Exploring Promega T7 Technology in Molecular Biology
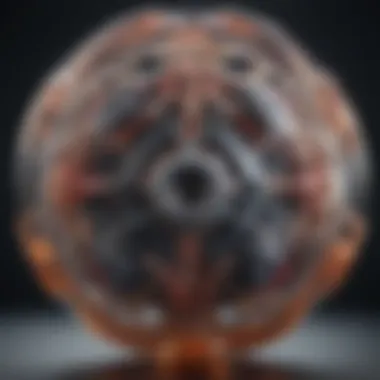
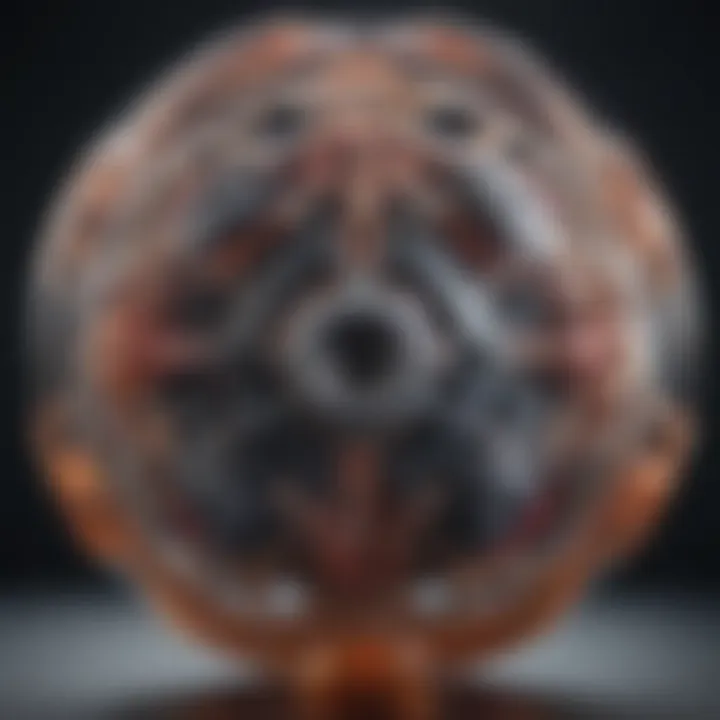
Intro
Promega T7 technology represents a significant advance in molecular biology, particularly in the areas of genetic engineering and biochemical research. Its applications span transcription and translation processes, making it a crucial tool for researchers. Understanding this technology not only reveals its mechanisms but also its broader implications in scientific research.
The use of T7 RNA polymerase, a bacteriophage enzyme, facilitates efficient transcription of DNA templates into RNA. Researchers utilize this system for various purposes, including the synthesis of RNA probes, mRNA for protein expression, and more. Highlighting the important features of Promega's specific systems helps to clarify the advantages they offer in these processes.
This article aims to traverse the landscape of Promega T7 technology, examining its operational stages, troubleshooting methods, and current research applications. By delving into this topic, students, researchers, educators, and professionals can gain a nuanced understanding of the relevance of this technology in advancing molecular biology.
Research Overview
Summary of Key Findings
Promega T7 technology has been employed effectively in multiple studies, yielding substantial findings. Research indicates that using T7 systems can enhance the yield and purity of RNA produced. This advantage is pivotal in applications such as vaccine development and gene therapy. Additionally, the system's flexibility facilitates adaptations to various experimental designs, making it a valuable tool across diverse research fields.
Research Objectives and Hypotheses
The primary objectives of this research involve:
- Examining the transcriptional efficiency of T7 RNA polymerase.
- Identifying optimal conditions for RNA synthesis.
- Comparing the Promega T7 systems with alternative technologies.
The hypothesis posits that Promega's T7 technology will demonstrate superior efficiency and yield in RNA production compared to other methods in specified experimental setups.
Methodology
Study Design and Approach
A comparative study design will be used, analyzing various T7 systems against other established methods. These methods include T3 and SP6 RNA polymerases, enabling a holistic understanding of their respective performances.
Data Collection Techniques
Data will be collected through a series of controlled experiments. Key techniques will include:
- Quantitative analysis of RNA yield.
- Qualitative assessments via gel electrophoresis.
- Statistical analysis of variance to establish the significance of the findings.
Understanding the mechanisms of Promega T7 technology helps elucidate its critical role in molecular biology and genetic engineering.
Prelims to Promega T7
Promega T7 technology stands at the intersection of molecular biology and genetic engineering, offering tools that form the backbone of various research initiatives. This section details the foundation of Promega T7, clarifying its importance, scope, and relevance in research settings. Understanding Promega T7 helps both new and experienced researchers grasp its utility in manipulating RNA and proteins, ultimately advancing their investigative goals.
Understanding T7 RNA Polymerase
The heart of Promega T7 technology lies in T7 RNA polymerase, a crucial enzyme originally derived from the T7 bacteriophage. This enzyme is specifically designed for synthesizing RNA from a DNA template. Its synthetic capacity is largely attributed to its high fidelity and efficient rate, enabling the rapid production of RNAs necessary for diverse downstream applications. Researchers exploit T7 RNA polymerase due to its capacity to produce significant amounts of RNA, facilitating experiments such as mRNA synthesis for protein expression, RNA interference studies, and more.
The ability to control transcription by specifying exact promoters allows for the targeted manipulation of gene expression. This level of control is especially valuable in synthetic biology where precise programming of genetic systems is required.
Historical Context and Development
To appreciate Promega T7 technology fully, itβs important to consider its historical backdrop. T7 RNA polymerase was first characterized in the late 1960s, a time marked by significant advancements in molecular biology techniques. The early work with T7 paved the way for various transcription systems leveraged in modern laboratories.
Promega Corporation embraced this technology and further developed it into commercially available kits and systems, aiming to streamline research applications. The advent of these systems democratized access to advanced transcription options, promoting innovation in molecular biology research.
Over the decades, enhancements to T7 systems have been made, leading to improved yields and efficiency. Researchers now enjoy a wealth of knowledge regarding the properties and best practices associated with T7 RNA polymerase, making this enzyme a staple in contemporary laboratories. By understanding its development, scientists can recognize how foundational concepts evolve into sophisticated tools that expedite research.β
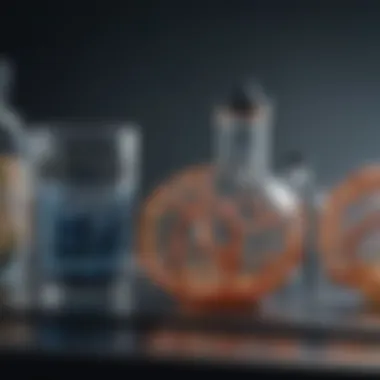
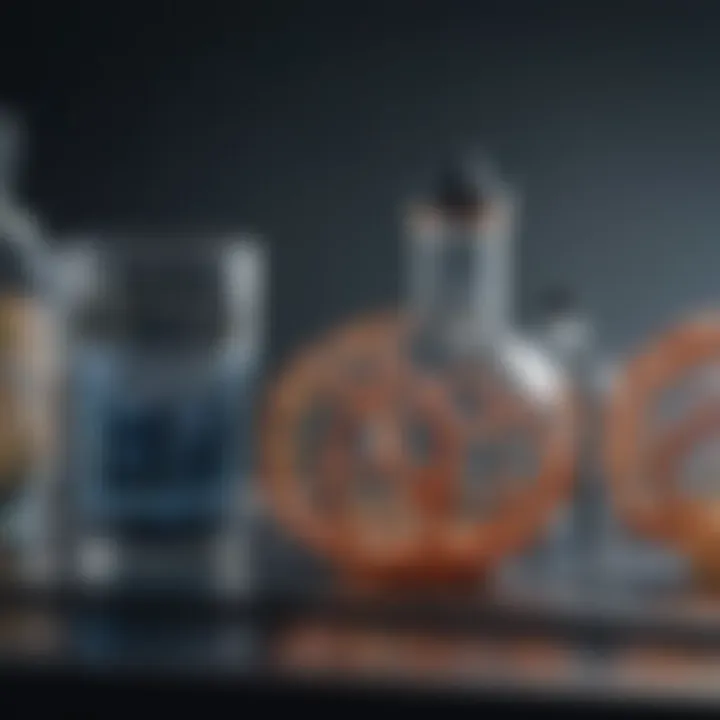
Mechanism of T7 Transcription
Understanding the mechanism of T7 transcription is crucial for grasping how Promega's T7 technology operates. This intricate process is the foundation upon which numerous applications in molecular biology rely. The T7 RNA polymerase catalyzes the synthesis of RNA from a DNA template. By explaining the stages of transcriptionβinitiation, elongation, and terminationβwe uncover how this technology can be harnessed effectively for research and development. The mechanism not only provides insights into transcription itself but also highlights the advantages of using T7 polymerase over other systems. It is essential for researchers to understand these stages to optimize their use of T7 technology in various applications.
Initiation of Transcription
The initiation phase is the first crucial step in the transcription process. During this phase, T7 RNA polymerase binds to a specific region called the promoter. The T7 promoter, which is a short stretch of nucleotides, is recognized by the T7 polymerase, allowing it to initiate the transcription process. This interaction is highly specific, as T7 polymerase is designed to work optimally with its own promoters.
Once the polymerase binds to the promoter, it forms a closed complex. This complex then undergoes a transition to an open complex, wherein the DNA strands separate around the promoter region, exposing the template strand. The enzyme then begins synthesizing RNA, starting with the first nucleotide. This phase is characterized by several factors:
- Specificity: The T7 polymerase requires its specific T7 promoter, ensuring precise transcription of target genes.
- Efficiency: The transition from closed to open complex happens rapidly, underscoring the efficiency of T7 polymerase in starting RNA synthesis.
- Control mechanisms: Various factors can influence the binding and initiation processes, affecting yields and purity in RNA production.
Elongation Phase
During the elongation phase, the RNA polymerase moves along the DNA template, synthesizing the RNA chain. As T7 RNA polymerase begins to elongate the RNA strand, it does so in a 5' to 3' direction. This phase is marked by several critical features:
- Speed: T7 polymerase is known for its rapid elongation rate, averaging about 1000 nucleotides per second, which is significantly faster than many other polymerases.
- Processivity: The ability of T7 RNA polymerase to remain attached to the DNA template without dissociating contributes to high RNA yield during transcription.
- Transcription fidelity: This phase also incorporates proofreading mechanisms. Though not as extensive as some DNA polymerases, T7 RNA polymerase has a relatively low error rate, ensuring accurate RNA production.
Termination of Transcription
The termination phase concludes the transcription process. This phase involves specific signals within the RNA sequence or structure that trigger the enzyme to stop RNA synthesis and release the newly formed RNA molecule. Key points to note include:
- Termination signals: In many systems, specific sequences in the RNA signals that transcription should end. In the case of T7, it often involves polyadenylation sequences.
- Release of RNA: Once termination occurs, RNA polymerase dissociates from the DNA, freeing the synthesized RNA for downstream applications such as translation or further manipulation.
- Implications for downstream processing: The quality and integrity of the RNA produced can be influenced by how effectively transcription is terminated. Proper termination ensures there are no residual RNA strands that could complicate later experiments.
Effective transcription termination is essential in obtaining pure RNA for subsequent procedures like cDNA synthesis or in vitro translations.
In summary, the mechanism of T7 transcription is fundamental to its applications in various fields of molecular biology. A clear understanding of each phase can significantly improve experimental outcomes and the effectiveness of using T7 systems.
Applications of Promega T7 Technology
Promega T7 technology has become integral in the fields of molecular biology and genetic engineering. Its applications across various areas underline its versatility and profound impact on research. This section explores the significance of Promega T7 technology, emphasizing some practical applications while considering benefits and critical factors influencing these areas of research.
In Vitro Transcription Systems
In vitro transcription systems utilizing Promega T7 technology are widely used to produce RNA. The T7 RNA polymerase enables high levels of transcription from DNA templates. One significant benefit of this system is its ability to generate large quantities of RNA quickly and efficiently. This feature is crucial in situations where time and volume are essential, such as when producing RNA for functional studies or assays.
Additionally, in vitro transcription systems help researchers create specific RNA sequences, which is beneficial for studies involving RNA structure and function. A downside to consider is the need for careful optimization of reaction conditions to maximize yield and quality. Parameters such as temperature, ion concentration, and enzyme activity must be monitored to achieve reproducible results.
Gene Expression and Protein Production
Promega T7 technology facilitates gene expression studies using T7-driven expression systems. It allows researchers to express recombinant proteins efficiently in various host systems, including bacteria, yeast, and mammalian cells. This capability has changed the landscape for protein production, making it easier to produce large amounts of proteins for research, drug development, and therapeutic applications.
Some of the advantages include:
- High-yield production: The T7 system can achieve higher expression levels than many other systems. This feature is essential for producing proteins in sufficient quantities for purification and functional assays.
- Post-translational modifications: Utilizing the correct host can allow for necessary modifications to proteins, which is critical for their functionality.
- Rapid expression: Researchers can quickly move from gene cloning to protein expression, significantly shortening the timelines for projects.
However, it is important to acknowledge that not all proteins express equally well. Some proteins might require additional optimizations and specific conditions to ensure proper folding and activity.
RNA Vaccines Development
With the emergence of mRNA vaccines, Promega T7 technology finds an essential application in vaccine development. The rapid production of RNA using T7 RNA polymerase allows scientists to create mRNA that can instruct cells to produce proteins that function as antigens. This process is crucial in eliciting a desired immune response.
Promegaβs T7 technology offers several benefits in vaccine research:
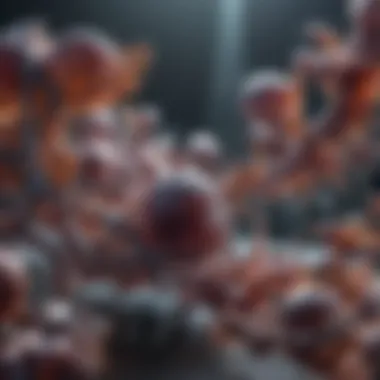
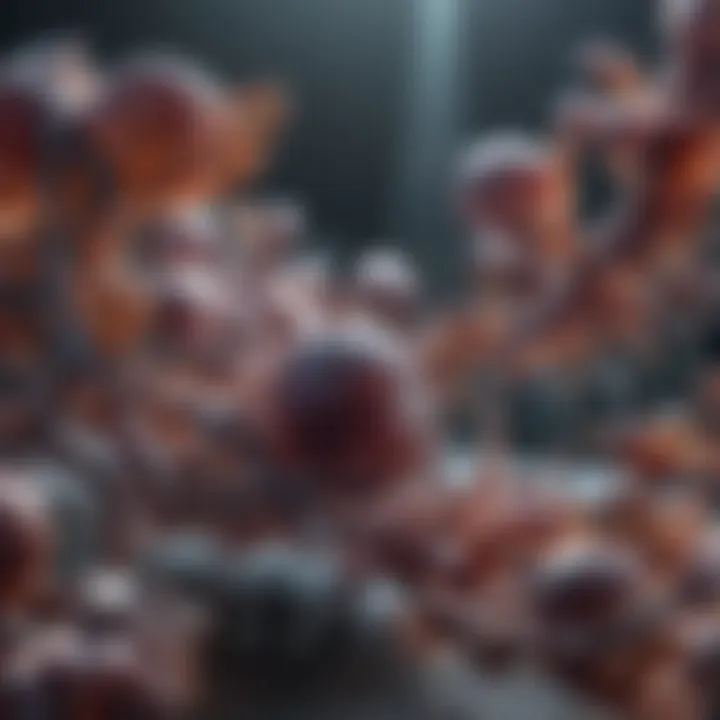
- Quick response times: The ability to synthesize mRNA rapidly means that vaccine candidates can be developed and tested in much shorter time frames. This capability has proved indispensable during health emergencies like pandemics.
- Scalability: Production can be scaled to meet large-scale demands for clinical trials and eventual rollout.
- Safety: Using in vitro transcription reduces the risk of contamination with pathogens, as there is no live virus involved in producing the RNA.
Despite these advantages, researchers must also ensure that the mRNA is accurately formulated to maintain stability and facilitate effective translation in target cells.
Promega T7 Systems: A Comparative Analysis
Promega T7 systems play a significant role in molecular biology by providing researchers with efficient tools for RNA synthesis and study. This section analyzes the distinctive features of Promega's platform compared to other transcription systems, underscoring its advantages and applications. Understanding these elements is essential for researchers making informed choices in their projects. By juxtaposing Promega T7 systems with alternative methods, we gain a clearer perspective on their efficacy and relevance in today's scientific landscape.
Advantages of Promega T7 Systems
Promega T7 systems come with several advantages that enhance their usability in molecular biology research:
- High Fidelity: Promega's T7 RNA polymerase is known for its accuracy in transcription, ensuring the produced RNA molecules faithfully represent the DNA template.
- Robust Performance: The system is designed to function efficiently across a range of conditions, allowing for flexibility in experimental setups.
- Ease of Use: The protocols provided are straightforward, making it accessible even for newer researchers. This is critical in educational settings and research labs alike.
- Compatibility: These systems are highly compatible with various downstream applications, including in vitro translation and structural studies.
- Scalability: Promega T7 systems can be scaled easily to accommodate different project sizes, making them suitable for both small-scale and larger experiments.
Overall, these advantages not only promote reproducibility in research but also foster innovation by simplifying complex procedures.
Alternative Systems Overview
While Promega T7 systems offer numerous benefits, it is also important to consider alternative transcription systems available in the market. These include:
- Ambion T7 Transcription Kits: Known for their efficiency, the Ambion kits are often used in RNA synthesis, but they may not provide the same level of detail in protocols as Promega.
- Thermo Fisher Scientific's MaxScript T7: This system is recognized for its versatility and application variety, though sometimes it struggles with sensitivity in output.
- Wako Pure Chemical's T7 RNA Polymerase: This option is sometimes favored for specific research needs, but the technical support and documentation may not match the levels offered by Promega.
Comparing these systems helps researchers identify which transcription kit aligns with their specific needs. Factors such as ease of use, performance under varying conditions, and technical support should be key determinants in the decision-making process.
Troubleshooting in T7 Transcription
Troubleshooting in T7 transcription is crucial for ensuring reliable results in molecular biology experiments. Various factors can affect the efficacy of T7 systems, leading to suboptimal yields or failed reactions. Understanding these issues and their solutions can save time and resources.
The importance of this section cannot be overstated, as a solid grasp of troubleshooting techniques enables researchers to design efficient experiments. Correctly identifying problems can make the difference between successful protein production or gene expression and frustration. Moreover, effective troubleshooting practices enhance reproducibility, which is essential for scientific validity.
Common Issues and Solutions
T7 transcription can encounter several common issues. Here are a few frequently observed problems and their corresponding solutions:
- Low RNA Yield
- Contamination with Enzymes
- Inefficient Transcription
- RNA Degradation
- Check the quality of the DNA template. Pure and intact DNA is critical for optimal transcription.
- Increase the duration of transcription. A longer incubation time may improve yield.
- Ensure appropriate enzyme concentrations. Using too little can limit reaction efficacy.
- Utilize clean, dedicated lab equipment to minimize risk of contamination.
- Implement enzyme inactivation steps where feasible to maintain integrity of your reactions.
- Verify that transcription buffer is correctly prepared. Buffers should be prepared fresh for each experiment.
- Assess temperature settings. Transcription often requires specific temperatures for optimal enzyme activity.
- Include RNase inhibitors in reactions to protect RNA from degradation.
- Store RNA samples promptly in appropriate conditions to prevent breakdown.
By addressing these common issues with targeted solutions, researchers can overcome many challenges in T7 transcription, streamlining the process significantly.
Optimization Strategies
Optimizing T7 transcription can dramatically enhance the quality and quantity of synthesized RNA. Here are essential strategies to consider:
- Template Design
Design templates with appropriate promoter sequences for T7 RNA polymerase. This step ensures efficient initiation of transcription. - Buffer Conditions
Experiment with different buffer compositions. For example, varying salt concentrations can influence enzymatic activity and yield. - Temperature Optimization
Conduct reactions at various temperatures to identify the optimal one for your specific template and enzyme combination. - Additives
Incorporate additives like betaine or DMSO. These can enhance transcription efficiency in difficult templates. - Time and Scale
Perform reactions at larger scales and for extended time periods to see if yields improve.
By applying these strategies, researchers can not only resolve current issues but also prevent future problems, leading to smoother workflows.
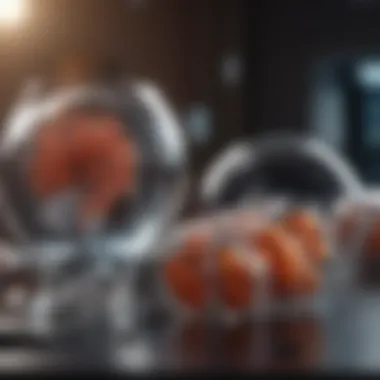
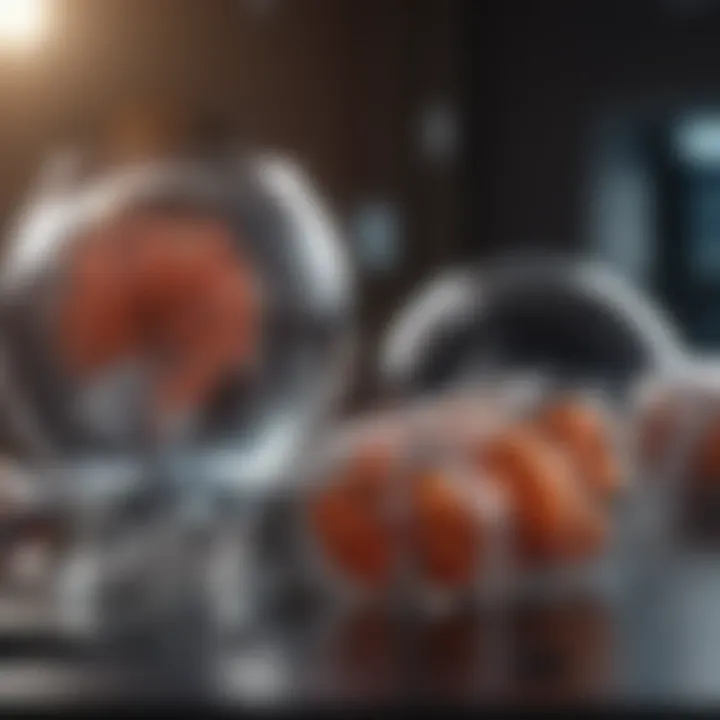
Optimization in T7 transcription workflow can be seen not just as a means to overcome issues but as an integral part of any experimental design. It empowers scientists to push boundaries in research.
Current Research Trends Involving T7 Technology
The exploration of Promega T7 technology continues to evolve rapidly within the field of molecular biology. Researchers are actively utilizing this technology as a strategic tool for various applications, particularly in gene editing and synthetic biology. Understanding the current trends makes clear the significance and versatility of T7 systems in modern genetics and bioengineering.
CRISPR and Gene Editing
Gene editing has expanded significantly due to advancements in T7 technology, particularly in combination with the CRISPR-Cas9 system. T7 RNA polymerase facilitates precise in vitro transcription of guide RNAs, required for targeting specific DNA sequences. This enhances the efficiency of CRISPR-based approaches, resulting in more successful genome modifications.
Some benefits of integrating T7 technology with CRISPR include:
- Higher Efficiency: Using T7 systems can lead to increased yield of functional guide RNAs, improving the rate of successful edits.
- Scalability: T7 technology allows for large-scale production of RNA components, making it easier to conduct extensive genetic studies.
- Versatility: Various T7-driven methods can adapt to different genomic targets, allowing researchers to customize their approaches based on specific experimental needs.
However, challenges remain. Researchers must consider potential off-target effects and the optimization of each component in the editing process. Addressing these concerns is crucial for the reliable application of CRISPR technologies.
Synthetic Biology Applications
Synthetic biology represents another frontier where T7 technology plays a critical role. It is leveraged for the engineering of biological systems, allowing for the creation of novel organisms or pathways. T7 systems provide an adaptable framework for synthesizing RNA and proteins, which are essential for building complex biological circuits.
Significant applications in this area include:
- Gene Synthesis: T7 systems can efficiently produce multiple genes through in vitro processes, helping to assemble larger genetic constructs needed for synthetic organisms.
- Metabolic Engineering: It allows programmers to redesign metabolic pathways in host organisms, enhancing their abilities to produce valuable compounds.
- Biocontainment Strategies: Researchers utilize T7 technology to design biological safeguards, ensuring genetically modified organisms cannot survive outside of controlled environments.
Future Directions in Promega T7 Technology
Understanding the future directions of Promega T7 technology is crucial for researchers and practitioners in molecular biology. This section discusses emerging trends and advancements that may redefine how this powerful tool is utilized. The continuous development in the field may enhance the efficiency, effectiveness, and applications of T7 technology. Insights into these advancements help establish clarity about how they can be integrated into ongoing and future research.
Innovations on the Horizon
Innovations in Promega T7 technology are likely to focus on optimizing transcription efficiency and extending the range of applications. Current research is exploring engineered T7 RNA polymerases that can exhibit superior processivity and fidelity. Moreover, novel co-factors and modifications may enhance the polymerase's performance in different conditions. Such innovations could increase the versatility of T7-driven systems for specific tasks in gene expression and vaccine production.
Another promising area is the development of real-time monitoring systems. Advances in fluorescence or bioluminescence will enable researchers to observe transcription events as they unfold. This capability will improve the understanding of transcription dynamics. Additionally, integrating T7 systems with other technologies, such as CRISPR or next-generation sequencing platforms, may open avenues for groundbreaking applications.
Expanded Applications in Research
The applications of Promega T7 technology are expanding rapidly across various fields. One notable direction is within the realm of personalized medicine, where T7 technology assists in creating tailored RNA constructs for therapeutic use. This capability is essential for advancing targeted therapies, including those aimed at specific genetic markers.
In synthetic biology, researchers are increasingly leveraging T7 technology to construct new biological systems. It allows for precise control over gene expression, enabling the design of more efficient microorganisms for biofuel production or environmental remediation.
Furthermore, the role of T7 technology in the production of mRNA vaccines has gained prominence. The need for rapid and scalable production of mRNA has never been more evident. T7 systems can streamline the process and enhance yield, making it a valuable tool in vaccine development against emerging infectious diseases.
"The adoption of T7 technology in innovative applications reflects its foundational role in modern molecular biology."
As we look to the future, the integration of artificial intelligence in optimizing transcription protocols poses an intriguing possibility. AI can analyze vast datasets to identify optimal conditions for T7 transcription, further enhancing productivity.
In summary, as we explore the future directions of Promega T7 technology, its potential to impact molecular biology is vast. Innovations and expanded applications promise to deliver new methodologies and insights, maintaining its essential role in research and practical applications.
Ending
In this article, we have explored the significance of Promega T7 technology in molecular biology. The ability to produce RNA transcripts efficiently is a cornerstone in various fields, including genetic engineering and vaccine development. With T7 RNA polymerase, researchers can transcribe a DNA template into RNA with high fidelity and yield. This technology also revolutionizes how scientists study gene function and protein expression.
Summary of Key Points
- T7 RNA Polymerase is critical for synthesizing RNA from DNA templates.
- Applications of T7 technology include in vitro transcription, protein production, and RNA vaccine development.
- Promega's specific systems have advantages over other available systems, making them a popular choice in laboratories.
- Troubleshooting strategies are vital for optimizing T7 transcription and overcoming common issues encountered during research.
- Current research trends concerning T7 technology include applications in CRISPR and synthetic biology, showcasing its versatility and relevance.
- Future directions offer exciting possibilities for expanding the use of T7 technology in various scientific disciplines.
Final Thoughts on Promega T7 Technology
Promega T7 technology stands as a vital tool in molecular biology research. It addresses significant challenges researchers face in transcription and transcriptional regulation. The accessibility of Promega's products enables researchers and educators to harness this technology effectively. As molecular biology advances, so too will the applications and methodologies surrounding T7 transcription systems. Embracing these developments is essential for any individual or institution aiming to remain at the forefront of biological research.
"Understanding T7 technology is like unlocking new pathways in molecular biology that enhance our scientific explorations."