these risks, reinforcing the ongoing quest for improved precision in genetic engineering.
The Process of Gene Editing
Targeting DNA
Targeting DNA is the first essential step in the gene-editing process with Crispr-Cas9. This involves locating the specific sequence that researchers want to edit. The key characteristic of this step is the crucial role that guide RNA plays in identifying the target site accurately. It is a beneficial process since it precedes the actual cutting of the DNA, ensuring that the interventions are made where they are intended.
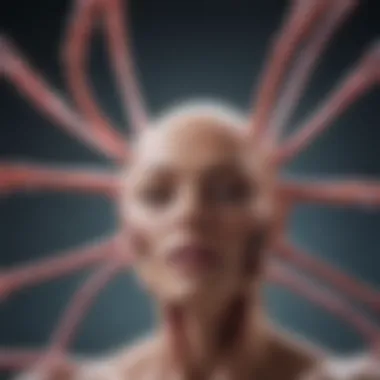
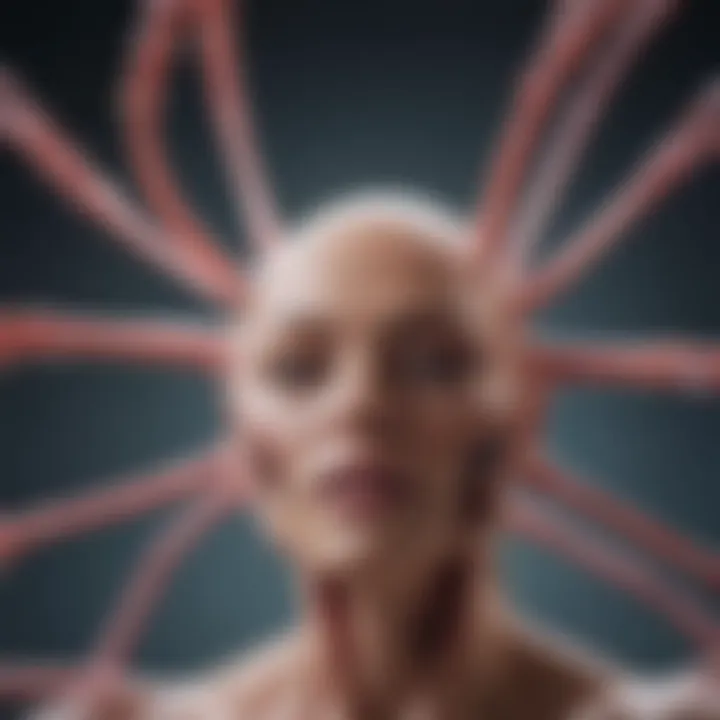
The unique feature of target DNA identification is the sequence specificity tied to the guide RNA. When a precise match is found, this enables researchers to minimize unintended modifications outside of the target area. However, without careful design, there remain risks regarding misalignment, which could lead to incorrect edits.
Cutting the DNA
Cutting the DNA is the subsequent action that follows targeting. Cas9 performs this task by creating a double-strand break at the specified location. The key characteristic of this cutting process is its efficiency, often occurring in a single operation. This affects the overall speed and effectiveness of genetic editing, making it a popular choice among scientists.
The unique feature of this cutting mechanism is its reliance on precise guide RNA. Well-designed guide RNA can lead to clean cuts without affecting nearby genetic material. The challenge here, however, lies in ensuring that cuts are always accurately made. Any off-target action can lead to unwanted genetic changes, which necessitates thorough verification by researchers.
Repair Mechanisms
Repair mechanisms refer to the cellular processes that follow the DNA cut made by Cas9. After the DNA is cut, cells will attempt to repair the break. The key characteristic of these mechanisms is that they can be harnessed for gene editing. Depending on how the cell repairs the break, scientists can either inactivate a gene or insert new genetic material. This versatility makes it a beneficial aspect of gene editing.
One unique feature of repair mechanisms is the use of donor DNA templates, which guide the incorporation of new genetic sequences during the repair process. However, this also poses risks. If the donor template is not properly designed, it can lead to random insertions or deletions, resulting in unpredictable genetic alterations. Thus, understanding these repair mechanisms is critical for effective editing and minimizing unintended consequences.
Applications of Crispr-Cas9
The applications of Crispr-Cas9 are vast and varied, affecting multiple fields including medicine, agriculture, and biotechnology. The significance of these applications lies not only in their innovative approaches but also in the profound implications they hold for the future of genetic research. Crispr-Cas9 opens new possibilities for precise genetic modifications which could lead to breakthroughs in treating diseases, improving food security, and advancing industrial processes. Both the benefits and ethical considerations surrounding these applications warrant significant attention.
Medical Applications
Gene Therapy
Gene therapy represents one of the most promising applications of Crispr-Cas9 technology. This technique allows for the direct alteration of genes in a person's cells to treat a disease or disorder. The key characteristic of gene therapy is its ability to target specific genetic mutations at their source. This offers a potentially life-changing alternative for patients with previously untreatable genetic conditions.
A unique feature of gene therapy is its ability to introduce corrected genes into a patient's genome, enhancing or replacing malfunctioning genes. While this approach presents significant advantages, such as the potential for permanent cures, it also faces challenges. Concerns include the risk of unintended consequences or off-target effects, where the gene editing may inadvertently alter unintended areas of the genome. The balance between potential benefits and these risks continues to drive research and discussion in the field.
Targeting Genetic Disorders
Targeting genetic disorders through Crispr-Cas9 allows researchers to focus on specific diseases caused by genetic anomalies, such as sickle cell disease or cystic fibrosis. This aspect contributes to the overall goal of reducing the prevalence of inherited conditions, which impact millions worldwide. The prominent feature of this application is its tailored approach, enabling precise modifications that can significantly improve the quality of life for those affected.
The unique attribute of targeting these disorders lies in the personalized treatment possibilities it offers. For example, patients may receive therapies that are custom-designed to correct their specific genetic mutations. Nevertheless, ethical dilemmas and long-term implications of such therapies need to be addressed fully. The discussion around these implications emphasizes the dual-edged nature of technological advances in genetic engineering.
Agricultural Innovations
Crop Improvement
Crop improvement using Crispr-Cas9 focuses on enhancing the yield and resilience of staple crops to meet global food demands. This approach can lead to the development of crops that are more resistant to climate change, pests, and diseases. The key characteristic of this application is its ability to produce rapid changes in crop genetics without the introduction of foreign DNA, which addresses some consumer concerns around genetically modified organisms (GMOs).
A distinguishing feature of crop improvement involves the efficiency with which Crispr-Cas9 can create desired traits, such as drought resistance or improved nutritional content. While this holds immense potential for food security, it also raises questions about biodiversity and the long-term effects of uniform genetic modifications across agricultural systems. The dialogue on sustainability versus improvement remains crucial in ongoing studies.
Pest Resistance
Pest resistance mechanisms developed through Crispr-Cas9 are designed to reduce reliance on chemical pesticides, which have harmful effects on ecosystems. This aspect is vital as it contributes to more environmentally sustainable agricultural practices. The primary characteristic of this application is its targeted nature, enabling specific genetic pathways in plants that deter pest attacks.
The unique feature of pest resistance cultivated through genetic editing is the potential reduction in crop losses. This can lead to increased food availability and reduced costs for farmers. However, the long-term implications include the possibility of pests evolving resistance over time, thus necessitating an adaptive strategy for pest management. The balance between immediate benefits and long-term agricultural sustainability must be thoughtfully considered.
Biotechnology Developments
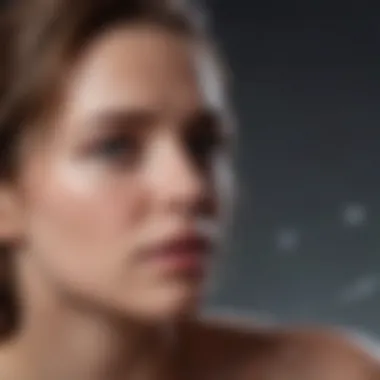
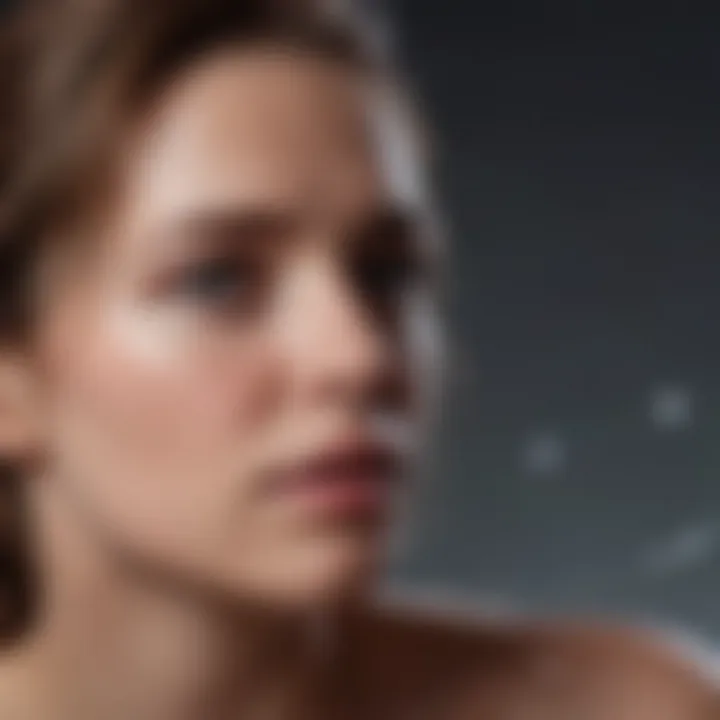
Production of Biofuels
In the realm of biotechnology, the production of biofuels using Crispr-Cas9 technology emerges as a compelling application. This method targets specific genes in organisms like algae or bacteria to enhance their efficiency in biofuel production. The key characteristic here is its potential to create more sustainable energy sources, addressing the growing need for renewable energy.
A unique feature of biofuel production through Crispr-Cas9 is its ability to optimize the natural processes of organisms to yield higher energy outputs. This presents advantages in reducing greenhouse gas emissions compared to fossil fuels. However, challenges such as scalability and economic feasibility are significant hurdles that still need addressing for wider implementation.
Industrial Biotechnology
Industrial biotechnology leverages Crispr-Cas9 to improve the production processes of various products, ranging from enzymes to bioplastics. This application focuses on enhancing the efficiency and sustainability of these processes. The key characteristic is the customization of microbial strains to produce desired outputs more effectively than traditional methods.
The unique feature of industrial biotechnology applications is the potential for significant cost reductions and efficiency gains in producing chemicals and materials. This can lead to a more sustainable approach to manufacturing and resource consumption. Nevertheless, the long-term impacts on microbial biodiversity and ecosystem balance are concerns that require careful monitoring.
The impact of Crispr-Cas9 technology is reshaping the landscape of multiple fields, guiding both researchers and industries towards greater efficiency and sustainability.
Ethical Considerations in Crispr-Cas9 Research
The advent of Crispr-Cas9 technology has raised significant discussions surrounding ethical considerations in genetic engineering. The ability to edit DNA with precision brings potential benefits but also risks that cannot be overlooked. It is crucial to explore these ethical dimensions to ensure responsible usage and guide future research. This section highlight the importance of addressing these considerations, encompassing the ethical frameworks applied and the public perception surrounding this powerful tool.
Ethical Frameworks
Bioethics Overview
Bioethics serves as a foundational aspect of modern medical and scientific inquiry, providing principles to navigate moral dilemmas. The field of bioethics emphasizes respect for individuals, their autonomy, and the societal implications of scientific advancements. One key characteristic of bioethics is its interdisciplinary nature, pulling insights from philosophy, law, and social science, enhancing the overall understanding of ethical dilemmas.
The importance of bioethics in the context of Crispr-Cas9 lies in its role in establishing guidelines for research and application. This can effective prevent misuse and ensure that scientific inquiries Aligns with the values of society. The unique feature of bioethics is its adaptability to emerging technologies, which is particularly relevant in the case of genome editing. However, there are disadvantages, such as potential inconsistencies in ethical standards among different cultural or legal frameworks.
Ethical Dilemmas in Editing Germline Cells
Editing germline cells involves altering the genetic material passed on to future generations, raising profound ethical dilemmas. The key characteristic of these dilemmas centers around altering human inheritance and its long-term impact on the gene pool. This aspect is highly relevant to this article, as it exposes tensions between scientific progress and ethical responsibility.
One of the unique features of addressing these dilemmas is the risk of creating social inequalities based on genetic modifications. While the potential benefits of curing genetic disorders are significant, they may also lead to new forms of discrimination or enhancement that exacerbate existing disparities. Thus, this topic reflects the dual-edged nature of Crispr-Cas9 technology, demanding careful consideration and robust discussions among scientists, ethicists, and policymakers.
Public Perception
Public perception shapes the direction of scientific research, and Crispr-Cas9 is no exception. Much of the public concerns arise from both understanding and skepticism about genetic modifications. On one hand, there is excitement regarding the potential breakthroughs in medicine and crop sciences. On the other hand, some individuals express apprehension about the unintended consequences of manipulating the genetic code.
Education plays a vital role in bridging this gap. Enhancing public knowledge helps mitigate fears and encourages informed discussions. Open dialogues between scientists and the general public are necessary to demystify Crispr-Cas9 technology, illuminating its possibilities and addressing concerns.
Crispr-Cas9 technology presents both opportunity and risk. Ethical considerations in its application must be a priority. Only by ensuring that diverse perspectives are considered can scientific advancements ensure responsible and equitable outcomes.
Future Directions for Crispr-Cas9 Technology
The evolution of Crispr-Cas9 technology has far-reaching implications for genetic engineering and related fields. As research progresses, several avenues are opening up for future exploration. The continued development of this system holds the promise of enhanced applications in medicine, agriculture, and beyond. Key areas of focus will be innovations in precision and the reduction of off-target effects, along with the potential for new discoveries.
Innovations and Improvements
Enhancements in Precision
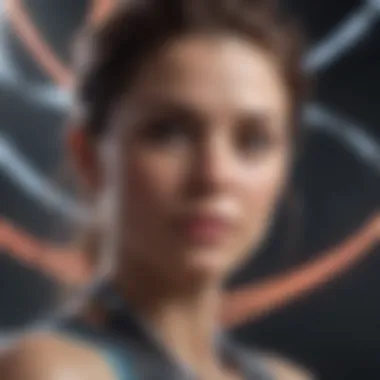
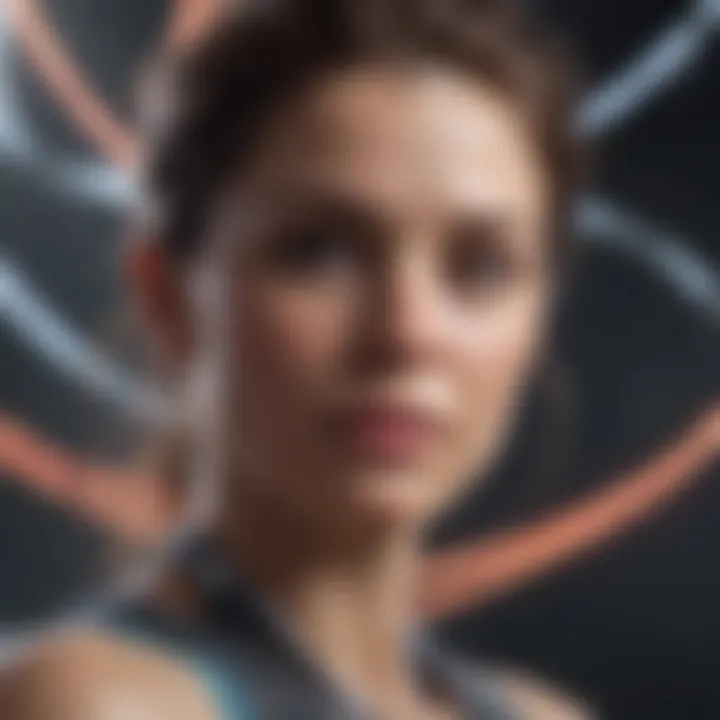
Enhancing precision in Crispr-Cas9 is critical for successful applications. Precision refers to the accuracy with which the technology targets specific genes, minimizing unintended edits. With improved precision, research benefits because it reduces the likelihood of off-target modifications that can lead to unforeseen consequences. The goal here is to create systems that can effectively target complex genetic sequences without unintended collateral damage. This enhancement is essential for therapeutic applications, especially in gene therapy, where specificity can determine success.
A key characteristic of this precision enhancement is the development of high-fidelity variants of Cas9 enzymes. These variants are designed to recognize and bind to specific DNA sequences with greater accuracy, making them a favored choice among researchers. As scientists rethink and optimize the Crispr technology, they prioritize improving tools that would contribute more accurately to genetic editing efforts.
However, challenges remain. While increased precision may limit unintended effects, it might also slow down the editing process. Researchers need to balance speed and efficiency with accuracy.
Reducing Off-Target Effects
Reducing off-target effects is another major focus for the future of Crispr technology. These unintended edits can have serious implications, especially in clinical applications where safety is paramount. Reducing these effects involves refining the design of guide RNAs to improve targeting capabilities. When errors occur, they can alter genetic function unpredictably, leading to complications.
The key characteristic of reducing off-target effects is the adaptation of testing methods. These methods allow researchers to assess the accuracy of their targeting strategies comprehensively. This is vital in establishing the safety and reliability of Crispr-Cas9 applications.
As with precision enhancement, minimizing off-target effects also has advantages and disadvantages. Fewer unintended changes promote safer research, particularly in medical applications. However, the intricate design required to achieve such specificity might complicate the application process, possibly making it more labor-intensive than simpler alternatives.
Potential for New Discoveries
Exploring New Applications
The exploration of new applications for Crispr-Cas9 is vital for maximizing its utility. Researchers are continually looking to apply this technology outside traditional fields. The flexibility of Crispr-Cas9 allows it to potentially impact areas like environmental science, where it might be used to engineer organisms capable of degrading pollutants.
A highlighted feature of exploring new applications is the ability to target novel cellular pathways that were previously difficult to manipulate. This opens up possibilities for innovations not previously conceived. The exploration could yield numerous benefits, including the correction of genetic defects in species that are difficult to edit using standard procedures.
Despite its potential, researchers face challenges in validating these methods across diverse organisms. Each application may require unique considerations, which can complicate the research and development process.
Integrating Crispr with Other Technologies
Integrating Crispr with other technologies represents an exciting frontier. Combining Crispr-Cas9 with systems like synthetic biology or artificial intelligence may unlock new levels of genetic engineering. For instance, AI technologies can help predict the outcomes of genetic edits more reliably, leading to better-targeted interventions.
A distinctive aspect of such integration is its ability to augment existing capabilities. For example, integrating Crispr with advanced gene sequencing technologies can provide unprecedented insights into genetic landscapes. By understanding the genome better, researchers can achieve remarkable precision when applying Crispr systems.
While this integration promises great benefits, challenges persist. Research in this area can become highly complex, requiring interdisciplinary collaboration to develop effective systems. As technology evolves, achieving seamless integration will demand ongoing attention.
The future of Crispr-Cas9 technology is bright, combining enhancements in precision with exciting opportunities for new discoveries. As this field grows, researchers continue to aim for safer and more effective applications in various sectors.
Ending
The conclusion serves as a pivotal component in understanding the complex landscape of Crispr-Cas9 technology. Here, it synthesizes the various themes discussed throughout this article and reflects on the importance of genetic engineering advancements.
One vital element to underline is the significant impact of Crispr-Cas9 across multiple domains, notably in medicine, agriculture, and biotechnology. The precision and efficiency of this technology enable remarkable advancements in gene therapy, pest-resistant crops, and the sustainable production of biofuels, among other applications. Furthermore, the ethical considerations previously outlined, particularly relating to germline editing, emphasize the dual-edged nature of scientific progress. Researchers must navigate these ethical waters carefully, balancing innovation with responsibility.
Additionally, the broader scientific context situates Crispr-Cas9 within ongoing global efforts to address pressing biological challenges. Future developments could include integration with artificial intelligence or other emerging technologies, pushing the boundaries of what is possible in genetic modification. This optimistic outlook, coupled with the existing challenges, delineates a transformative pathway for the future of science.
Overall, recognizing the implications of Crispr-Cas9 is essential for students, researchers, and professionals alike. A comprehensive grasp of its capabilities and limitations is necessary in advancing both the technology itself and its applications in society.
Summary of Key Points
- Crispr-Cas9 Overview: This revolutionary tool has altered genetic engineering by allowing precision in editing DNA.
- Diverse Applications: Its utility spans across medical interventions, agricultural enhancements, and industrial biotechnological applications.
- Ethical Considerations: The contemplation of ethics in genetic modification reflects on society's responsibility toward future generations.
- Future Perspectives: The evolution of Crispr technologies signifies not just scientific advancement, but also the need for responsible governance in bioengineering.
Crispr-Cas9 in the Broader Scientific Context
Crispr-Cas9 technology operates within a larger framework of scientific exploration aimed at addressing some of humanity's most pressing challenges. Its development indicates a confluence of multiple disciplines, including molecular biology, genetics, and bioinformatics. Understanding this interplay is crucial for anyone involved in genetic research.
This technology sets the stage for further advancements, such as synthetic biology, where biological systems can be re-engineered to produce novel functionalities and products. Researchers are already investigating how Crispr can be used alongside other gene-editing tools, potentially enhancing efficiency and precision.
Moreover, the global pandemic has underscored the urgent need for rapid advancements in medical technology. Crispr-Cas9 may play a vital role in swiftly addressing infectious diseases through targeted interventions, ultimately emphasizing its importance in public health.