Understanding the Roles of Immune Organs in Health
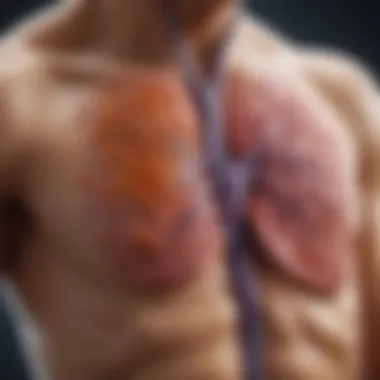
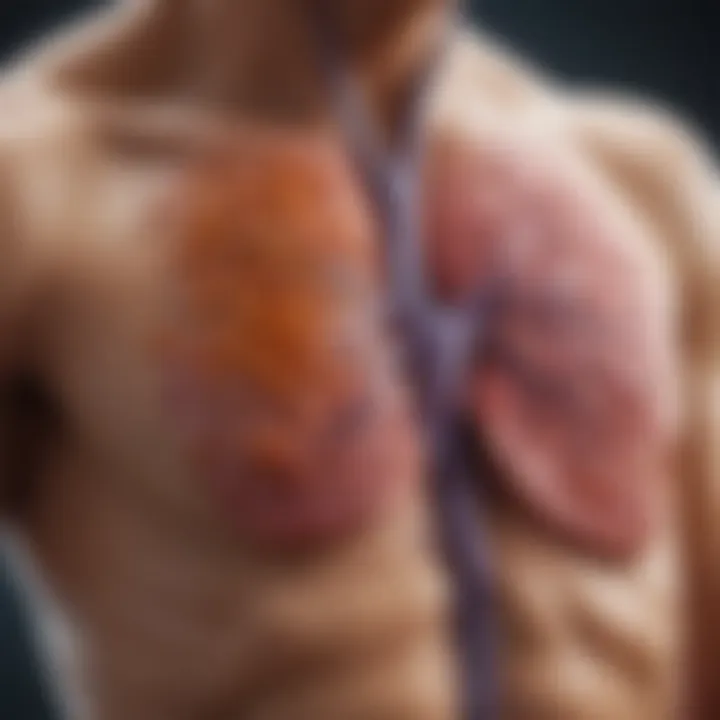
Intro
Among the key players, the thymus, bone marrow, spleen, and lymph nodes take center stage. Each organ possesses unique structural attributes and functions, yet they form a symbiotic relationship crucial for effective immune surveillance and response. The standout feature is how these organs, while performing their specific duties, communicate and collaborate, ensuring a robust defense against disease.
Understanding this functionality and interconnectivity necessitates a thorough examination, not only of individual organs but also of how they interact with each other. It is this interaction that informs recent research findings, which have painted a richer picture of the immune system's workings in health and disease. The subsequent sections will delve into fundamental research insights, the experimental processes involved, and significant observations that contribute to a more nuanced understanding of this essential system.
Overview of the Immune System
Understanding the immune system is like peering through a complex maze that connects various components, each with its own unique role. For this article, we delve into the intricate relationship between immune organs and their collective contribution to protecting the body. Everything from the minute cellular interactions to the sophisticated filtration systems of organs plays a key role in maintaining health and fighting against diseases. By grasping the functionalities of these organs, one gets a better picture of how our body stays resilient against pathogens and other harmful agents, emphasizing the vital importance of diligent immune responses in a constantly changing environment.
Definition and Importance
The immune system is often likened to a fortress, with numerous layers and defenses prepared to fend off invaders. At its core, it consists of organs, cells, and proteins that harmonize together to identify and neutralize harmful substances. Without this intricate coordination, our body would be vulnerable to infections, leading to serious health complications.
Understanding the immune system is paramount not just for medical professionals but also for researchers and educators. The knowledge gleaned from immunology extends well into various fields, from developing vaccines that boost immune responses to understanding autoimmune diseases where the body attacks itself. Education surrounding these aspects shapes public health decisions and medical advancements.
Components of the Immune System
Recognizing the various components that comprise the immune system is crucial for grasping its functionality. These components can be classified into:
- Primary Immune Organs: Which include the bone marrow and thymus, play a fundamental role in developing immune cells. These organs serve as the birthplace for lymphocytes, the white blood cells that are integral to immune responses.
- Secondary Immune Organs: These organs, such as lymph nodes, spleen, and mucosa-associated lymphoid tissues (MALT), provide the environment for immune reactions to occur and facilitate communication between different cellular components of the immune system.
The interplay between these organs ensures a rapid and effective response to potential threats, bringing together all the elements needed to protect the body.
"The interconnectedness of these organs illustrates a symphony of defense mechanisms that safeguard our well-being."
In summary, comprehending the immune system's overview gives insight into its critical functions. It paints a clear picture of why every layer, every component matters in maintaining overall health, providing a prelude to the more detailed examination of individual immune organs that follows in this article.
Primary Immune Organs
The role of primary immune organs is a cornerstone in understanding how our body defends itself against diseases. This section dives into the bone marrow and thymus, the two principal organs responsible for the development of immune cells. These organs not only have specialized structures but also perform critical functions necessary for immune responses. Each supports the body in building a competent defense system capable of responding to pathogens.
Bone Marrow
Structure and Function
The bone marrow, often likened to a bustling city, is majorly composed of hematopoietic stem cells encased in a supportive stroma. This unique structure is essential because it provides the environment and signals that stem cells need to differentiate. The honeycomb-like architecture facilitates the easy movement of the newly formed cells into circulation.
A notable function of bone marrow is its ability to produce both red and white blood cells, with a particular focus on providing lymphocytes, the key players in adaptive immunity. The interconnectivity with the bloodstream allows for immediate dissemination of these cells throughout the body, enhancing the speed of the immune response.
Hematopoiesis Role
Hematopoiesis, the process of blood cell formation, takes precedence in the bone marrow. This is where the journey truly begins—stem cells evolve into various types of blood cells. Importantly, this process is not merely functional but is finely tuned, dependent on signals like cytokines and growth factors.
The key characteristic here lies in its dynamic nature, allowing the body to respond to the demand for white blood cells during infections. With the ability to ramp up production when needed, bone marrow showcases its adaptability, making it an exemplary model of biological optimization.
Interaction with Other Immune Organs
The collaboration of bone marrow with other immune organs illustrates the deeply ingrained connections within the immune system. For instance, the thymus relies on T cells that have developed in the bone marrow before maturing. This interaction underscores a vital aspect: immune organs do not operate in isolation but rather in a cohesive network, each providing support to the other.
The constant communication between these organs means that the body can maintain a balance. Without such interactions, the immune response could either be too weak or overly aggressive, leading to potential diseases.
Thymus
Anatomy of the Thymus
The thymus is another primary organ that is structured not only for physical presence but for maximum efficacy. Shaped somewhat like a butterfly, this organ is found in the upper chest, nestled under the sternum. Its distinct compartments contribute to its functionality; with the outer cortex housing immature T cells and the inner medulla facilitating their maturation.
This organ stands out primarily because of its significant role in shaping the repertoire of T cells. These cells are integral to the immune system, making the thymus a linchpin in ensuring a robust defense mechanism is in place.
T Cell Maturation
T cell maturation is a remarkably sophisticated process that occurs within the thymus. This process involves both positive and negative selection, ensuring that only those T cells that are properly responsive to pathogens and non-self antigens succeed. The complex signaling environment present in the thymus acts like a boot camp, honing the skills of T cells to differentiate between friend and foe.
Highlighting T cell maturation’s importance, one can understand that any aberration can lead to severe consequences, including autoimmunity or immunodeficiency. Thus, the maturation of T cells inside the thymus validates its crucial function in overall immune health.
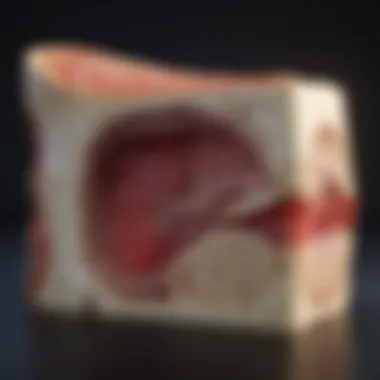
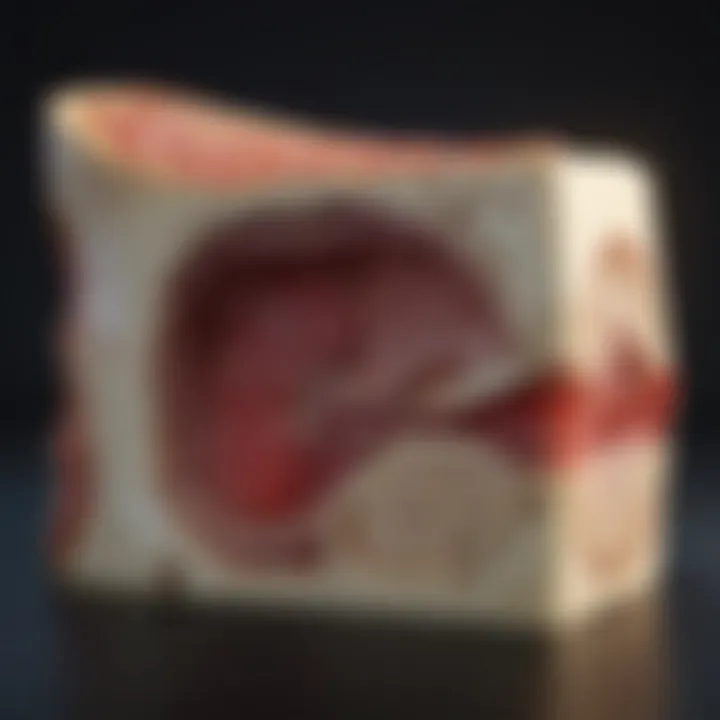
Thymus in Disease
The significance of the thymus extends into various medical conditions. Thymic atrophy can occur with age or as a result of certain diseases, leading to a decline in T cell numbers and functionality. Conditions such as myasthenia gravis are directly associated with thymic issues, reinforcing the idea that the thymus is not just an anatomical structure but a critical player in immunological diseases.
Understanding the impacts of the thymus in disease leads to greater insight into therapeutic approaches. For instance, strategies aimed at enhancing thymic function in older adults or post-transplant patients can have profound implications for improving immune responses.
Secondary Immune Organs
Secondary immune organs hold a pivotal role in the immune system, acting as critical sites for immune responses and interactions. Unlike primary organs, which are responsible for the production and maturation of immune cells, secondary organs serve as the battlegrounds where these cells encounter pathogens and respond to threats. Their structural diversity, such as lymph nodes, spleen, and mucosa-associated lymphoid tissue (MALT), allows them to fulfill distinct but interconnected functionalities. Not only do they facilitate communication among different immune cells, but they also help to filter out harmful substances and activate the immune system effectively.
Lymph Nodes
Structure and Types of Lymph Nodes
The lymph nodes are small, bean-shaped organs scattered throughout the body, linked to the lymphatic system. One key characteristic is their organization into distinct regions: the cortex, paracortex, and medulla. This structure supports various types of immune cells, including lymphocytes and dendritic cells, allowing for specialized immune functions.
What makes lymph nodes particularly fascinating is their ability to act as gateways for immune cell traffic. When pathogens invade, these nodes swell, indicating immune activity as they trap and destroy foreign substances. This feature is beneficial because it ensures that the immune response is rapid and localized. Their segmentation means different types of immune responses can occur simultaneously, but this complexity can also lead to misunderstandings about how each type operates during infections.
Function in Immune Response
Lymph nodes are crucial to the immune response because they serve as meeting points for immune cells. When pathogens or other antigens are detected, dendritic cells transport them to the lymph nodes, activating naive T and B cells. A major benefit of this functionality is that these nodes allow the immune system to mount a targeted response rather than a broad, indiscriminate attack.
However, this concentrated environment can be a double-edged sword. While it enables a quick response, it also means that infections can sometimes leap from one lymph node to another, complicating treatment strategies. Understanding the specific immune functions within these nodes can clarify why certain pathogens evade immune detection.
Role in Cancer Metastasis
The role of lymph nodes extends beyond infection fighting; they are also critical players in cancer metastasis. Cancer cells can spread to lymph nodes, using them as way stations on their journey to distant sites. The lymphatic system's structure facilitates this movement, making it easier for cancer cells to establish themselves in new areas.
A critical aspect of this is that lymph nodes can sometimes become the first point of metastasis, raising the importance of targeted therapies in these regions. Therefore, while lymph nodes ensure immune protection, their involvement in cancer dynamics presents significant challenges, especially in treatment approaches and understanding disease progression.
Spleen
Anatomy of the Spleen
The spleen is another vital secondary immune organ, located in the upper left abdomen. Its distinctive structure consists of red and white pulp. The red pulp helps in the filtration of blood, while the white pulp is rich in lymphoid tissue, providing a site for immune cell activation.
A defining feature of the spleen is its ability to filter out damaged or aged red blood cells. This function underlines its importance in not only immune surveillance but also maintaining healthy blood composition. However, while the spleen holds many advantages in cleansing the bloodstream, its loss can lead to increased susceptibility to infections due to a reduced ability to respond to pathogens in the blood.
Functions in Blood Filtration
The spleen's filtration capabilities are remarkable. It removes senescent red blood cells while recycling iron and other components for new cell production. This process is vital for maintaining a healthy blood supply and preventing anemia, which can result from ineffective filtration.
Additionally, the spleen serves as a reservoir for blood. In times of increased demand, such as during physical exertion or bleeding, it can release stored blood into circulation. Although beneficial, this extraordinary blood filtration and retrieval function can become a disadvantage when it underlies serious conditions, such as splenic rupture.
Immunological Role
Immunologically, the spleen plays a dual role. Not only does it respond to blood-borne pathogens, but it also produces antibodies. Its unique architecture supports the interaction of B and T cells, making it a hub for mounting effective immune responses. The ability to host immune cell proliferation enhances its value as an immune organ.
However, the reliance on the spleen for managing blood-related immune responses means that individuals without a functional spleen may face increased risk of infections, highlighting how the organ integrates with overall health and disease prevention efforts.
Mucosa-associated Lymphoid Tissue (MALT)
Structure and Locations
MALT comprises a significant portion of the immune system, found in the mucosal surfaces of the body, such as the gut (GALT), respiratory tract (BALT), and even the urogenital tract. Its structure varies from isolated lymphoid follicles to larger aggregates, allowing flexibility to combat pathogens invading through mucosa.
A striking characteristic of MALT is its ability to sample antigens directly from mucosal surfaces. Thus, it can mount immune responses locally, providing tailored defense aligned with where invasion occurs. However, this localized response can sometimes overlook potential systemic ramifications if not supported by other immune structures.
Role in Mucosal Immunity
MALT plays a pivotal role in mucosal immunity, acting as the first line of defense against ingested or inhaled pathogens. By producing secretory IgA, a specific antibody, it helps to prevent the colonization of pathogens at mucosal surfaces.
What stands out about its role is the enhancement of tolerance toward non-pathogenic antigens, such as food proteins, allowing for integration with daily life without provoking unnecessary immune responses. However, this same tolerance can present challenges—if the balance tips, conditions like allergies may arise.
Impact on Infectious Diseases
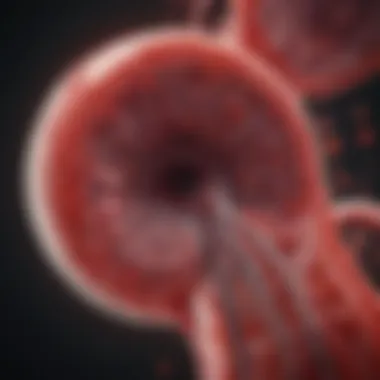
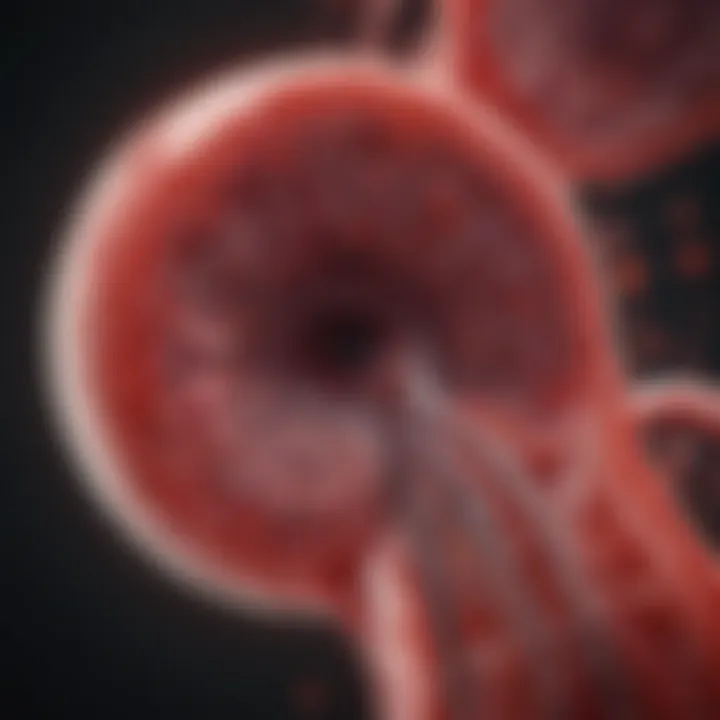
The impact of MALT on infectious diseases is profound. It acts as a rapid responder to invasive pathogens while maintaining a level of homeostasis that minimizes unnecessary inflammation. This ability gives individuals critical protection, particularly against intestinal infections.
Nonetheless, the diverse locations of MALT mean that specific infections might bypass these defenses. Moreover, the dynamics of MALT’s response can lead to variability in immunity among populations, making it an interesting area for further research into vaccine development and mucosal treatments.
Immune Responses and the Role of Immune Organs
Understanding the immune responses and their interaction with various organs is essential for grasping the complexities of our body's defense mechanisms. Immune responses are the actions our body takes in reaction to pathogens, such as bacteria, viruses, and other foreign invaders. The organs involved in these responses not only house immune cells but also facilitate their communication and cooperation, forming a complex network that is crucial for maintaining health.
Immune organs serve as command centers, where specific responses are initiated based on the type of threat faced. Without this intricate system of coordination, our defense strategies would be chaotic, diminishing the effectiveness of our immune response. Thus, the interplay among immune organs is vital for recognizing and neutralizing threats, while ensuring that the body doesn’t unjustly attack itself.
"An understanding of immune responses and the connectedness of immune organs is where effective immunological science begins."
Innate Immunity
Innate immunity is the first line of defense, characterized by a rapid response to infection. This system comprises various physical barriers, such as skin and mucous membranes, as well as immune cells that confront pathogens on sight. Key players in this realm are macrophages, neutrophils, and dendritic cells, often found in various immune organs. For instance, bone marrow produces and releases these cells, while the thymus plays a pivotal role in T-cell maturation for this broad-spectrum initial response.
Some hallmark features of innate immunity include:
- Immediate Response: Innate immunity kicks in within hours of pathogen detection, acting faster than adaptive immunity.
- Broad Specificity: It recognizes common features of pathogens, not specific strains or variants.
- Minimal Memory: Once the threat is neutralized, innate immune responses do not remember the pathogen for future encounters.
In summary, innate immunity relies on quick actions and a wide range of tools, laying the groundwork for the more elaborate adaptive immune system that follows.
Adaptive Immunity
Adaptive immunity is the body's tailored defense against specific pathogens. This system takes longer to mobilize but is highly effective and remembers previous encounters with pathogens, thanks to a sophisticated strategy. The key components of adaptive immunity include B cells and T cells. Unlike innate immunity, adaptive immunity relies heavily on the interaction between various immune organs, such as the thymus, lymph nodes, and spleen.
Important aspects of adaptive immunity include:
- Specificity: Each pathogen triggers a specific response, leading to the production of unique antibodies tailored for that pathogen.
- Memory Formation: After an infection, memory cells persist, providing long-lasting protection against future infections of the same pathogen.
- Slower Response: Adaptive immunity may take days to weeks to develop fully, as it involves recognizing foreign materials and generating a targeted response, often mediated by the lymph nodes and spleen.
The effectiveness of adaptive immunity hinges on the interconnectivity of immune organs, as they continually communicate and adapt to efficiently fend off threats. Organ systems work in concert, ensuring that responses are not only reactive but also preventative in helping mitigate the risks of future infections.
Interactions Among Immune Organs
The immune system is not an isolated army defending the body; rather, it functions as a finely tuned orchestra where each section—each immune organ—plays a critical role in maintaining harmony. Understanding the interactions among immune organs provides profound insights into how the entire system operates efficiently. This interconnectedness allows for swift responses to pathogens, ensures proper immune development, and maintains homeostasis. Additionally, knowing how these organs communicate informs advancements in medical treatments, including vaccines and immunotherapies, showcasing the relevance of these intricate collaborations.
Cellular Communication
Cytokines and Chemokines
Cytokines and chemokines serve as the messengers in this cellular communication ballet. These signaling molecules are crucial in orchestrating an effective immune response. Cytokines are proteins that regulate immunity, inflammation, and hematopoiesis. Chemokines, a subset of cytokines, are primarily responsible for directing the movement of immune cells towards sites where they are needed, such as areas of infection or tissue damage. They are like street signs guiding white blood cells to their destinations.
The key characteristic of these molecules is their nature of rapid and localized action, making them a popular choice for understanding immune dynamics. One unique feature is their capacity to enact both autocrine and paracrine signaling—where they act on the cells that produce them or neighboring cells, respectively. This dual functionality creates a finely tuned response system. However, it can also lead to complications. For instance, if cytokine levels become too elevated, it can result in cytokine storms, which are detrimental rather than protective. This potential downside underlines the importance of balance in signaling.
Cell Signaling Pathways
Cell signaling pathways are vital in translating the messages sent by cytokines and chemokines into cellular responses. When immune cells encounter pathogens, specific receptors on their surfaces bind to these signaling molecules, triggering a cascade of intracellular events. This intricate choreography ultimately directs the cell towards proliferation, differentiation, or apoptosis—ensuring that the right immune cells are activated at the right time.
The essential characteristic of these pathways lies in their complexity and specificity; different signals can lead to varied responses based on the cell type and the context of the interaction. This specificity makes it a valuable focus in our discussions about immune organ functionality because understanding these pathways can lead to targeted therapies. However, the challenge is that these pathways can become dysregulated, potentially leading to autoimmune diseases or malignancies. Thus, balancing their activity is crucial for maintaining immune health.
Network of Immune Response
Cooperation during Infections
The cooperation among immune organs during infections is nothing short of remarkable. When a pathogen breaches first-line defenses, the immune response quickly ramps up, involving multiple organs working in concert. Lymph nodes act as gathering hubs, where T and B cells are activated, while the spleen filters the blood for pathogens and helps produce antibodies. This coordinated effort enables rapid and effective clearance of infections, highlighting the powerful advantages of interconnected immune function.
The unique feature of this cooperation is its adaptability. The immune response can pivot based on the pathogen's nature. For instance, a viral infection might elicit a different response than a bacterial one, showcasing the versatility of this network. However, this also implies potential pitfalls; the miscommunication or delayed activation among organs can lead to insufficient immune responses, exacerbating infections.
Adaptations in Aging
As age creeps in, so does a noticeable shift in immune functionality. Many studies reveal a decrease in the efficiency of immune organs—thymic involution, for example, reduces T cell production, making older individuals more susceptible to infections. These adaptations in aging reveal how the interactions among immune organs might alter, emphasizing the importance of continuous research in this area.
The key characteristic here is the gradual decline in immune robustness, which necessitates a deeper dive into the implications of immune organ interconnectivity as we age. The unique feature of these adaptations lies in the body’s attempts to compensate, perhaps by increasing activity in other organs like the spleen or lymph nodes. But these compensatory mechanisms may not be enough, potentially leading to increased morbidity in older populations. Such findings underline the importance of understanding these interaction dynamics, as they pave the way for developing targeted interventions to enhance immune health in aging individuals.
The collaboration among immune organs showcases the complexity and adaptability of the immune system, an interplay that highlights the importance of understanding their interconnectivity to improve health outcomes.
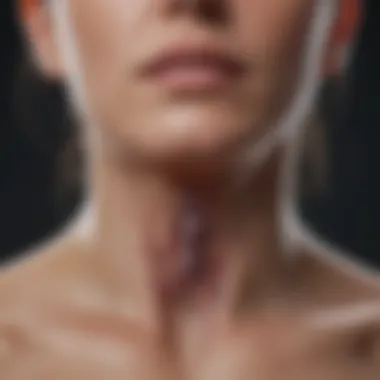
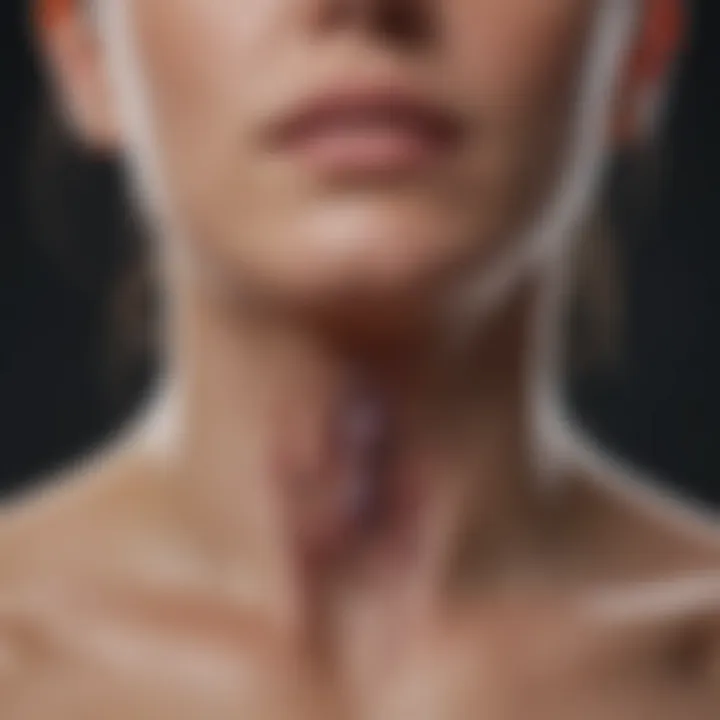
Pathologies Associated with Immune Organs
The examination of pathologies associated with immune organs is crucial for advancing our understanding of immune system functionality. Immune pathologies can have profound effects, not only on individual health but also on the development of treatments and therapies across various diseases. By delving into the dysfunctions of immune organs, we gain insights that can pave the way for better diagnostic tools, targeted therapies, and potentially new preventive measures. This section looks at two significant categories of immune-related diseases: autoimmune disorders and immunodeficiency conditions, with each presenting unique challenges and implications for health care.
Autoimmune Disorders
Autoimmune disorders occur when the immune system mistakenly identifies the body's own tissues as foreign, launching an unwarranted attack against them. This misfire can lead to chronic inflammation and damage across numerous organ systems—a wide array of conditions often contrasts significantly in their presentation and severity. Examples of autoimmune diseases include rheumatoid arthritis, lupus, and multiple sclerosis.
The complexity of autoimmune disorders often stems from a combination of genetic predisposition and environmental triggers. The thymus gland plays a pivotal role in the maturation of T-cells, helping to educate them on distinguishing self from non-self. Any disruption in this process could lead to harmful consequences.
- Key Elements of Autoimmune Disorders:
- Diverse Pathways: Different autoimmune diseases may involve multiple immune pathways, complicating diagnosis and treatment.
- Management Approaches: Treatment modalities often focus on dampening the immune response or re-establishing tolerance. Medications such as corticosteroids and immunosuppressants are commonly used, but the risk of infection remains a significant consideration.
- Research Insights: Recent studies emphasize the role of the gut microbiome in modulating autoimmune diseases. Investigating this connection can offer new avenues for intervention.
"Understanding the cellular communication involved in autoimmune responses is key to unlocking novel treatment strategies."
Immunodeficiency Conditions
Immunodeficiency conditions manifest when one or more components of the immune system are absent or dysfunctional, rendering individuals vulnerable to infections and illnesses that a healthy immune system would typically combat effectively. These can be primary, resulting from inherent genetic defects, or secondary, resulting from external factors such as infections (like HIV), malnutrition, or certain medications.
In cases of primary immunodeficiency, the role of the bone marrow in producing adequate immune cells becomes evident. Such conditions might necessitate treatments like bone marrow transplants or immunoglobulin therapy to restore immune function.
- Types of Immunodeficiency Conditions:
- Primary Immunodeficiencies: Genetic disorders such as Severe Combined Immunodeficiency (SCID)
- Secondary Immunodeficiencies: Conditions acquired through exposure to external agents, like chemotherapy.
- Epidemiology: Understanding the prevalence and risk factors associated with these conditions can improve health resources and support networks.
Research in this field is progressing rapidly. Genetic screening and innovative therapies, including gene editing technologies like CRISPR, hold promise for correcting genetic defects responsible for primary immunodeficiencies.
Addressing these pathologies not only highlights the critical roles immune organs play but also underscores the need for ongoing research and development in immunology. As such, we continue to evolve our understanding of how immune organs impact health and disease.
Research Frontiers in Immune Organ Studies
The exploration of immune organs has taken a significant leap in recent years, drawing attention not only because of their fundamental role in health but also due to the complexities associated with various diseases. The study of these organs is continuously evolving, with cutting-edge research illustrating promising insights into their functionality and interconnectivity. The vital nature of understanding these components is underscored by emerging patterns in disease manifestations, immune therapies, and chronic illness pathways. This section delves into the fresh terrain of research in immune organs, focusing on emerging technologies and future directions that shape our understanding.
Emerging Technologies
As advancements in technology burgeon, the intersection of such innovations with immunology has opened doors for enhanced exploration and understanding of immune organs. Current technologies allow for unprecedented precision in studying these organs, making it possible to probe their intricate environments . For instance:
- Single-cell sequencing: This technology aids in comprehensively profiling immune cell populations within organs like the thymus or spleen, offering detailed insight into the diversity and functionality of immune cells.
- Immunoassays: These tests help determine the presence and quantity of immune-related proteins in tissues, leading to a better grasp of how these organs respond during various challenges.
- In vivo imaging: Techniques like two-photon microscopy visualize immune organ dynamics over time, revealing real-time interactions between various immune cells.
These tools not only enhance our knowledge but also pave paths for therapies that can effectively target immune organs' functions. For example, targeting cell migration patterns seen in lymphatic systems could inform treatment strategies for lymphatic diseases.
Future Directions in Immunology Research
The future of immunology research is ripe with possibilities, particularly concerning the interconnectivity and roles of immune organs. A few intriguing directions include:
- Personalized medicine: With the understanding that each individual's immune response is unique, research is steering toward tailored treatments based on specific immune organ profiles. This concept aims to revolutionize how conditions like autoimmune diseases and allergies are approached.
- Molecular mechanisms: Deeper investigation into the molecular interplay between various immune organs might unravel how chronic inflammation or autoimmunity develops. Identifying triggers and metabolic pathways could lead to groundbreaking prevention strategies.
- Bioengineering: The potential for creating synthetic immune organ models holds promise for testing therapies and understanding immune responses in a controlled environment. Such bioengineered organs could provide key insights into organ-specific responses to infections or injuries.
- Integration with Artificial Intelligence: Leveraging AI for analyzing complex data obtained from immune organs could significantly enhance our understanding. The potential to predict immune responses based on organ interactivity is a game changer in both research and clinical settings.
"The dynamics of the immune system calls for a research approach that is as sophisticated as the system it investigates. Emerging technologies and innovative research directions can illuminate the road ahead in immunological studies."
As the field progresses, the insights gained from these studies may not only aid in understanding disease mechanisms but could also lead to the development of novel therapies that improve patient outcomes. Keeping a close watch on these developments is essential for students, researchers, educators, and professionals alike, as they pave the way for a new era in immune organ studies.
The End
The discussion has emphasized how primary organs—such as bone marrow and the thymus—serve as the cradle for immune cell development. Meanwhile, secondary organs like the spleen and lymph nodes act like airports for immune activities, facilitating communication among cells. Their interactions are vital; a breakdown in these could lead to autoimmune disorders or immunodeficiencies.
Summary of Key Points
- Comprehensive Overview: The immune system is intricately structured, comprising primary organs responsible for cell maturation and secondary organs specialized for immune responses.
- Significant Interconnectedness: Each immune organ plays a unique role but is interconnected, allowing for efficient communication that enhances the body’s defense mechanisms.
- Role in Health and Disease: Understanding these organs aids in deciphering complex diseases and designing better therapeutic strategies.
- Research Frontiers: Emerging technologies in immunology research open avenues for breakthroughs in handling diseases like cancer and autoimmune disorders.
"The immune system is a complex network, not just a sum of its parts."
Implications for Health and Medicine
The implications of a deeper knowledge of immune organs extend far beyond academic realms; they directly impact clinical practices and public health strategies. For instance, knowing how immune organs develop and function can lead to better vaccination protocols, as the effectiveness of vaccines relies on an intact immune system that recognizes and remembers pathogens.
Furthermore, this understanding is crucial for addressing public health challenges such as pandemics. Strategies can be devised to strengthen immune responses against emerging infectious agents. Also, with insights into the interplay of different immune organs, personalized medicine is becoming a reality, allowing treatments to cater based on individual immune profiles.
Challenges such as autoimmune disorders or immunodeficiencies can be tackled more effectively with this knowledge. By focusing on the interconnectivity of these organs, researchers can develop targeted therapies that restore balance to the immune response, paving the way for new treatment options.
Finally, this information aids scientists and healthcare professionals in anticipating and managing complications arising from organ malfunctions, ensuring a proactive, rather than reactive, approach to health care.