Next Generation Sequencing and Bioinformatics Insights
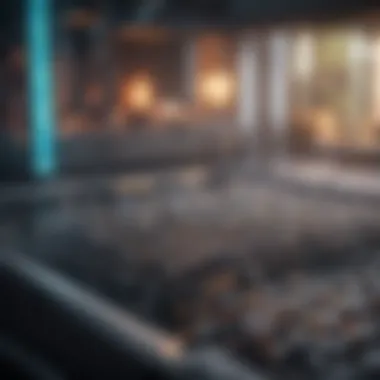
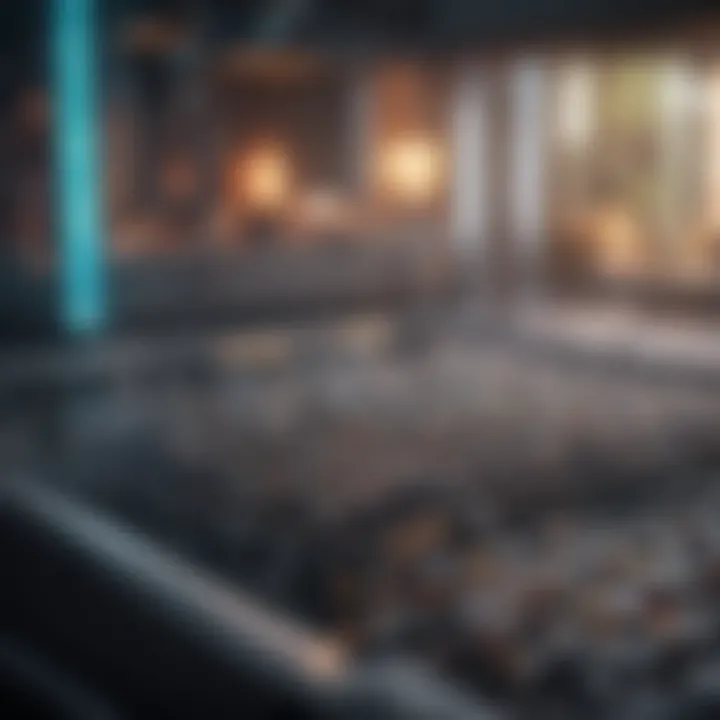
Intro
Next Generation Sequencing (NGS) and bioinformatics are increasingly defining the horizon of modern biological research. The intersection of these two fields has sparked remarkable advancements in genomics, driving discoveries that influence areas such as medicine, agriculture, and evolutionary biology. This article embarks on a detailed exploration of NGS and bioinformatics, aiming to provide readers with a thorough understanding of the current landscape, including technological advancements, practical applications, and potential future directions.
As sequencing technologies mature and data generation becomes more accessible, the need for proficient analytical tools in bioinformatics rises. These tools are essential for deciphering the relevant biological information hidden within the vast amounts of data produced by NGS techniques. Whether in the realm of personalized medicine or large-scale genomic studies, the interplay between NGS and bioinformatics serves a critical role in transforming raw sequences into meaningful insights.
In the sections to follow, we will delve into:
- Key principles of NGS
- Various bioinformatics tools
- Challenges faced in the integration of these technologies
- Implications for future research and therapies
Together, these elements will frame a comprehensive overview of how NGS and bioinformatics shape biological inquiries today and how they might evolve in upcoming decades.
Preface to Next Generation Sequencing
Next Generation Sequencing (NGS) represents a significant leap in the field of genetics and molecular biology. This transformative technology enables rapid sequencing of entire genomes, providing insights that were once beyond reach. It serves as a foundation for modern biological research, including genomics, transcriptomics, and beyond.
NGS is important for several reasons. Firstly, it dramatically reduces the time and cost associated with sequencing. Traditional methods, like Sanger sequencing, are often labor-intensive and slow. In contrast, NGS allows for the simultaneous sequencing of millions of fragments, vastly increasing throughput. This capability enables researchers to undertake large-scale projects, such as whole-genome sequencing, which was previously impractical for many labs.
Another critical aspect of NGS is its contribution to personalized medicine. By analyzing an individual’s genetic makeup, healthcare providers can tailor treatments more effectively, leading to better patient outcomes. Furthermore, NGS plays a vital role in understanding diseases at the genetic level, opening new avenues for diagnosis and therapeutic interventions.
The following sections will outline the definition and significance of NGS, and detail its historical context, revealing how this technology has evolved over time. This introductory segment sets the stage for a comprehensive exploration of NGS and its integration with bioinformatics, emphasizing the coupling of these two fields as they drive advancements in research and clinical applications.
Definition and Importance
Next Generation Sequencing can be defined as a set of advanced DNA sequencing technologies that allow for the rapid sequencing of an entire genome. Unlike earlier methods, NGS can simultaneously carry out massive parallel sequencing of millions of DNA strands, providing a huge amount of data in a comparatively short time.
The significance of NGS extends into various fields. In research, it powers genetic studies, genome assembly, and variant discovery, among others. In clinical settings, NGS is pivotal for cancer genomics and hereditary disease diagnosis, where understanding genetic variations is crucial for effective treatment plans.
History and Evolution
The history of sequencing began with Sanger sequencing in the 1970s, which laid the groundwork for genetic analysis. This method was revolutionary at the time but had limitations regarding the speed and cost of sequencing.
The advent of NGS in the early 2000s marked a critical turning point. The first NGS technology, developed by 454 Life Sciences, enabled an entire human genome to be sequenced in just a few days instead of the years required by Sanger sequencing. This rapid advancement set in motion a series of innovations that led to the development of various NGS platforms, including Illumina and Ion Torrent technologies.
The drive towards efficient and cost-effective sequencing has continued to evolve, making NGS an indispensable tool in modern biology and medicine. Understanding its history helps appreciate the remarkable pace of technological advancement and its impact on research and healthcare.
Key Technological Advancements
Key technological advancements in next generation sequencing (NGS) and bioinformatics have significantly shaped the landscape of genomic research. By streamlining processes, these innovations lead to enhanced accuracy, speed, and overall efficacy in analyzing genetic information. Such advancements are foundational for various applications from basic research to clinical settings. Understanding these elements is essential for anyone engaged in the fields of genomics and bioinformatics.
Sequencing Platforms
The choice of sequencing platform significantly impacts the quality and type of data obtained from NGS. The most crucial platforms available today include Illumina Technology, Ion Torrent, and PacBio and Nanopore Sequencing. Each platform comes with specific characteristics that cater to particular needs in genomic research.
Illumina Technology
Illumina Technology is widely regarded as the leading sequencing platform in the field. It is known for its high throughput capability which allows for sequencing millions of fragments simultaneously.
This technology employs a sequencing-by-synthesis method that generates a significant amount of data quickly and with substantial accuracy. The primary benefit of Illumina sequencing is its cost-effectiveness for high-volume data generation, making it a common choice for large-scale projects. However, its short-read length (up to 300 base pairs) can be a limitation in resolving complex genomic regions such as repeat sequences.
Advantages:
- High throughput and lower cost per base.
- Reliable and accurate data generation.
Disadvantages: - Shorter read lengths can complicate genome assembly.
Ion Torrent
Ion Torrent is another prominent platform, distinguished by its unique approach of detecting changes in pH that occur during the sequencing of DNA. This method allows for relatively rapid sequencing and offers the ability to perform real-time data analysis.
The benefit of Ion Torrent lies in its flexibility, allowing for adjustments based on project needs. It has gained popularity among researchers looking for quick results, especially in smaller studies. However, its read accuracy can be somewhat lower than that of Illumina, which may present challenges for certain applications.
Advantages:
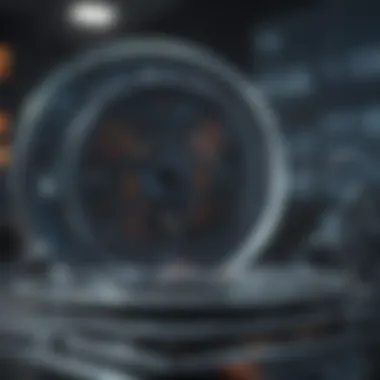
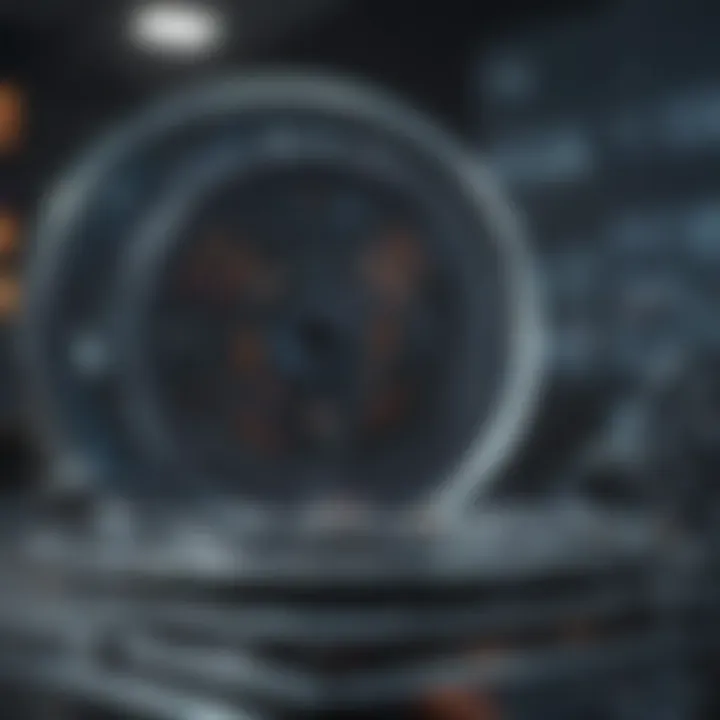
- Fast turnaround time for sequencing data.
- Cost effective for smaller studies.
Disadvantages: - Lower accuracy in comparison to Illumina.
PacBio and Nanopore Sequencing
PacBio and Nanopore Sequencing are groundbreaking technologies that provide long-read sequencing capabilities. PacBio utilizes single-molecule real-time sequencing (SMRT) which allows for longer reads that can improve the resolution of complex genomic regions. Similarly, Nanopore technology offers the unique ability to sequence DNA strands as they pass through a nanopore.
Both technologies serve as valuable tools for deciphering structural variations and for conducting full-length transcript sequencing. Their primary drawback is the higher costs involved and the lower throughput relative to short-read platforms. Nevertheless, their unique capabilities make them indispensable in certain genomic studies.
Advantages:
- Capable of resolving difficult genomic regions.
- Suitable for comprehensive genomic analysis.
Disadvantages: - Typically higher costs and lower throughput.
Library Preparation Techniques
Library preparation is a critical phase in NGS that directly influences the quality of data obtained. Techniques in this area often involve fragmenting DNA or RNA, followed by the addition of various adapters that facilitate sequencing. Different methods exist, such as whole-genome amplification and PCR enrichment. Choices in library preparation impact sequencing efficiency and the successful capture of diverse genomic regions.
In summary, advancements in sequencing platforms and library preparation techniques are at the forefront of NGS progress. Assessing these key elements allows researchers to choose suitable methods for their specific applications, ensuring accurate and meaningful genomic insights.
Bioinformatics: An Overview
Bioinformatics is a crucial field that integrates biology, computer science, and statistics. It serves the purpose of managing biological data, particularly in the context of next generation sequencing (NGS). As the volume and complexity of genomic data increases, bioinformatics becomes indispensable. It allows researchers to handle large datasets efficiently and extract meaningful insights from them.
Definition and Significance
Bioinformatics can be defined as the application of computational tools to manage, analyze, and interpret biological data. This field is significant for several reasons:
- Data Management: With growing sequencing technologies, bioinformatics provides effective ways to organize and store the vast amounts of genetic data generated.
- Data Analysis: It offers a suite of tools for analyzing raw sequencing data. Techniques like genome assembly, alignment, and functional annotation rely heavily on bioinformatics.
- Predictive Modeling: Bioinformatics contributes to predictive models that help understand gene function and interaction, further aiding in the study of complex biological systems.
The significance of bioinformatics is evident in areas such as personalized medicine, where patient outcomes can improve through tailored treatment plans based on individual genetic profiles. Understanding bioinformatics is essential for anyone involved in genomics and computational biology.
Historical Context
The history of bioinformatics aligns closely with the progression of biology and technology. Early applications emerged in the 1960s, focusing on DNA sequencing methods. The advent of the Human Genome Project in the 1990s marked a major milestone for bioinformatics, spotlighting the need for advanced computational tools as the research aimed to map the entire human genome. As sequencing technologies evolved, the field expanded rapidly.
In the 2000s, increased computational power and improved algorithms allowed bioinformatics to flourish. New databases emerged, like GenBank and Ensembl, which facilitated better data sharing and accessibility. Today, bioinformatics encompasses a broad spectrum of subfields, including genomics, transcriptomics, and systems biology, reflecting its vital role in contemporary biological research.
"Bioinformatics helps bridge the gap between biological sciences and computational techniques, making it a cornerstone of modern genomics."
Core Components of Bioinformatics
Bioinformatics stands as a crucial bridge between raw biological data and its meaningful analysis. In the realm of next generation sequencing, understanding bioinformatics components is vital. These core elements enable the management, storage, and analysis of data generated during sequencing experiments. Effective bioinformatics facilitates insights into genetic information, leading to breakthroughs in research and clinical applications.
Data Acquisition
Data acquisition refers to the process of collecting biological data. This is the first step where information is obtained from various sequences and samples. Raising standards in data acquisition is significant as it sets the foundation for subsequent analyses. High-fidelity data is essential. Techniques such as PCR amplification and various sequencing methods contribute to efficient data acquisition. Through these methods, the quality of data can be monitored, ensuring accuracy at later stages.
Data Management and Storage
Next, managing and storing data becomes critical. Given the enormous volume of data produced in sequencing efforts, effective storage solutions are needed. Traditional data storage often proves insufficient. Therefore, cloud computing has emerged as a favored choice. It offers scalability and flexibility, accommodating the increasing data requirements of bioinformatics.
Also relevant, databases such as GenBank and the European Nucleotide Archive support data storage by providing structured formats for huge datasets. These databases ensure that researchers have access to reliable data for their studies. This aspect of bioinformatics addresses not only storage but also retrieval, which is crucial for researchers looking to analyze specific datasets.
Data Analysis Tools
Data analysis tools are pivotal in interpreting the vast datasets generated. These tools help in making sense of the acquired data, leading to meaningful results and conclusions. Two main categories of tools exist: alignment algorithms and variant calling tools. These tools have distinct functions yet are interlinked in the bioinformatics workflow.
Alignment Algorithms
Alignment algorithms play a significant role in comparing sequences. The main goal is to identify similarities and differences between sequences. One of the key characteristics of these algorithms is their ability to handle large datasets efficiently. They are popular choices due to their effectiveness in aligning millions of sequences to a reference genome. A unique feature is their use of scoring systems that help in identifying optimal alignments. While alignment algorithms can be resource-intensive, their benefits in providing accurate results often outweigh their computational demands.
Variant Calling Tools
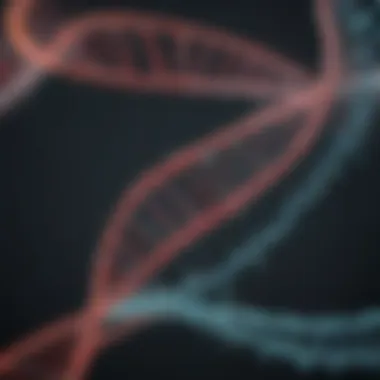
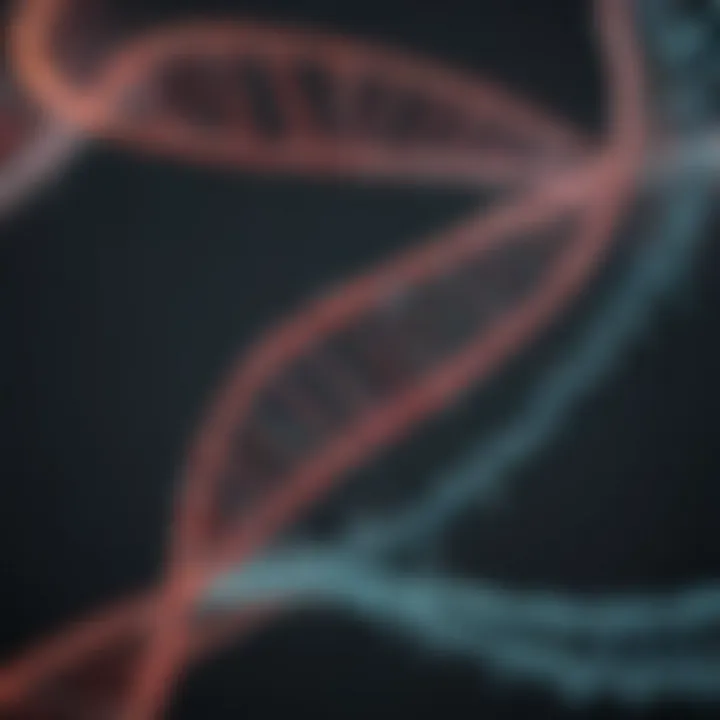
Variant calling tools are essential for identifying genetic variants from genomic sequences. From the data produced, these tools determine differences, such as single nucleotide polymorphisms, insertions, or deletions. A notable characteristic is their high sensitivity, allowing researchers to detect even minor variations. This makes them a beneficial choice for studies focusing on genetic diversity or disease understanding. However, it's important to consider that false positive calls can occur with variant calling. Thus, optimizing parameters is crucial in improving the accuracy of results.
The importance of robust data analysis tools cannot be overstated in bioinformatics. They provide the necessary framework to extract biological meaning from vast datasets, ultimately driving scientific discoveries forward.
Applications of NGS in Research
Next Generation Sequencing (NGS) has revolutionized the field of research across various disciplines. It substantially enhances our understanding of genetic material and provides critical insights into health, evolution, and biodiversity. The application of NGS in research can be classified into multiple domains, each offering unique benefits and methodologies. This section explores three major areas: Genomics and Transcriptomics, Metagenomics, and Clinical Applications.
Genomics and Transcriptomics
Genomics refers to the comprehensive study of an organism's entire set of DNA, including its genes. NGS facilitates massive parallel sequencing, making it possible to decode complex genomes in unprecedented detail. This capability allows researchers to identify genetic variations that influence traits, diseases, and evolution.
Transcriptomics extends genomics by analyzing RNA transcripts produced within a cell. NGS technologies enable the sequencing of RNA molecules, providing insights into gene expression patterns. These insights are fundamental for understanding cellular responses to various stimuli and conditions. As a result, genomics and transcriptomics contribute to advancements in agricultural science, evolutionary biology, and personalized medicine.
Metagenomics
Metagenomics is the study of genetic material recovered directly from environmental samples. NGS allows for the analysis of microbial communities without needing to culture organisms in a laboratory. This is crucial for studying complex ecosystems, such as soils, oceans, and the human microbiome.
Through NGS, researchers can characterize microbial diversity, uncover novel species, and investigate the functional roles of different microorganisms in their environments. This understanding has implications for ecology, infectious disease diagnosis, and even biotechnology, where microbial consortia can be harnessed for various applications.
Clinical Applications
Clinical applications of NGS are transformative in the field of medicine. They have broad implications for diagnosing genetic disorders, informing treatment strategies, and improving patient outcomes. Two key areas within clinical applications are personalized medicine and cancer genomics.
Personalized Medicine
Personalized medicine leverages genetic information to tailor treatment to individual patients. The key characteristic of personalized medicine is its focus on the unique genetic profile of each patient, allowing for more effective and targeted therapies. This approach is increasingly becoming a popular choice in oncology, pharmacology, and various other medical fields.
A unique feature of personalized medicine is its capacity to minimize side effects by matching drugs with patients' genetic makeups. However, it also presents challenges, such as the need for extensive genomic data and ethical considerations surrounding privacy and data security.
Cancer Genomics
Cancer genomics emphasizes the role of genetic mutations in cancer development and progression. Understanding these mutations is crucial for identifying potential targets for personalized treatment. The important characteristic of cancer genomics lies in its capacity to not only enhance diagnostic accuracy but also predict the disease's response to specific therapies.
A notable advantage of cancer genomics is its potential to facilitate early detection and intervention, ultimately improving patient survival rates. Nevertheless, it comes with disadvantages as well. The complexity of tumor heterogeneity can complicate treatment approaches, requiring continuous adaptations to evolving genetic landscapes.
The integration of NGS into various aspects of research, particularly genomics, metagenomics, and clinical applications, marks a significant advancement in our understanding of life sciences.
Challenges Faced in NGS and Bioinformatics
Next Generation Sequencing (NGS) and bioinformatics represent forefronts of biotechnological research. However, these fields face several challenges that professionals, researchers, and educators must navigate. Understanding these challenges is vital because it informs the development of effective strategies to maximize the utility of sequencing data and ensures that the findings are applied correctly in various fields, including personalized medicine and clinical research.
Data Volume and Complexity
The sheer volume of data generated from NGS is staggering. Current sequencing methods can produce terabytes of information from a single run. This enormous scale brings about significant complexities regarding data management. Storing and processing such large datasets requires substantial computational resources. Moreover, organizing this data effectively is crucial. Without proper systems in place, researchers might overlook critical information hidden within the datasets.
The complexity is not just in volume but also in the nature of the data itself. NGS data is highly heterogeneous, encompassing variations in sequence quality, read length, and coverage, which can complicate the analysis. It is imperative for bioinformatician to develop robust pipelines that can handle this complexity. Efficient algorithms and data processing tools are essential to derive meaningful insights from raw sequence data.
Interpretation of Results
Interpreting the results produced by NGS is another significant challenge. The findings derived from sequencing data can be ambiguous or conflicting, leading to potential misinterpretations. This becomes particularly problematic in clinical applications where decisions based on these results impact patient outcomes. Researchers must employ rigorous statistical methods and validation techniques to ensure the reliability of their findings.
Additionally, with the growing volume of genomic data in public databases, researchers are faced with the challenge of distinguishing clinically relevant differences from benign variations. The need for comprehensive annotation and interpretation tools is crucial. Establishing standardized protocols can help mitigate interpretation issues and enhance the trustworthiness of results.
Integration with Clinical Practices
Integrating NGS and bioinformatics into routine clinical practice presents significant challenges. While the technological capabilities exist, the healthcare infrastructure often struggles to keep pace with innovations. There are issues surrounding the standardization of tests and translation of genomic findings into actionable clinical data.
Furthermore, healthcare professionals require adequate training on how to interpret genetic information responsibly and effectively. The implementation of policies that govern the use of genetic data is also vital to ensure ethical considerations are taken into account. Collaboration between bioinformaticians, clinicians, and policy makers is necessary to bridge this gap and facilitate the integration of these advanced technologies into everyday healthcare.
Future Directions in NGS and Bioinformatics
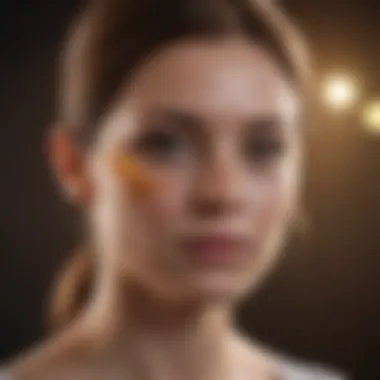
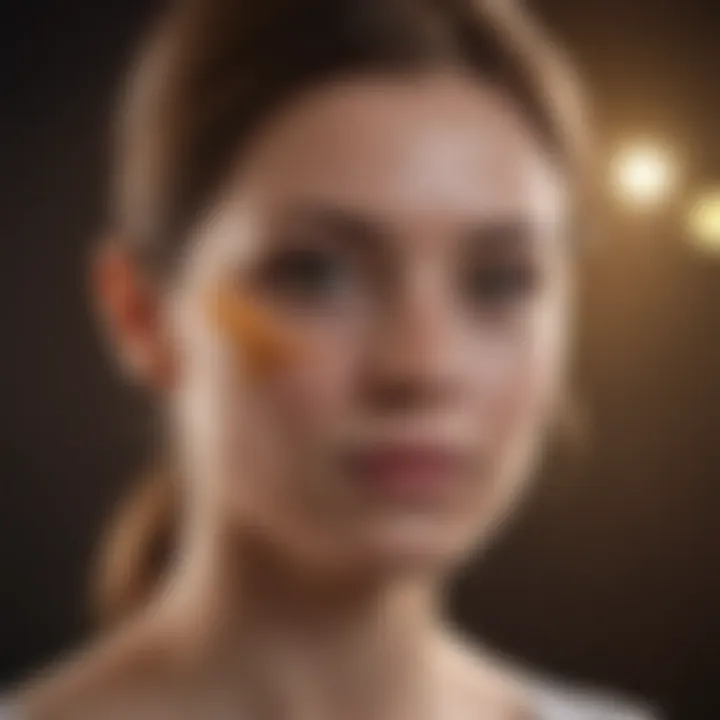
The landscape of Next Generation Sequencing (NGS) and bioinformatics is evolving rapidly. This section focuses on the upcoming trends that could reshape these fields, emphasizing technological advancements and the transformative role of artificial intelligence.
Emerging Technologies
The pace of innovation in NGS technology is relentless. New platforms and methodologies emerge frequently. These innovations promise not just to enhance the speed and accuracy of genomic sequencing but also to reduce costs significantly. Recent advancements in sequencing technologies, like single-cell sequencing and long-read sequencing, enable researchers to gather information at a much finer resolution. This granularity can uncover details about cellular diversity in tissues and organs that were previously hidden.
For instance, companies like Oxford Nanopore Technologies have developed portable sequencing devices. These devices enable real-time data generation, allowing scientists to analyze samples in situ, which may prove crucial during outbreaks.
Moreover, with the advent of CRISPR technology, there are innovative methods to edit genomes. These enhancements in sequencing methods linked with gene editing applications could lead to significant strides in personalized medicine, agricultural biotechnology, and numerous other fields.
Key Emerging Technologies to Watch:
- Single-cell sequencing
- Long-read sequencing platforms
- Portable sequencing devices
- Integration of NGS with geospatial and environmental data
Role of Artificial Intelligence
Artificial intelligence (AI) is becoming essential in bioinformatics. It aids in processing the vast amounts of data generated by NGS. Algorithms powered by machine learning can identify patterns in genomic data that may be overlooked manually. For example, AI techniques are being applied in variant interpretation, which is crucial for personalized medicine.
Furthermore, predictive analytics enabled by AI enhance our understanding of disease mechanisms. The ability to asses large datasets allows researchers to identify potential biomarkers for diseases earlier than traditional methods would allow.
In clinical settings, AI tools can also help doctors in making informed decisions swiftly. By integrating medical histories and genomic data, AI can assist in tailoring treatment for individual patients.
"The application of AI in genomics is not just about efficiency; it is about achieving insights from data that were unattainable without such technologies."
Considerations for AI in Bioinformatics:
- Ethical implications regarding data privacy
- The need for transparency in AI algorithms
- Collaboration between biologists and data scientists for effective solutions
Ethical Considerations
Ethical considerations in the realm of next generation sequencing (NGS) and bioinformatics are of paramount importance as they address both the implications of data handling and the broader societal impact. Given the sensitivity of genetic data, it becomes essential to strike a balance between innovation and ethical responsibility. This section highlights critical elements such as data privacy, security, and the societal consequences of advances in genetic research.
Data Privacy and Security
Data privacy is a major concern in genomic studies. The increasing amount of genetic data generated by NGS raises questions about who controls this information, how it is collected, and how it is stored. Genetic data can reveal not just individual health risks but also information about family relations and ancestral backgrounds. Therefore, safeguarding this data against unauthorized access is crucial.
Organizations working with genomics must implement stringent protocols to ensure the security of health records and genetic information. This includes employing encryption, secure access controls, and compliance with regulations such as HIPAA (Health Insurance Portability and Accountability Act). Failure to protect this data could lead to breaches that not only compromise personal information but can also erode public trust in genomic research.
**"Ensuring data security in bioinformatics is not just a technical necessity; it is ethical responsibility."
Moreover, participants in genomic research should be fully informed about how their data will be used and who has access to it. Informed consent plays a crucial role in protecting the privacy rights of individuals. Lack of transparency can lead to exploitation and misuse of genetic data, reinforcing the need for ethical standards in research practices.
Impact on Society
The societal impact of NGS and bioinformatics extends beyond individuals, affecting families, communities, and even entire populations. Knowledge gained through genomics can lead to significant benefits, including advancements in personalized medicine, which tailor healthcare to an individual's genetic makeup. Such progress has the potential to improve treatment outcomes and reduce healthcare costs.
However, this progress raises ethical questions about equity in access to genetic technologies. If only certain socioeconomic groups benefit from these advancements, it could exacerbate existing health disparities. Therefore, it is necessary to consider how to make genomic technologies accessible to all, ensuring that benefits are distributed fairly.
Additionally, genetic information can sometimes lead to stigmatization. Individuals found to have specific genetic predispositions may face discrimination in employment or insurance. It is vital to establish policies that prevent such outcomes while promoting the responsible use of genetic information.
Finale
In summary, the discussion of next generation sequencing (NGS) and bioinformatics culminates in a nuanced understanding of their critical roles in modern biology. NGS technologies are rapidly advancing, leading to vast amounts of data generation. Consequently, bioinformatics emerges as an essential discipline that enables researchers to interpret and manage this complex data effectively.
A significant aspect presented in this article is the relationship between technological innovation and its applications. With more accessible sequencing technologies, applications in genomics, metagenomics, and personalized medicine become more impactful. Researchers can now explore genetic variations with unprecedented ease, paving the way for advancements in health care and biological research.
One of the key insights is the importance of integrating bioinformatics tools with sequencing efforts. Without robust data management and analytical strategies, the potential of NGS cannot be fully realized. This intersection is what drives scientific discovery and informs clinical applications, illustrating the necessity of collaboration between biologists, computer scientists, and healthcare professionals.
Ethical considerations, particularly regarding data privacy and societal implications, also deserve attention. As we navigate through the proliferation of genetic data, addressing these concerns is vital to maintain public trust and to optimize the benefits of NGS in everyday life.
As we consider future directions, emerging technologies such as artificial intelligence may reshape the landscape of bioinformatics. Machine learning algorithms can enhance data analysis, potentially leading to quicker and more accurate insights. This evolution promises to further intertwine NGS with bioinformatics, creating a robust framework for future biological research.
Therefore, the thorough exploration of both NGS and bioinformatics highlights their interconnected nature and emphasizes the importance of continuous evolution in both fields. The interplay of these domains not only enhances our understanding of life at a molecular level but also holds the key to transformative applications in health and disease management.
Summary of Key Insights
- Technological Advancement: NGS technologies are fundamental to data generation in genomics.
- Role of Bioinformatics: Critical for organizing and analyzing vast data from sequencing efforts.
- Interdisciplinary Collaboration: Essential for harnessing findings from sequencing in clinical and research settings.
- Ethical Considerations: Importance of safeguarding data privacy as NGS becomes more prevalent.
- Future Trends: Artificial intelligence may play a transformative role in bioinformatics applications.