Understanding mRNA Sequences: A Comprehensive Study
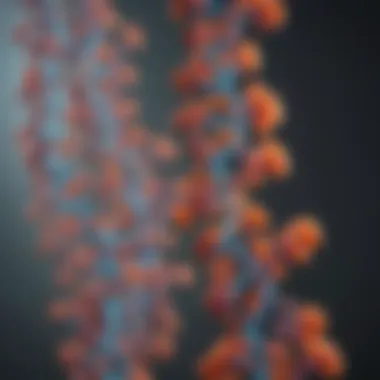
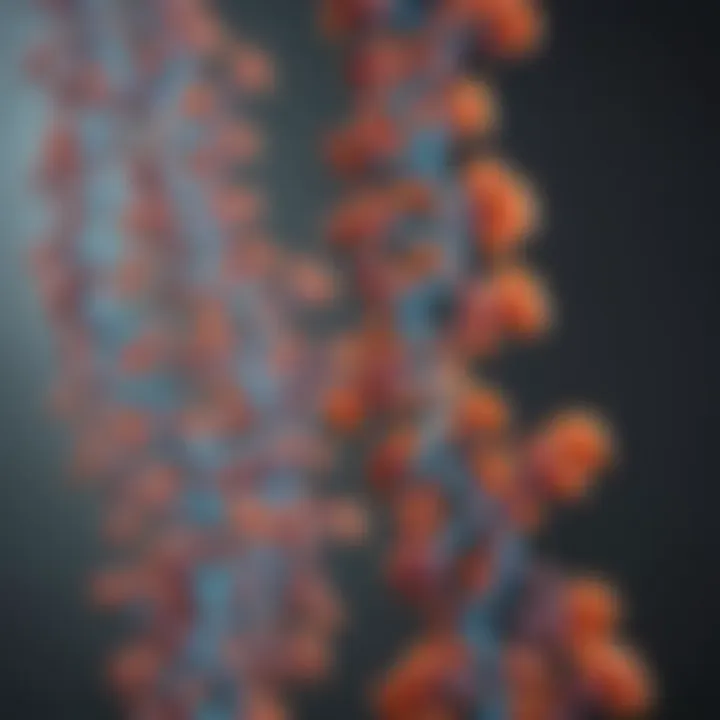
Intro
mRNA sequences play a critical role in the expression of genes across diverse biological systems. They serve as the intermediaries that transfer genetic information from DNA to ribosomes, where proteins are synthesized. Understanding these sequences is foundational for various fields, including genetics, molecular biology, and biotechnology. The focus of this analysis will revolve around the processes relevant to the synthesis and function of mRNA, with an emphasis on their derivation from DNA.
In this exploration, we will discuss how transcription operates, the biochemical mechanisms involved, and the significance of different sequences within mRNA. The implications of these mRNA sequences extend to cellular function and organism development. With advances in genetic engineering, this knowledge becomes even more pertinent as we explore applications in medicine and agriculture.
This article is structured to cater to both newcomers and seasoned researchers. With a balance of theoretical insights and practical applications, readers can expect a comprehensive understanding of mRNA and its critical role in biology.
Research Overview
Summary of Key Findings
The analysis highlights several key findings regarding mRNA sequences:
- mRNA is synthesized through a process called transcription, where RNA polymerases play a vital role.
- The sequence and structure of mRNA are crucial for its function in protein synthesis.
- Variations in mRNA sequences can have significant implications for gene expression and cellular function.
- Recent advancements in genetic engineering techniques, like CRISPR, have opened new avenues for manipulating mRNA.
Research Objectives and Hypotheses
The primary objectives of this research include:
- Examining the molecular mechanisms of transcription in detail.
- Analyzing the importance of specific mRNA sequences in protein synthesis.
- Evaluating how new technologies can harness mRNA for practical applications in various fields.
The underlying hypothesis states that a deeper understanding of mRNA formation and function will lead to significant advancements in genetic tools and therapeutic applications.
Methodology
Study Design and Approach
The study uses a qualitative approach that incorporates literature reviews and experimental data from recent studies. By synthesizing these diverse sources, a comprehensive narrative about mRNA sequences is formed.
Data Collection Techniques
Data is gathered through several techniques, including:
- Review of peer-reviewed journal articles and scientific papers.
- Analysis of experimental conclusions published in high-impact journals like Nature and Science.
- Interviews with experts in the field of molecular biology, ensuring insights are aligned with the latest findings.
This multifaceted approach allows for a thorough overview, integrating both the latest research and foundational knowledge on mRNA sequences derived from DNA.
Understanding transcription and mRNA functions is crucial for developments in genetic research and biotechnology.
Preface to mRNA and DNA
Understanding mRNA and DNA is crucial in molecular biology and genetics. These nucleic acids are fundamental to life and provide the framework for everything from cellular function to heredity. This section will cover the definitions and roles of DNA and mRNA, illuminating bridges between these two vital macromolecules.
Defining DNA and mRNA
DNA, or deoxyribonucleic acid, is the molecule that carries genetic information in organisms. Its structure is a double helix, consisting of two strands of nucleotides twisted around each other. Each nucleotide contains a phosphate group, a sugar molecule, and a nitrogenous baseβadenine, thymine, cytosine, or guanine. The specific sequence of these bases encodes the genetic instructions necessary for development and function.
mRNA, or messenger RNA, acts as an intermediary between DNA and protein synthesis. It is a single-stranded molecule synthesized from a DNA template during transcription. The sequence of bases in mRNA is complementary to the DNA sequence, except that uracil replaces thymine. This unique structure allows mRNA to carry genetic information from the nucleus to the cytoplasm, where it directs the synthesis of proteins.
The Role of Nucleic Acids in Genetics
Nucleic acids play central roles in the storage and expression of genetic information. DNA is the repository of hereditary information and is inherited from one generation to the next. It is here that genes are located, organized in a specific sequence that dictates phenotypic traits.
In contrast, mRNA serves a more dynamic role. It is the vehicle through which information in DNA is expressed. After transcription, mRNA undergoes various modifications before it is translated into proteins. This process illustrates how genes are expressed and controlled within a cell.
Through understanding mRNA and DNA, we gain insights into biotechnology, medicine, and various other fields. The connection between DNA and mRNA highlights the potential impacts of genetic research on health and industry.
The Process of Transcription
Transcription is a key process in molecular biology. It serves as the bridge between DNA and mRNA, facilitating the expression of genetic information. Understanding transcription allows for a deeper appreciation of how genes are regulated and expressed in various biological contexts. By examining the mechanisms involved, one can grasp the intricacies of gene expression and the underlying molecular biology that drives cellular function.
Overview of Transcription Mechanism
Transcription involves copying a specific segment of DNA into messenger RNA (mRNA). This process begins when the DNA double helix unwinds, exposing the nucleotide sequence of the gene that will be transcribed. The enzyme RNA polymerase plays a crucial role in this process, binding to a specific area called the promoter. This is the starting point for transcription.
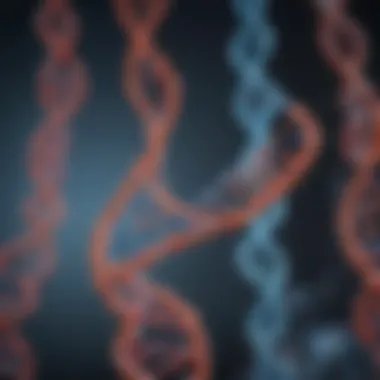
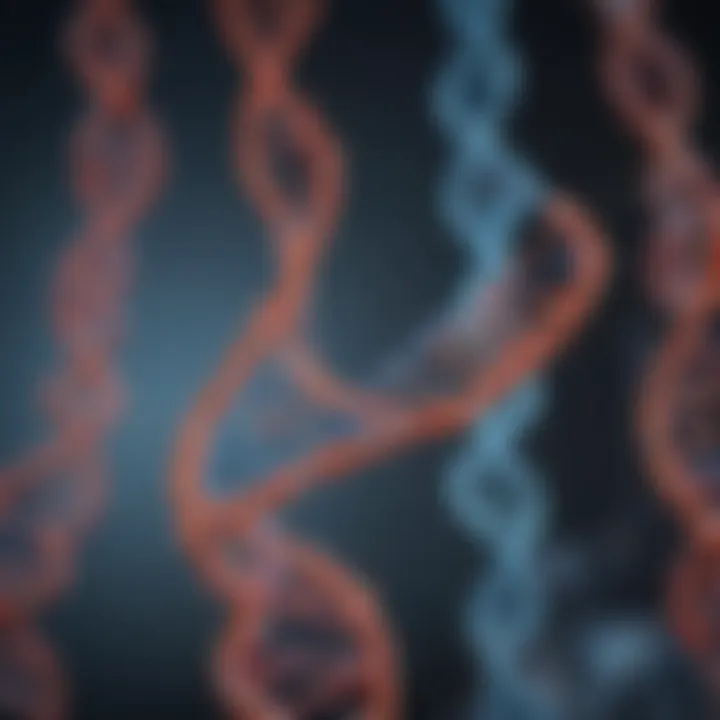
As RNA polymerase moves along the DNA strand, it synthesizes the RNA strand by adding complementary ribonucleotides. This complementary pairing means that adenine on the DNA binds to uracil on the RNA, and cytosine binds to guanine. The end result is a single-stranded mRNA that is a copy of the gene's sequence, ready for translation into protein.
Key Enzymes Involved
RNA Polymerase
RNA polymerase is the central enzyme in transcription. Its primary function is to synthesize RNA from a DNA template. This enzyme catalyzes the elongation of the RNA strand by progressively adding nucleotide units matched to the DNA sequence. One key characteristic of RNA polymerase is its ability to initiate transcription without the need for a primer, which is essential for a rapid response to cellular signals.
The unique feature of RNA polymerase is its iterative approach, allowing the enzyme to transcribe multiple genes efficiently in a single cellular event. This property enhances gene expression levels and ensures timely protein production. However, the enzyme's processivity could be affected by various factors such as transcription factors or other regulatory molecules, leading to potential challenges in gene expression.
Transcription Factors
Transcription factors are proteins that influence the transcription process. They bind to specific DNA sequences, usually near the promoter region, and play a crucial role in regulating gene expression. One beneficial aspect of transcription factors is their specificity; they can enhance or repress the activity of RNA polymerase on individual genes.
Additionally, transcription factors can form complexes with other proteins, thus allowing for a more integrated control of gene expression. However, the reliance on these factors can also present disadvantages. Disruptions in their functions can lead to improper gene regulation, potentially resulting in various diseases.
Understanding both RNA polymerase and transcription factors is essential in appreciating the complexities of transcription. Their interplay is critical for the proper expression of genes across various cellular contexts.
mRNA Synthesis: The Details
mRNA synthesis is a crucial aspect of gene expression. It bridges the gap between the genetic blueprint contained in DNA and the functional proteins that carry out numerous tasks in living cells. Understanding this synthesis provides insight into both fundamental biology and applied sciences, including genetic engineering and biotechnology.
In this section, we will discuss the components and processes involved in mRNA synthesis with a focus on initiation, elongation, and termination phases. These detailed aspects are essential for students, researchers, and professionals who desire a clear understanding of how mRNA molecules are formed and their implications in various fields.
Initiation of Transcription
Initiation marks the first step in the transcription process, where the DNA helix unwinds, allowing access to the template strand. The RNA polymerase enzyme binds to a specific region of the DNA known as the promoter. This region contains sequences that signal the start of a gene. The recruitment of transcription factors is also essential during initiation. These proteins help stabilize the RNA polymerase at the promoter and make necessary modifications to the chromatin structure.
A key point of consideration during this phase is the precise recognition of the promoter. Errors in this step can lead to incorrect transcription or silencing of genes, which can have significant biological consequences. The formation of the transcription complex is a tightly regulated process, ensuring fidelity in the initiation of mRNA synthesis.
Elongation and Termination
Once transcription initiation is complete, the elongation phase begins. RNA polymerase moves along the DNA template strand, synthesizing a complementary mRNA strand. This mRNA strand grows as nucleotides are added one by one. The RNA molecule undergoes several structural changes as it elongates. The transcription machinery moves in the 5' to 3' direction, synthesizing mRNA that reflects the sequence of the coding strand of DNA.
Termination is the final phase of mRNA synthesis. This process occurs when the RNA polymerase encounters a termination signal within the DNA sequence. Upon reaching this signal, it stops transcribing and releases the newly synthesized mRNA molecule. This mRNA is not yet mature, as it will need to undergo several post-transcriptional modifications before it is ready for translation.
The accurate execution of elongation and termination is fundamental because any errors could lead to incomplete or dysfunctional mRNA molecules.
Understanding these processes of mRNA synthesis is vital for advancing research in gene expression. As technologies evolve, new approaches to manipulate the transcription machinery are being explored, offering pathways to enhance mRNA stability and translation efficiency for biotechnological applications.
Comparative Analysis of mRNA and DNA Sequences
Understanding the relationship between mRNA and DNA sequences is crucial. This analysis goes beyond mere nucleotide comparison; it peels back the layers of genetic information. By examining these sequences, we gain insights into cellular functions, gene expression, and potential implications for biotechnology.
Sequence Composition Differences
From a structural perspective, mRNA and DNA exhibit distinct composition features.
- Nucleotide Differences: DNA contains deoxyribonucleic acid, featuring deoxyribose sugar, whereas mRNA comprises ribonucleic acid, containing ribose sugar. This distinction influences stability and function.
- Base Variations: In terms of bases, DNA uses thymine, while RNA uses uracil. This subtle difference can affect the way these molecules interact with other cellular components.
- Strand Configuration: DNA is typically double-stranded, forming a helical structure, contrasted by the single-stranded nature of mRNA. This structural variance plays a role in how genetic information is accessed during transcription and translation.
Further exploration reveals the functional ramifications of these differences. The presence of unstable ribose in mRNA allows for a more flexible structure, promoting dynamic interactions during protein synthesis.
Functional Implications of Sequence Variations
The variations observed between mRNA and DNA sequences have significant functional implications. Differences in sequence composition correlate with how genes are expressed and regulated within the cell. Here are some key points to consider:
- Gene Regulation: The presence of specific regulatory elements in mRNA can influence transcriptional activity. Enhancers and silencers, when present in DNA, often dictate when and how much of a gene product is made.
- Protein Formation: Codon usage in mRNA directly affects the translated proteinβs characteristics. Variations in these sequences can lead to different isoforms of proteins, influencing cell behavior and function.
- Mutation Impact: A point mutation in the DNA sequence may translate to a change in the corresponding mRNA. This could lead to altered proteins, which might have a range of consequences, from beneficial adaptations to detrimental diseases.
"Understanding mRNA and DNA sequence differences can reveal potential pathways for advancements in gene therapy and biotechnology."
Post-transcriptional Modifications
Post-transcriptional modifications play a crucial role in mRNA processing. After mRNA is synthesized from DNA, it undergoes several transformations that enhance its stability and functionality. These modifications are vital for the regulation of gene expression and ensuring that the mRNA can be effectively translated into proteins. The most significant modifications include capping, polyadenylation, and splicing mechanisms, which collectively ensure that mRNA can successfully exit the nucleus and reach the cytoplasm.
Capping and Polyadenylation
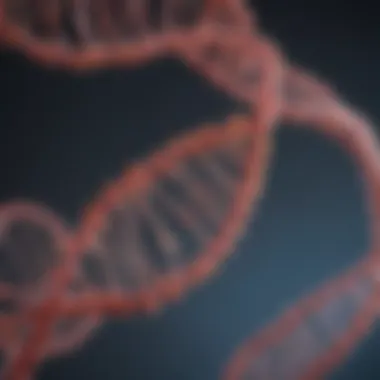
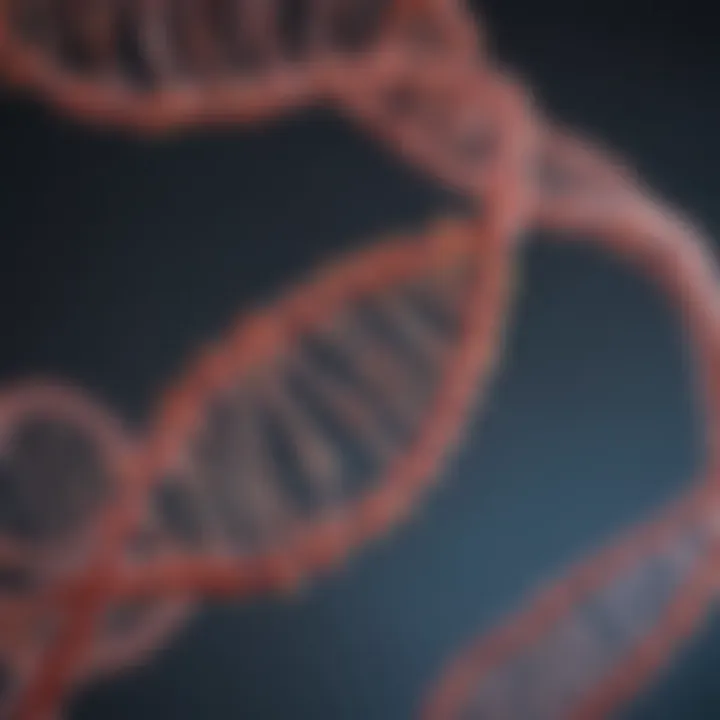
Capping is a chemical modification that occurs at the 5' end of the mRNA molecule. This modification involves the addition of a 7-methylguanylate cap, which protects the mRNA from degradation by exonucleases. The cap also serves as a recognition signal for ribosomes, facilitating the efficient initiation of translation. The presence of the cap is essential for the stability of the mRNA, influencing both its longevity within the cellular milieu and its translational fidelity.
Polyadenylation, on the other hand, refers to the addition of a long sequence of adenine nucleotides at the 3' end of the mRNA. This poly-A tail enhances mRNA stability and aids in the export of mRNA from the nucleus to the cytoplasm. Furthermore, the poly-A tail is also significant in the initiation of translation and can influence the half-life of the mRNA molecule.
"Post-transcriptional modifications are essential for mRNA stability, translation, and overall gene expression. Without them, mRNA would be rapidly degraded before being translated into functional proteins."
Splicing Mechanisms
Splicing is another critical aspect of post-transcriptional modification, characterized by the removal of non-coding sequences, known as introns, from the pre-mRNA. This process allows for the joining of coding sequences, called exons, resulting in a mature mRNA molecule that can be translated into a protein. Splicing is carried out by a complex known as the spliceosome, which is composed of small nuclear RNAs and proteins.
The alternative splicing mechanism allows a single gene to produce multiple protein isoforms, increasing the complexity of protein expression. This flexibility is fundamental to cellular differentiation and the overall adaptability of an organism to its environment. Errors in splicing can lead to various diseases, underscoring the importance of accurate post-transcriptional modifications.
In summary, post-transcriptional modifications are indispensable for the proper functioning of mRNA. They not only protect and stabilize the mRNA molecule but also enhance its translation efficiency and enable diverse protein production. Understanding these processes is critical for advancing fields such as genetic engineering and biotechnology, as they play a pivotal role in manipulating gene expression.
The Role of Regulatory Elements
Regulatory elements are critical components in the transcription of genes into mRNA. They serve as the switchboard for gene expression, determining when, where, and how much gene is expressed. Without these elements, the transcription process would be chaotic and unregulated, leading to potential dysregulation of cellular function.
The presence of regulatory elements ensures that specific genes are activated or silenced in response to various cellular signals or conditions. Key regulatory elements include promoters, enhancers, silencers, and insulators. Each of these plays a unique role in enriching the complexity of gene regulation. Understanding these roles is essential for grasping how cells respond to their environment and maintain homeostasis.
Promoters and Enhancers
Promoters are sequences located at the beginning of a gene that signal to RNA polymerase to begin transcription. They contain specific DNA sequences that bind transcription factors, which are proteins that help regulate gene expression.
Enhancers, on the other hand, can be located far from the gene they regulate. They enhance the transcription of specific genes by providing additional binding sites for transcription factors. This distant action can form loops in the DNA, bringing enhancers and promoters into close proximity. The interplay between promoters and enhancers amplifies the geneβs expression and responds dynamically to cellular signals.
Key characteristics of promoters and enhancers include:
- Specificity: Different genes have unique promoter and enhancer sequences.
- Binding Affinity: Strength of the interaction between the factors and the DNA is crucial for effective regulation.
- Context Dependence: The activity of these elements can vary depending on cell type and environmental signals.
"The precise regulation of gene expression through promoters and enhancers is foundational for developmental processes and adaptability to environmental changes."
Silencers and Insulators
Silencers are sequences that inhibit transcription when bound by specific proteins called transcriptional repressors. Their function provides a necessary counterbalance to enhancers, ensuring that not all genes are turned on simultaneously. This regulation is vital for maintaining cell identity and function.
Insulators are boundary elements that protect genes from inappropriate activation by nearby enhancers. They act as barriers that separate distinctly regulated genes, ensuring that the influence of enhancers is limited to their target genes. By doing this, insulators help maintain the integrity of gene expression in complex genomes.
Some key points about silencers and insulators include:
- Regulation of Expression: They are essential in determining which genes remain inactive under certain conditions.
- Genomic Architecture: Their presence contributes to the overall three-dimensional structure of the genome, affecting how genes interact with each other.
- Cellular Context: The action of silencers and insulators can change with the stage of development or cell type.
Understanding these regulatory elements provides significant insight into the mechanisms governing gene expression. This knowledge has vast implications for biotechnology, gene therapy, and the understanding of diseases linked to gene dysregulation.
Applications in Biotechnology
The integration of mRNA technologies into biotechnology has revolutionized many fields, including medicine, agriculture, and synthetic biology. The distinct characteristics of mRNA offer advantages that are pivotal for various applications. By understanding how mRNA can be harnessed, researchers can advance solutions to some of the most pressing health and environmental challenges.
In the realm of medicine, mRNA has emerged as a critical tool. One key benefit is its speed of development. Unlike traditional vaccines, which often require weeks or months for preparation, mRNA vaccines can be designed in a matter of days. This was notably demonstrated during the COVID-19 pandemic, where rapid development was essential.
Another important aspect to consider is the adaptability of mRNA vaccines. They can be tailored to produce specific antigens effectively. This flexibility makes them suitable for not just infectious diseases but also for cancers and other illnesses where personalized treatments are desired.
When discussing the use of mRNA, several considerations should be acknowledged. The stability of mRNA outside a controlled environment is less than that of DNA. Therefore, proper delivery methods, such as lipid nanoparticles, are critical to ensuring the effectiveness of mRNA applications. Additionally, potential side effects must be studied and understood thoroughly in clinical applications to guarantee patient safety.
Overall, the importance of mRNA in biotechnology cannot be overstated. Its unique properties and quick adaptability provide a remarkable foundation for future innovations.
mRNA Vaccines: A Case Study
The case of mRNA vaccines serves as a prime example of how mRNA technology can transform public health responses. The rapid emergence of the Pfizer-BioNTech and Moderna vaccines during the COVID-19 crisis highlighted both the potential and the efficacy of mRNA technology in generating swift immunity against viruses. These vaccines utilize modified mRNA to instruct cells to produce a harmless piece of the spike protein found on the surface of the virus.
This induced immune response trains the body to recognize and combat the actual virus if encountered. Studies have shown that these vaccines can provide robust immunity, leading to lower infection rates and reduced transmission.
Furthermore, this approach is paving the way for future applications. Researchers are now looking into mRNA strategies for other pathogens, such as influenza, Zika virus, and HIV. The lessons learned from mRNA vaccines during the pandemic are likely to influence vaccine development for years to come.
Gene Therapy Approaches
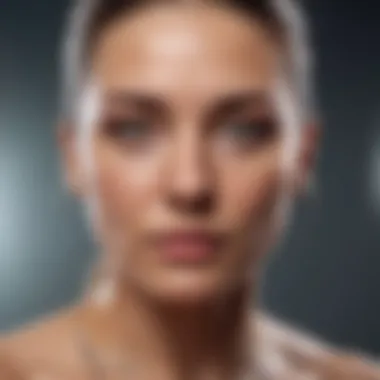
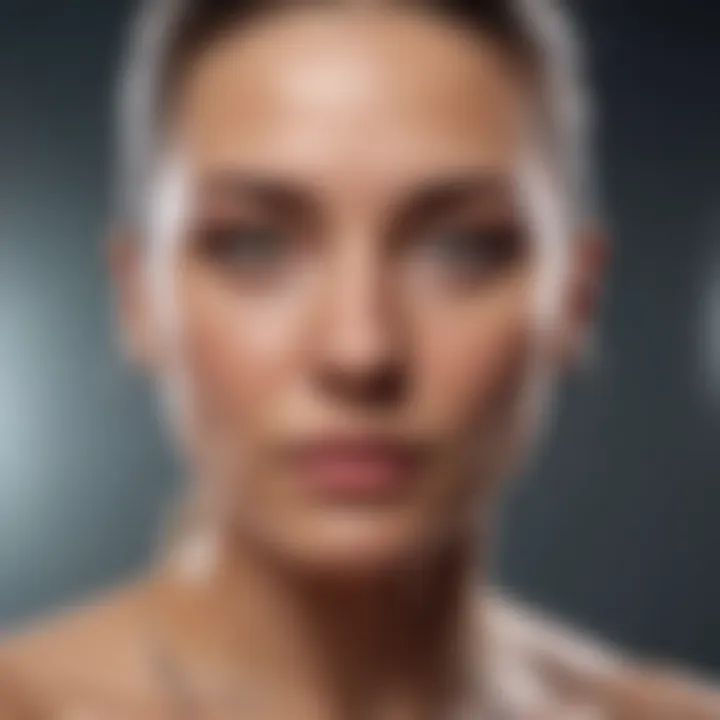
Gene therapy is another fascinating application of mRNA technology. Instead of traditional methods that focus on inserting new genes into cells, mRNA therapy aims to provide a temporary boost to the presence of specific proteins. This can be particularly beneficial in treating genetic disorders caused by a lack of functional proteins.
For instance, in diseases like cystic fibrosis, where mutations lead to absent or dysfunctional proteins, delivering the correct mRNA can temporarily restore protein function in target cells. This method allows for reversible treatments, minimizing long-term complications that could arise from permanent gene editing.
Moreover, ongoing research is exploring approaches to use mRNA in the treatment of muscular dystrophy and certain types of cancer. In these cases, the ability of mRNA to instruct cells to produce therapeutic proteins is invaluable and could change standard treatment paradigms.
In summary, mRNA applications in biotechnology are both broad and intricate. Whether through the speed and efficacy of mRNA vaccines or the innovative potential of gene therapy, the continued development and understanding of mRNA will undoubtedly lead to significant advancements in health and disease management.
Challenges and Considerations
In any field of biological research, especially in the realm of molecular genetics, it is essential to address the challenges and considerations that arise. When discussing mRNA sequences derived from DNA, many factors come into play. These factors can impact both the scientific research landscape and real-world applications. More than just a technical problem, these challenges can lead to ethical considerations and societal implications that require careful thought.
Stability of mRNA Molecules
The stability of mRNA molecules is a critical concern in any analysis involving gene expression. mRNA is inherently unstable and undergoes rapid degradation in cellular environments. The half-life of mRNA can vary significantly; many mRNA transcripts are degraded within minutes to hours of synthesis. This instability poses challenges for scientists who aim to study gene expression patterns or use mRNA for therapeutic purposes.
To enhance the stability of mRNA, several strategies have been developed:
- Modification of the nucleotide structure: Incorporating modified nucleotides into the sequence can help reduce degradation. For instance, using pseudouridine can improve mRNA stability and translational efficiency.
- Capping and Polyadenylation: The addition of a cap structure at the 5β end and a poly(A) tail at the 3β end significantly protects the mRNA from exonucleolytic attack.
These enhancements are essential in biotechnology applications, such as mRNA vaccines, where a stable mRNA is crucial for eliciting a robust immune response. Understanding and improving mRNA stability enhances the effectiveness of therapies and contributes to advancing genetic engineering.
Ethical Implications in Genetic Engineering
Genetic engineering raises ethical questions that cannot be ignored, especially as it relates to mRNA technologies. The power to manipulate genetic material holds promise for advancements but also poses significant risks. As researchers and practitioners explore mRNA applications like vaccines or gene therapy, it is vital to consider the broader impact on society.
The ethical implications can range from concerns over genetic privacy to the potential for unintended consequences in gene editing. Some key considerations include:
- Informed consent: Individuals have the right to understand how mRNA technologies can affect them. This principle applies particularly to clinical trials and therapies, where participants must be fully informed about potential risks and benefits.
- Equity in access: As genetic therapies evolve, ensuring equitable access to these technologies is crucial. Disparities in healthcare can lead to a divide where only certain populations can benefit.
- Long-term effects: The long-term effects of mRNA therapies are still under investigation. There are concerns about potential off-target effects or unintended mutations.
"As we push the boundaries of science, we must also focus on the ethical frameworks that guide these advancements."
Future Directions in mRNA Research
The study of mRNA sequences derived from DNA is evolving rapidly. Current advancements pave the way for significant developments in numerous areas of biology and medicine. Understanding future directions in mRNA research is vital for various scientific and practical applications. In particular, these advancements promise to enhance comprehension of how mRNA functions and its implications for health and disease.
Emerging Technologies in mRNA Analysis
Recent progress in technology has introduced new methods for mRNA analysis. Techniques like single-cell RNA sequencing allow unprecedented insights into gene expression at the individual cell level. This technology aids in understanding how different cells in the same organism can exhibit varied behavior based solely on mRNA differences.
Another significant advancement is the CRISPR technology. It enables precise modifications to mRNA through targeted editing. This can be important in research and therapeutic applications where altering gene expression may result in treating specific diseases.
Consider the following technologies:
- Nanopore Sequencing: This technology allows real-time sequencing of RNA molecules. Unlike traditional methods, it provides rapid data output and is less dependent on sample preparation.
- Digital Droplet PCR (ddPCR): A high-sensitivity method for quantifying mRNA. It allows researchers to detect low-abundance transcripts that may play critical roles in disease.
- Massively Parallel Signature Sequencing (MPSS): This technique provides quantification and sequencing of millions of transcripts simultaneously, enhancing throughput and data richness.
Together, these technologies advance mRNA research and unravel complex biological systems with greater fidelity.
Potential for Personalized Medicine
Personalized medicine utilizes individual genetic information to tailor treatments. In this context, mRNA research holds great promise. By analyzing specific mRNA profiles of patients, researchers can find unique markers for diseases. This enables a targeted approach in therapy, increasing efficacy while minimizing side effects.
For instance, cancer treatments increasingly focus on personalized strategies based on mRNA expression signatures. Specific mRNA variations have been correlated with the effectiveness of certain therapies. Thus, treatments can be designed to match the patient's specific mRNA profile, improving outcomes significantly.
Benefits of personalized medicine through mRNA understanding include:
- Enhanced treatment effectiveness based on individual mRNA profiles.
- Reduction in trial-and-error approaches in prescribing medications.
- Improved patient satisfaction and adherence to treatment protocols.
Future directions in mRNA research indicate a growing focus on personalized medicine, which continues to shape the landscape of healthcare. Innovation in mRNA analysis remains essential to achieving more responsive and tailored health interventions.
Culmination
The study of mRNA sequences, derived from DNA, is crucial to understanding the intricate processes of gene expression and regulation. This article has provided a comprehensive overview of the various aspects of mRNA and its synthesis, highlighting the fundamental mechanisms involved in transcription. These mechanisms play a vital role in translating genetic information into functional proteins, which are essential for cellular processes and overall organismal health.
Summary of Key Points
- Definition of mRNA and DNA: The distinction between messenger RNA (mRNA) and deoxyribonucleic acid (DNA) lies in their structure and function. While DNA serves as the genetic blueprint, mRNA acts as a copy that carries instructions to the ribosomes.
- Transcription Process: The transcription process was detailed, emphasizing the roles of RNA Polymerase and transcription factors. These components work together to initiate, elongate, and terminate the synthesis of mRNA from a DNA template.
- Post-Transcriptional Modifications: The importance of modifications such as capping, polyadenylation, and splicing was discussed, showing how they enhance mRNA functionality and stability.
- Regulatory Elements: The role of promoters, enhancers, silencers, and insulators in gene regulation demonstrates the complexity of gene expression control.
- Applications in Biotechnology: The article covers the real-world implications of mRNA research, including mRNA vaccines and gene therapy, which highlight its potential in advancing healthcare.
- Challenges in mRNA Stability: Stability of mRNA molecules was identified as a critical factor that influences their efficacy in therapeutic applications, necessitating ongoing research in this area.
Implications for Future Research
The exploration of mRNA sequences opens new avenues for research that could significantly impact various fields. The continuous evolution of genomic technology allows researchers to delve deeper into areas such as:
- Personalized Medicine: As more is understood about the unique mRNA profiles of individuals, the potential for tailored treatment plans based on specific genomic data becomes more accessible.
- Gene Therapy Advances: Furthering research into mRNA technology can lead to novel therapies for genetic disorders, enhancing our capacity to tackle previously untreatable conditions.
- Enhanced mRNA Stability Techniques: Developing methods to enhance the stability and efficacy of mRNA molecules in therapeutic applications will be paramount in overcoming current limitations.