Understanding the Intricacies of Metal Manufacturing
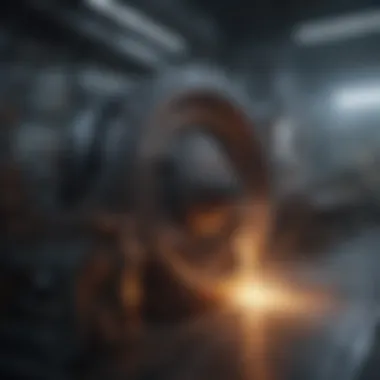
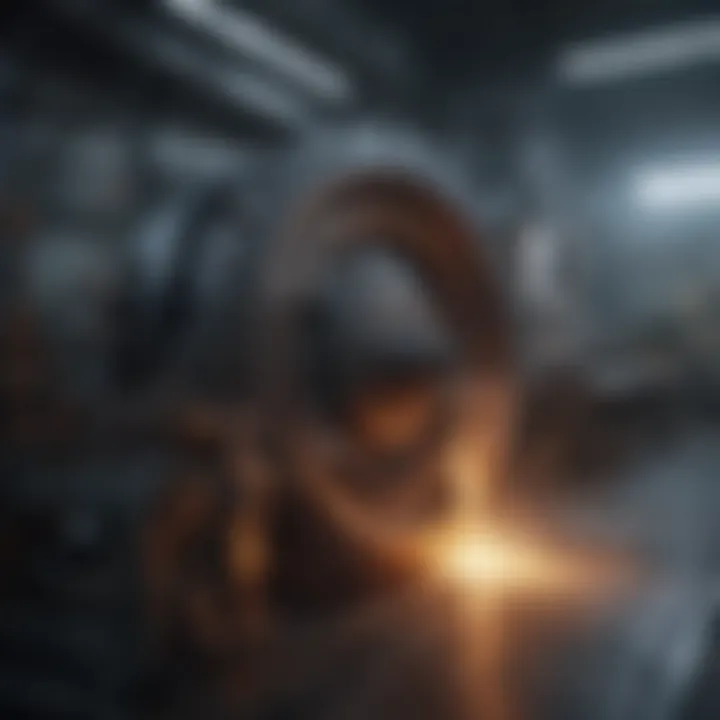
Intro
The world of metal manufacturing isn't just about blending raw materials together and calling it a day. It's an intricate dance that involves various elements, from ancient methodologies to cutting-edge technologies. This article dives into the multifarious aspects of how metals are crafted, the journey each material takes from the ground to its final form, and the forces that shape this essential industry.
Metal production has morphed significantly from the days of blacksmiths hammering away in dimly lit forges. Today, it's as much about precision engineering and sustainability as it is about tradition. Understanding these complexities means recognizing the delicate balance between history, innovation, and the environment. From the extraction of ores to the tedious processes of refinement, each stage is a crucial part of a larger narrative that we will unravel.
As we move through this exploration, keep in mind the pivotal role metal plays in modern society. Whether it's the steel in our buildings, the aluminum in our smartphones, or the copper in our electrical systems, the implications of metal manufacturing stretch far and wide. With that in mind, let’s embark on this journey to uncover the hidden intricacies behind metal production.
Historical Context of Metal Manufacturing
Understanding the historical context of metal manufacturing is key to appreciating how far we’ve come in this industry. It is not just about a timeline but also about the evolution of techniques, tools, and materials used. Each era contributes to the foundation upon which modern metalworking stands.
Metal has been an integral part of human civilization since the dawn of metallurgy. This evolution reflects not only technological progress but also shifts in social structures, economic systems, and even culture. By exploring these historical phases, we can glean insights into contemporary practices and the complexities they entail.
Ancient Metallurgy
Ancient metallurgy marks the beginning of mankind's manipulation of metals for practical use. The earliest records harken back to 6000 B.C.E., with societies in places like Mesopotamia and Egypt harnessing naturally occurring metals. These civilizations used meteoric iron, which, unlike most other metals, does not require extensive processing. Tools and ornaments made from native copper emerged soon after, signifying the shift from the Stone Age to the Metal Age. As they say, necessity is the mother of invention, and the burgeoning agrarian lifestyle generated a demand for more robust tools.
The techniques of smelting became crucial. Early humans learned to heat ores mixed with charcoal, which reduced ores into metal. It was a slow process, often inconsistent, leading to the distinct characteristics of early metal tools—prone to rust and easily breakable. Nonetheless, these early experiments were vital. They showcased mankind's relentless pursuit of innovation, fine-tuning their approaches over generations.
"The past is a guide for the future."
For instance, almost every civilization encountered the challenge of metalworking. The Phoenicians excelled in bronze work, a blend of copper and tin, enabling stronger and more durable tools. Their approach towards alloying can be seen as the foundation of modern metallurgy, where chemical compositions dictate properties. As copper and tin became scarce in regions close to their trade networks, innovations perpetually adapted to the availability and utility of resources, highlighting the dynamic relationship between environment and technology.
Industrial Revolution in Metalwork
The Industrial Revolution was a watershed moment for metal manufacturing, marking a transition from artisanal handcraft to mass production. It began in Britain in the late 18th century and forever changed how metals were extracted, produced, and utilized. Led mainly by innovations in steam power and advanced machinery, the mechanization of processes resulted in unprecedented productivity levels.
One of the most noteworthy advancements was the Bessemer process, developed by Henry Bessemer in the 1850s, which facilitated the mass production of steel. This marked a significant shift because steel could now be made cheaper and in larger quantities than ever before. As cities expanded and industries burgeoned, the need for stronger materials grew, and this process fulfilled that demand efficiently.
Notably, factories sprang up across Europe and the United States, emphasizing specialization in production. Tasks that were once accomplished by skilled artisans were now divided into simpler operations, facilitating faster manufacturing at a lower cost. Women and children often made up the workforce, reflecting the societal shifts that accompanied industrialization, yet bringing to light the challenges of labor rights as well.
Despite the tremendous progress, the Industrial Revolution did not come without its complications. Environmental degradation, a byproduct of unregulated metalworking, began to surface. This era laid the groundwork for future considerations in sustainable manufacturing practices—a topic still hotly debated today.
Essential Properties of Metals
Understanding the essential properties of metals is not just a technical necessity; it lays the foundation for everything that follows in metal manufacturing. These properties impart characteristics relevant to application, performance, and usability, making them indispensable when developing tools, structures, and machines. Grasping these properties supports manufacturers in selecting the right materials for specific tasks, ultimately influencing quality and durability.
Physical Characteristics
Density
Density plays a critical role in metal manufacturing. It refers to the mass per unit volume and varies significantly among different metals. A higher density implies a greater mass in the same volume, which can offer greater strength but can also weigh down the final product. For example, lead, with its high density, is often used in applications requiring radiation shielding, even though it can make products cumbersome.
Density's key characteristic is its influence on material selection for specific applications. Lighter metals like aluminum are popular for their favorable density, especially in aerospace and automotive industries where reducing weight can improve efficiency and fuel economy. Conversely, while dense metals provide advantages in strength, they may be less suitable for lightweight applications.
A unique feature of density is how it correlates with other properties, such as tensile strength and thermal conductivity, thus influencing mechanical performance. However, a disadvantage of focusing too heavily on density can lead to over-engineered solutions that may be unnecessarily expensive or impractical.
Melting Point
The melting point of a metal indicates the temperature at which it transitions from solid to liquid. This property is paramount for processes that involve casting and welding, where precise temperature control is required. For instance, steel has a melting point that enables it to withstand high temperatures during forging, yet it can also be remelted for recycling purposes.
A striking characteristic of melting point is its direct impact on manufacturing processes. A metal with a low melting point, like tin, allows for simpler casting but may not be suitable for high-stress applications. On the other hand, metals like tungsten, with extremely high melting points, are essential in high-performance applications such as aerospace.
The unique feature of melting points varies across metal types, influencing how they are processed and utilized. Balancing the necessity of high melting points against the difficulty of manipulation can create challenges during production, particularly in a cost-sensitive environment.
Conductivity
Conductivity refers to a metal's ability to conduct electricity and heat. Certain metals, like copper, are coveted for their outstanding electrical conductivity, making them the go-to choice for electrical wiring and circuitry. Similarly, metals with high thermal conductivity, like aluminum, are essential in heat exchangers and cooking utensils.
The importance of conductivity lies in its fundamental role in many applications. High conductivity allows for efficient energy transfer, which is particularly critical in electronics where heat management is paramount. However, a drawback of metals with high conductivity can be their susceptibility to corrosion, demanding protective measures in many environments.
A unique aspect of conductivity is how it intersects with other physical and mechanical properties. Metals with exceptional conductivity may not always boast high strength, leading to applications tailored to exploit their specific conductive properties without compromising structural integrity.
Mechanical Properties
Strength
In metal manufacturing, strength is a pivotal property that defines a material's ability to withstand applied forces. It denotes various types of strength, including tensile strength, yield strength, and compressive strength. Utilizing iron, known for its robust strength, metal manufacturers can create structures that can support heavy loads, from skyscrapers to bridges.
Strength is crucial in determining the longevity and reliability of a metal product under various stress factors. The capability to select appropriate alloys, which can enhance strength while maintaining other necessary properties, makes this a vital consideration in manufacturing. However, metals with high strength often face challenges concerning weight and ductility, a balancing act manufacturers must carefully navigate.
Ductility
Ductility reflects a metal’s ability to undergo deformation without breaking, often measured by how far a material can stretch before it fractures. This property is key in processes such as drawing and rolling, where materials are shaped into new forms. Copper is a prime example, known for being both ductile and malleable, allowing it to be transformed into wires efficiently.
The essential characteristic of ductility is its contribution to processing flexibility. Ductile materials can be easily manipulated, which is critical in forming operations and reducing waste. Nevertheless, high ductility can sometimes coincide with reduced strength; thus, manufacturers must weigh the trade-offs in material choice.
Toughness
Toughness is the ability of a metal to absorb energy and plastically deform without fracturing. While strength measures the maximum applied force a material can withstand, toughness adds a layer, indicating how much energy it can absorb before failure. Metals like steel are revered for their toughness and are widely used in construction to ensure durability in challenging environments.
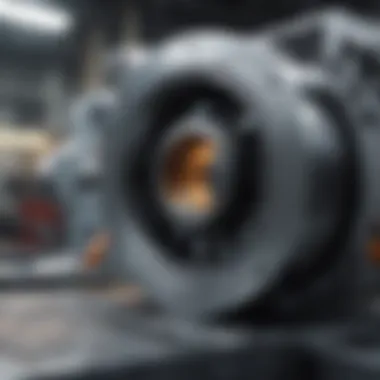
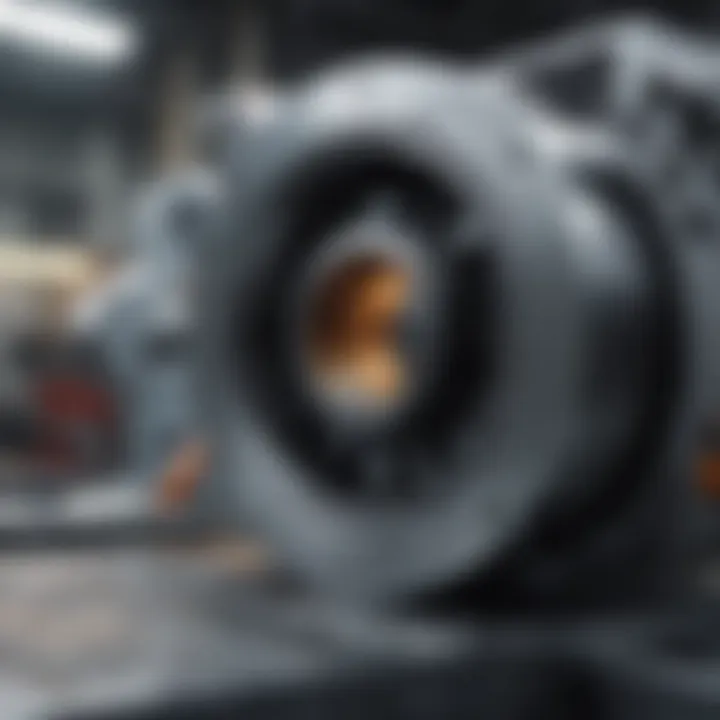
The significance of toughness lies in real-world applications that often expose materials to impact or stress over time. This property ensures that metal structures endure not just initial loads but repeated stresses. However, tough metals may come with a cost in terms of weight or production complexity, requiring careful evaluation by manufacturers to optimize designs for performance.
A balanced understanding of essential properties is vital to making informed decisions during metal manufacturing, ensuring that the end product fulfills both functional requirements and aesthetic aspirations.
Extraction Processes for Metal Production
The extraction processes for metal production are the cornerstone of the entire metal manufacturing industry. This stage lays the groundwork for all subsequent processes, transforming raw materials into usable metals. Without efficient extraction, the entire chain of manufacturing can collapse like a house of cards. Understanding these processes not only highlights their role in supplying metals but also showcases some of the more pressing challenges of sustainability and efficiency that industries face today.
Mineral Mining Techniques
Mining is fundamental in the journey from ore to metal. There are various techniques, each with its own set of methodologies aligned with the type of metal being extracted and the conditions of the mining site. Generally, the two main methods dominate this aspect: surface mining and underground mining.
Surface Mining
Surface mining is like peeling an onion, gradually stripping away layers to expose the core. This technique is widely regarded for its cost-effectiveness and efficiency. The main characteristic that sets surface mining apart is that it operates at the surface level, where ores are directly accessible. It's a popular choice for extracting metals like copper and gold, where the ore bodies are near the topsoil.
However, there are some downsides as well. The disturbance of large areas of land can lead to significant environmental concerns. The key is to balance these factors, making sure the benefits are not drowned out by the consequences. Moreover, the actual process involves removing the overburden, which can create large pits that may take years to rehabilitate.
Underground Mining
Conversely, underground mining is a more concealed method—the hidden behemoth of metal extraction. This approach delves into the earth, accessing deeper ore bodies that surface mining cannot reach. The main benefit of underground mining is its reduced surface footprint, which minimizes environmental disruption compared to its surface counterpart.
However, entering these subterranean realms comes with its own sets of risks and challenges. The complex ventilation systems and safety measures required can lead to higher operational costs. It's a delicate dance between risk and reward, making this method a nuanced choice that demands careful planning and execution.
Beneficiation Methods
Once the raw ores are extracted, they often contain a mix of valuable and worthless material. Beneficiation methods are crucial in concentrating the valuable components and enhancing the quality of the ore before it undergoes refining. This stage encompasses both crushing and grinding, alongside concentration techniques, which are vital in ensuring efficient metal production.
Crushing and Grinding
The primary function of crushing and grinding is to reduce the size of the ore. Imagine trying to make a smoothie with whole fruits—you need to chop them up first. In metal production, the massive rocks housing the metals need to be broken down into smaller pieces. This method is cost-effective and allows for easier processing. The key characteristic lies in the ability to prepares the ore for further enrichment.
On the flip side, it's worth noting that excessive energy consumption during this stage can raise concerns. It is pivotal to find that sweet spot to minimize waste while maximizing output.
Concentration Techniques
After crushing and grinding, the next step is concentration techniques. These methods can effectively separate valuable minerals from gangue or waste material. Technologies such as flotation and magnetic separation play crucial roles here. A striking feature of these techniques is their adaptability; different methods can be tailored to specific ore types.
This adaptability makes them a favorable choice in metal extraction as they can manage various ore qualities and enhance recovery rates. However, the downside is that the need for advanced equipment can require substantial investments, both upfront and in maintenance.
"Metal extraction is not just about getting the ore; it's about every step refining that ore into something valuable for society's needs."
In closing, the extraction processes reflect the complexities of metal manufacturing. They underscore the blend of innovation, sustainability, and scientific advancements that characterize modern extraction methodologies. As we continue to explore what lies ahead, understanding these foundational processes becomes ever more essential, not only for industry professionals but also for the community interested in the direction of metal manufacturing.
Refinement Processes in Metal Manufacturing
The significance of refinement processes in metal manufacturing cannot be overstated. This stage is crucial as it ensures that the raw materials obtained from various extraction processes are transformed into usable metal forms with superior quality and properties. The refinement processes directly influence the durability, strength, and overall performance of the final metal products, making it a core consideration in the manufacturing industry. The benefits of efficient refinement include not only improved material characteristics but also enhanced efficiency in downstream processes. It allows manufacturers to operate at higher yields while minimizing waste and energy consumption.
Smelting
Smelting marks a pivotal phase in the journey from ore to refined metal. During this process, heat is applied to ores, typically mixed with other materials, to encourage chemical reactions that extract metal. The era of high-temperature furnaces became a game changer in smelting processes, fundamentally altering how metals are produced.
Era of High-Temperature Furnaces
The development of high-temperature furnaces represented a leap forward in smelting technology. These furnaces can achieve temperatures that are high enough to completely melt metal ores, allowing for a more thorough extraction process. A key characteristic of such furnaces is their ability to reach temperatures exceeding 1,000 degrees Celsius, often using coke or natural gas as fuel.
One unique feature of high-temperature furnaces is their capacity to be operated continuously, facilitating larger-scale production. The main advantage of this type of furnace is the efficiency it brings to the metal production process. On the flip side, the high operational costs and energy demands can be seen as disadvantages, necessitating a careful cost-benefit analysis for manufacturers.
Methods of Reducing Oxides
Methods of reducing oxides are essential to the refining process, focusing on chemically altering metal oxides back to their metallic state. This is critical, especially for metals that naturally occur as oxides, such as iron. The reduction typically employs methods like carbon reduction or the use of hydrogen, each offering distinct operational characteristics.
A standout feature of oxide reduction methods is their adaptability in terms of the reducing agent used. This variety provides manufacturers with flexible options depending on the raw materials and desired outcomes. The key advantage is the controlled operational conditions that lead to improved recovery rates of the desired metals. However, one must also consider the environmental implications tied to these processes, as the by-products of some reductions can lead to pollution if not managed correctly.
Electrolytic Refining
Electrolytic refining is another sophisticated technique widely adopted in the metal manufacturing realm. This method utilizes an electrolytic solution where metal ions are sent through an electrical current to deposit pure metal onto a cathode. It's particularly lauded for its role in purifying metals such as copper and silver.
Notably, electrolytic refining offers that high purity level which cannot be easily achieved through traditional methods. The method allows for exceptional precision in controlling the thickness of the deposited metal, which can be crucial for specific applications. However, the setup can involve significant initial investment costs, and the process requires rigorous control measures to manage the electrolytic environment effectively. Though it has its challenges, the benefits of electrolytic refining make it a valuable process in modern metal manufacturing, addressing both quality and efficiency needs.
"Refinement processes not only dictate the quality of the metal, but they also encompass the innovation and sustainability of the manufacturing process itself."
In summary, refinement processes such as smelting and electrolytic refining play a vital role in the evolution of metal manufacturing, with distinct techniques offering a mix of benefits and challenges. A deep understanding of these processes enables manufacturers to enhance production efficiency while maintaining the integrity and quality of the metals they produce.
Alloy Formation and Characteristics
Alloys play a crucial role in the metal manufacturing realm. They are not merely combinations of metals but hold key properties that enhance performance and broaden application scopes across industries—from automotive to aerospace, and even art. Understanding the intricacies of alloy formation is fundamental for grasping modern metallurgy. Different elements might be combined, reacting with each other to create materials that oftentimes surpass the individual properties of the constituents. The benefits are profound; alloys can exhibit resistance to corrosion, improved strength, and even specialized features tailored for specific uses. However, it’s not just about mixing metals; there are considerations that manufacturers must account for such as composition ratios, processing techniques, and environmental impacts.
Foreword to Alloys
Alloys can be thought of as a marriage of metals, each partner bringing its strengths to the table while sidestepping their weaknesses. In essence, when you amalgamate elements like iron with carbon, you form steel, which is significantly stronger and more versatile than iron alone. Various phases can emerge within these mixtures based on temperature and processing methods, leading to entirely new characteristics. This opens avenues for innovation. The alloying process can involve melting, dissolving, and cooling, which sometimes adds layers of complexity—yet it allows for custom-tailored materials for diverse applications.
Types of Alloys
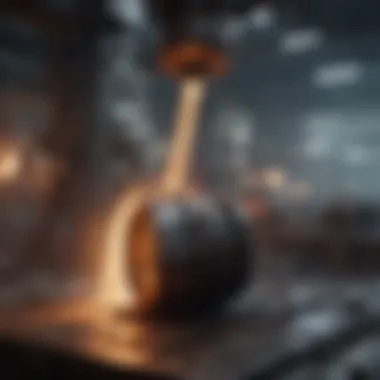
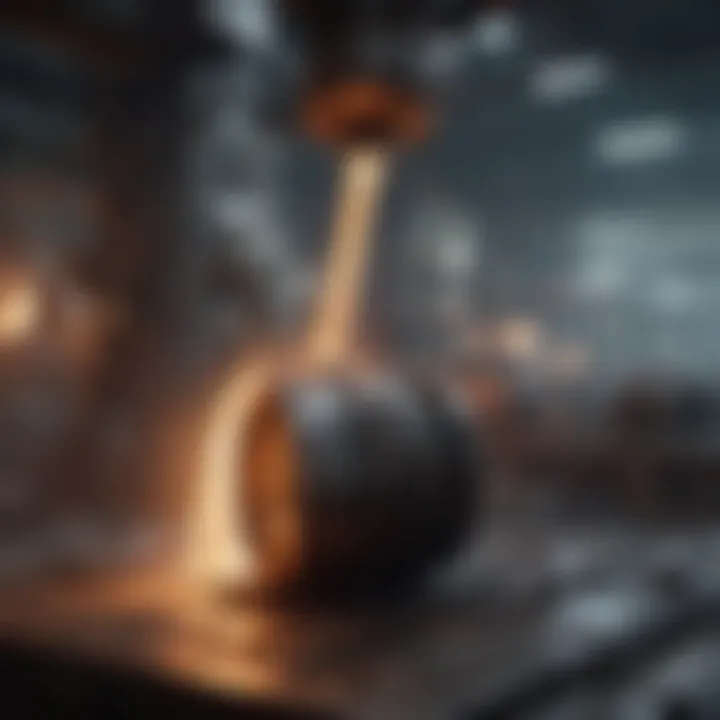
Alloys can be categorized primarily into two classes: Ferrous Alloys and Non-Ferrous Alloys. Each class has its unique properties and applications that significantly contribute to the field of metal manufacturing.
Ferrous Alloys
Ferrous alloys are iron-based and boast a prominent position in the world of manufacturing. One of their key characteristics is high tensile strength, complemented by remarkable ductility. This makes ferrous alloys a go-to choice for structures that demand durability, such as bridges and buildings. Moreover, they’re often more cost-effective compared to their non-ferrous counterparts.
However, they aren't without their drawbacks; ferrous alloys can be prone to corrosion. While manufacturers have developed coatings and treatments to combat this, the necessity for maintenance is an ongoing concern. Also, the weight of these alloys can be a disadvantage depending on the specific application. Their versatility and strength still firmly establish ferrous alloys as a fundamental element in metal manufacturing.
Non-Ferrous Alloys
Non-ferrous alloys, on the other hand, do not contain significant amounts of iron. This type includes materials like aluminum, copper, titanium, and nickel, each bringing distinctive features. One notable aspect is their corrosion resistance, making them ideal for applications in harsh environments, such as marine and chemical processing. The lightweight nature of aluminum alloys is particularly sought after in aerospace applications, where weight savings can lead to fuel efficiency.
Despite their advantages, non-ferrous alloys often come with a higher price tag and sometimes lower tensile strength, which can limit their usefulness in particular applications. Developers must carefully balance performance needs with cost considerations.
Different alloys have their own set of challenges and benefits. The right choice often boils down to the specific requirements of a project—whether that be strength, weight, or resistance to environmental factors.
In summary, understanding the nuances of alloy formation and their characteristics serves as a foundation for successful metal manufacturing. Choices between ferrous and non-ferrous alloys will ultimately dictate not just functionality but also economic viability in any project.
Modern Techniques in Metal Manufacturing
Modern techniques in metal manufacturing represent a definite shift from traditional methods, bringing with them a blend of innovation and efficiency that enhances productivity and reduces waste. This evolution is driven by the need for sustaining performance and cost-effectiveness in a rapidly changing industrial landscape. Technological advancements, including automation and computer-aided processes, are helping companies to optimize their operations and produce higher-quality products. Understanding these advancements is fundamental for students, researchers, and professionals alike, as they shape the future of the metal industry.
Additive Manufacturing
3D Printing with Metals
3D printing with metals stands out as a transformative process in metal manufacturing. This technology offers the ability to create complex geometries that would be near impossible to achieve using conventional methods like machining or casting. One of the key characteristics of this technique is its layer-by-layer approach, which not only allows for intricate designs but also minimizes material waste, contributing significantly to the sustainability aspect of manufacturing.
A unique feature of 3D printing with metals is its adaptability; designs can be easily altered and customized to meet specific requirements of a project without the need for extensive tooling changes. However, this does come with certain disadvantages, including the relatively high cost of metal powders and the need for specialized equipment. Nonetheless, the potential for rapid prototyping and low-volume production makes it an appealing choice for many industries, especially in aerospace and medical applications.
Applications and Challenges
The applications of additive manufacturing in the metal sector are vast, reaching sectors such as aerospace, automotive, and healthcare. A notable characteristic of these applications is the ability to reduce lead times drastically because parts can be printed on demand rather than manufactured through traditional assembly lines. This on-demand production is particularly beneficial in situations where customization is essential or inventory costs need to be minimized.
However, despite these benefits, there are also significant challenges. Ensuring high material quality and mechanical properties equivalent to those of traditionally manufactured parts remains a major hurdle. A unique feature of these applications is the need for rigorous testing and certifications to meet industry standards, which adds layers of complexity to the adoption process. Overall, while there is immense promise in using additive techniques, navigating the related challenges is crucial for their successful implementation in metal manufacturing.
Computer-Aided Design and Simulation
Computer-Aided Design (CAD) and simulation tools have become indispensable in modern metal manufacturing. They allow engineers and designers to visualize and alter their designs in a virtual environment before any physical work begins. These technologies simplify the process of prototyping and testing, ensuring that potential problems can be identified and rectified early in the design phase. Additionally, CAD systems have become more intuitive, enabling seamless integration into the production workflow.
Simulation also plays a crucial role in the manufacturing arena, as it helps predict how materials will behave under various conditions, leading to better-informed decisions about material selection and processing techniques. By leveraging simulation, manufacturers can optimize refining processes to enhance product quality and durability, thus ensuring that the end products meet not just design specifications but also operational expectations.
Quality Control in Metal Manufacturing
Quality control in metal manufacturing plays a crucial role in ensuring that materials meet the required specifications and standards. This process is not just about maintaining product quality; it also involves safeguarding the integrity of the entire production system. By implementing effective quality control measures, manufacturers can enhance operational efficiencies, reduce waste, and ensure customer satisfaction.
A meticulous quality control system helps in identifying any deviations in the production line, which can prevent faulty materials from reaching the market. For instance, through regular assessments, a manufacturer can detect inconsistencies in purity levels, mechanical properties, or surface finish. Therefore, quality control acts as the backbone of reliability in metal manufacturing, acting as a safety net before products reach consumers.
Testing Methods
Effective quality control hinges largely on robust testing methods, which serve as the gatekeepers of manufacturing integrity. These methods can range from simple visual inspections to complex analyses conducted under controlled conditions.
Tensile Testing
Tensile testing measures a material's strength and ductility—the ability to stretch without breaking. This specific testing method involves applying a uniaxial load until the material deforms or fails, providing vital data on how a metal will behave under stress. The valuable insight it offers into material performance makes tensile testing a popular choice in the metal manufacturing industry. Its key characteristic lies in its ability to simulate real-world conditions and predict how metals will perform in application.
The unique feature of tensile testing is the generation of a stress-strain curve, which graphically represents how a material reacts under tension. By analyzing this curve, manufacturers can determine the ultimate tensile strength, yield strength, and elongation, which are critical parameters in assessing material suitability. However, it is important to note that while tensile testing is highly informative, it primarily focuses on tensile properties and may not give a complete picture of material behavior under other forms of stress.
Hardness Testing
Hardness testing explores a material's resistance to deformation, which is critical in applications where surface wear and tear are likely. This method, while straightforward, provides invaluable insights into the potential longevity of a product. The key characteristic of hardness testing is its ability to quantify resistance through various scales, like Brinell, Rockwell, or Vickers.
A standout feature of this type of testing is that it is typically quick and requires minimal sample preparation, making it a beneficial choice for manufacturers looking to expedite the quality control process. However, a disadvantage is that hardness does not always correlate directly with other mechanical properties, thus necessitating complementary testing methods for a more rounded analysis.
Standards and Certifications
Adhering to established standards and certifications ensures that products not only meet industry benchmarks but also comply with safety regulations. Various organizations, like ASTM International or ISO (International Organization for Standardization), provide guidelines that manufacturers must follow to certify their products. Compliance with these standards demonstrates a commitment to quality and reliability, enhancing the credibility of manufacturers in the eyes of consumers and partners alike.
In summary, taking quality control seriously in metal manufacturing can lead to improved product quality, operational efficiency, and a solid reputation in the market. The testing methods like tensile and hardness testing, coupled with strict adherence to standards, form a powerful triad that propels the manufacturing industry toward excellence.
Environmental Considerations in Metal Manufacturing
As the world continues to grapple with environmental degradation and climate change, the manufacturing sector, particularly metal manufacturing, finds itself under a microscope. This aspect of the industry is crucial due to the heavy resource consumption and waste generation associated with metal production processes. It's not just a question of production efficiency; it’s about sustainability and responsibility towards our planet.
Sustainability Challenges
The metal manufacturing industry faces significant sustainability challenges. Firstly, resource depletion is a pressing issue. Metals like aluminum and copper are finite resources that require diligent management. Mining activities disturb ecosystems, consume enormous amounts of water, and contribute to pollution when managed poorly. Furthermore, energy consumption in metal production is notably high. Traditional methods often rely on fossil fuels, further amplifying the carbon footprint of the industry.
Secondly, there is the problem of emissions. During operations like smelting, considerable greenhouse gases are released into the atmosphere. This not only contributes to climate change but also affects air quality in surrounding regions, posing a health risk to local communities. Finally, there’s the societal aspect of sustainability. Communities dependent on these industries often grapple with the trade-offs of economic growth versus environmental integrity. Balancing these elements demands innovative approaches and technologies that lead the way towards more sustainable practices.
Waste Management Practices
Effective waste management is essential in addressing the environmentally detrimental aspects of metal manufacturing. To begin with, recycling is one of the best practices. The recycling of metals not only reduces the amount of waste generated but also conserves raw material resources and energy. For instance, recycling aluminum saves up to 95% of the energy needed to produce it from bauxite ore. Therefore, implementing robust recycling programs is essential for mitigating environmental impact.
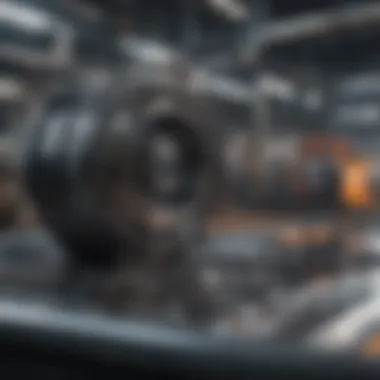
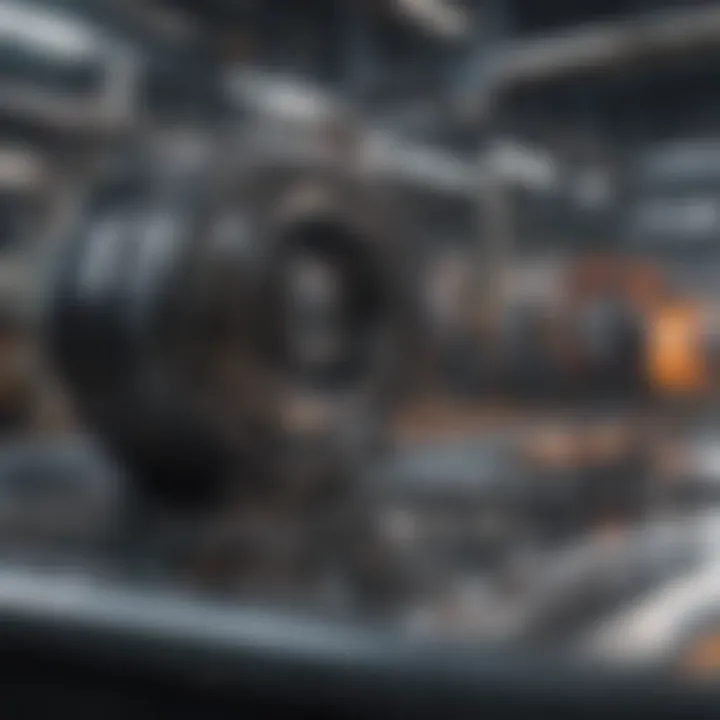
Secondly, organizations must adopt circular economy principles. This entails designing processes that minimize waste and maximize resource recovery. Companies are increasingly exploring methods for reusing scrap metal in production, thereby closing the loop on resource consumption.
Lastly, proper disposal practices for hazardous waste are crucial. Heavy metals and toxic materials often leftover from manufacturing processes can contaminate soil and water if not managed correctly. Establishing stringent regulations and ongoing training around hazardous waste management is essential for the workforce.
"The approach a company takes toward waste management can either mitigate or exacerbate environmental issues. It’s a critical aspect for today's metal manufacturers."
Future Trends in Metal Manufacturing
The landscape of metal manufacturing is continually evolving, influenced by technological innovation and a growing need for sustainability. Understanding the future trends in this industry is not merely an exercise in forecasting; it offers critical insights into how businesses can adapt and prosper. As the world embraces new technologies and shifts towards greener practices, metal manufacturing faces both challenges and opportunities.
Automation and Robotics
One of the most significant shifts in metal manufacturing is the integration of automation and robotics. These technologies are transforming how metal products are made, leading to increased efficiency and reduced production costs. Automation allows for the speedy and precise execution of repetitive tasks, minimizing human error and enhancing product quality.
Moreover, robots can work in environments that may be hazardous for humans, such as high-temperature areas or those with toxic materials. This not only safeguards human workers but also optimizes workflow.
The implementation of these systems isn’t without its challenges, though. Initial setup costs can be steep, and there's a constant need for ongoing maintenance and updates. However, the long-term benefits often outweigh these initial hurdles. Companies that adopt automation stand to gain a significant competitive advantage, fostering a trend towards smarter manufacturing practices.
Innovative Material Science Advances
The second pillar of future trends in metal manufacturing lies in innovative material science. Recent advancements in this field have led to exciting developments that impact not only how metals are produced but also how they perform.
Biomaterials
Biomaterials are emerging as a compelling solution to various challenges in metal manufacturing. These materials, derived from living entities, offer a unique alternative to traditional metals. One of their most appealing traits is their biocompatibility, making them highly favorable in medical applications, such as implants or prosthetics. The key characteristic of biomaterials is their ability to integrate into biological systems seamlessly, which makes them a popular choice in modern manufacturing.
A unique feature of biomaterials is their ability to be designed to degrade naturally, reducing waste and environmental impact in manufacturing processes. However, they can also present disadvantages, notably in terms of their physical properties. Many biomaterials may not have the same mechanical strength as traditional metals, which can limit their application in some industrial contexts. Despite this, the move toward sustainability makes biomaterials a vital subject of study in future manufacturing considerations.
Nanomaterials
Another vital aspect of material science is the rise of nanomaterials. These materials are engineered at the nanoscale, offering significant improvements in performance. Their key characteristic is their high surface area-to-volume ratio, which enhances reactivity and strength. Nanomaterials are increasingly utilized for their excellent properties, such as lightweight strength combined with enhanced conductivity and durability.
A distinct feature of nanomaterials is their potential to transform traditional metal products into multifunctional materials that can sense environmental changes or self-repair. While they present various advantages in terms of strength and adaptivity, drawbacks often include the complexity of manufacturing processes and the need for stringent safety evaluations due to their novel properties.
"The future belongs to those who believe in the beauty of their dreams." - Eleanor Roosevelt
By staying informed about these advancements, organizations can better prepare themselves for the shifts ahead, ensuring they are not only relevant but also leaders in a rapidly changing field.
Impact of Global Demand on Metal Manufacturing
The impact of global demand on metal manufacturing serves as a critical component of the intricate narrative surrounding the industry. As nations continue to develop and global economies expand, the demand for metals takes on new shapes and forms. The interplay between the economy and metal production isn't just a side note; it shapes the processes, influences technological advancements, and drives innovation within the sector.
Market Dynamics
In understanding the dynamics of the market, it’s important to recognize that the consumption of metals is largely driven by various sectors such as construction, automotive, and electronics. These industries have shifting demands based on economic cycles, technological evolutions, and consumer preferences.
- Demand Fluctuations: For instance, during economic booms, construction projects surge and thus increase the demand for steel and aluminum. Conversely, in recessionary periods, these needs tend to dwindle, causing manufacturers to adapt swiftly to new market realities.
- Price Sensitivity: The prices of raw materials can skyrocket or plummet based on global demand. When demand outpaces supply, as seen recently with lithium for batteries in electric vehicles, prices surge, encouraging investment in mining and refining operations.
- Global Supply Chains: The interconnectivity of global supply chains also plays a vital role. Supply disruptions—such as those seen during health crises or geopolitical tensions—can create gaps that ripple through markets, forcing manufacturers to reassess and innovate their approaches.
Trade Policies and Regulations
Trade policies and regulations can either bolster or hinder the flow of metals across borders, which in turn affects the overall landscape of manufacturing. When nations establish tariffs or quotas, they can unintentionally create bottlenecks that impact the availability of materials.
- Tariffs and Duties: For instance, a country imposing steep tariffs on imported steel can lead domestic manufacturers to raise prices in response to lower competition. This scenario can force them to shift focus to alternative materials or innovate their product lines.
- Bi-lateral Agreements: On the flip side, trade agreements that reduce tariffs can facilitate easier access to essential raw materials, strengthening the manufacturing base and encouraging competitiveness on a global scale.
- Regulatory Compliance: Furthermore, regulations related to environmental sustainability are becoming more stringent. Manufacturers must adapt their practices to not only comply with the law but also meet growing consumer expectations for eco-friendly operations and products.
"The understanding of global demand is not just about numbers; it's about predicting future trends and aligning strategies to accommodate change."
In summary, the impact of global demand on metal manufacturing underscores a landscape marked by ongoing shifts and changes driven by economic forces, activities in various industrial sectors, and regulatory environments. Recognizing and navigating these complexities is vital for stakeholders aiming to sustain and grow within the metal production ecosystem.
Human Resources in Metal Manufacturing
The significance of human resources in metal manufacturing cannot be overstated. People are at the heart of this complex industry, blending their skills with technology to create products that drive economies. Skilled workers are fundamental for navigating challenges that arise in processes ranging from extraction to refinement. Without a competent workforce, even the most sophisticated technology is rendered ineffective.
Human resources development is not just about filling positions; it’s about nurturing talent, ensuring safety, and fostering innovation. Effective training programs enhance skill sets, allowing workers to adapt to the swiftly changing landscape of metal manufacturing. As the industry evolves with advancements like automation and additive manufacturing, keeping the workforce well-informed and trained is paramount.
"The greatest asset of a company is its people."
This quote holds true, especially in an intricate field like metal manufacturing, where teamwork and collaboration often lead to groundbreaking outcomes. Moreover, investing in human capital helps in achieving operational efficiency and improves product quality, ultimately benefiting the stakeholders involved.
Skills and Training
In the realm of metal manufacturing, skills and training pave the way for success. The diverse tasks required—from machining to welding—demand specific competencies, which can only be acquired through rigorous training programs. These programs enhance technical knowledge and also bolster soft skills, like teamwork and problem-solving abilities.
Here are some key areas of focus in skills development:
- Technical Proficiency: Workers must be adept in operating complex machinery and understanding the nuances of metallurgy.
- Safety Training: Given the hazardous nature of the industry, ensuring that workers are aware of safety protocols is critical. A culture of safety can significantly reduce workplace accidents.
- Continuous Learning: The nature of metal manufacturing is changing rapidly, with new technologies emerging constantly. Ongoing education ensures that workers stay ahead of the curve, adapting swiftly to innovations like 3D printing and robotics.
Manufacturers often collaborate with educational institutions to tailor training programs that address current industry needs. This not only fills the skill gaps but also helps in creating a pipeline of qualified candidates ready to step into roles that are increasingly demanding.
Workforce Diversity and Inclusion
Creating a diverse and inclusive workforce in metal manufacturing isn't just a moral obligation; it’s a business imperative. A variety of perspectives fosters creativity and drives innovation, qualities essential to succeed in today's competitive market.
Diversity in the workforce can enhance problem-solving capabilities. Different life experiences lead to varied approaches in tackling challenges that arise in production and process improvement. Inclusive environments also promote employee satisfaction, which ultimately translates into higher retention rates and improved productivity.
Some considerations for promoting workforce diversity include:
- Recruitment Strategies: Actively seeking candidates from underrepresented groups helps in broadening the talent pool and supports equitable representation.
- Inclusive Workplace Culture: Implementing practices that recognize and value contributions from individuals of diverse backgrounds creates an environment where everyone feels empowered to share their ideas.
- Mentorship Programs: Developing mentorship initiatives can support the growth and advancement of diverse employees, ensuring that everyone has equal opportunity for success.
By recognizing the value of both skills training and diversity, metal manufacturing can transform challenges into opportunities, leading to a robust and dynamic workforce capable of innovating for the future.