The Role of Microscopes in Advancing Scientific Discovery
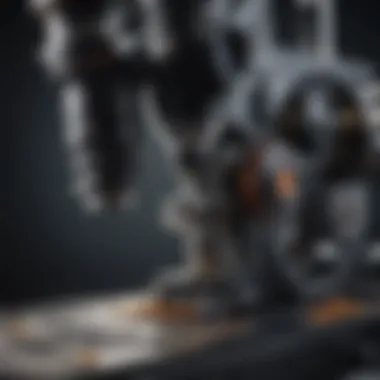
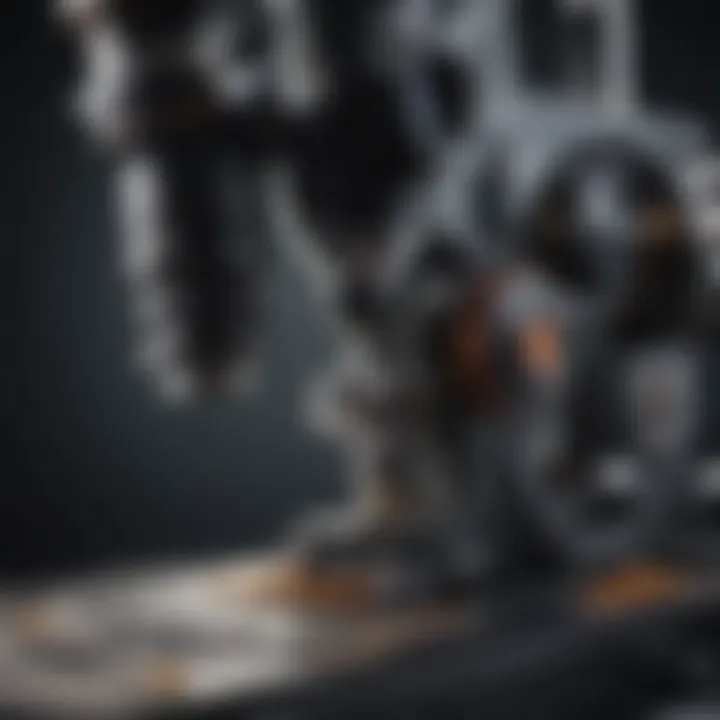
Intro
The evolution of microscopy has fundamentally reshaped the landscape of scientific discovery. Understanding the role of microscopes offers insight into how researchers explore the unseen details of life and materials. This article aims to illuminate the significance of these instruments in advancing various scientific fields, including biology, chemistry, and materials science.
Microscopes bridge the gap between the macroscopic and microscopic worlds, facilitating observations that are critical for experimentation and analysis. From early optical microscopes to today's advanced digital systems, their evolution reflects progress in both scientific inquiry and technological innovation. This intersection lays the groundwork for understanding the intricate details of structures and processes at smaller scales, which in turn enhances our comprehension of the larger systems in which they exist.
Research Overview
Summary of Key Findings
Microscopes have played an essential role in a wide range of research disciplines. Key findings include:
- The ability to visualize cellular structures has led to significant advances in biology, particularly in cell theory and microbiology.
- In chemistry, microscopy assists in interpreting molecular arrangements and interactions, crucial for chemical synthesis and reaction monitoring.
- Materials science benefits from microscopy through the analysis of materials at the atomic level, aiding in the development of new substances with desirable properties.
Research Objectives and Hypotheses
The primary objective is to explore how microscopy enhances scientific understanding and research methodologies. Several hypotheses guide this inquiry:
- Microscopy increases the accuracy of scientific observations, enabling more reliable data collection.
- Advanced microscope technologies lead to novel scientific discoveries by providing insights into previously unattainable structures.
- Educational applications of microscopy foster deeper comprehension of scientific principles among students.
Methodology
Study Design and Approach
This article employs a comprehensive literature review, examining peer-reviewed studies and historical advancements in microscopy. Additionally, case studies highlight the applications of various types of microscopes across disciplines. The approach focuses on understanding the progression of microscopy and its ramifications for scientific exploration.
Data Collection Techniques
Data collection involves synthesizing existing research, analyzing methodological frameworks employed in different studies, and extracting relevant findings related to microscope applications. Emphasis is placed on:
- Comparing evolving microscopy techniques and their contributions.
- Understanding how these advancements correlate with breakthroughs in scientific methodology.
"Microscopes have not only expanded our vision but have fundamentally altered our conceptualization of complexity in the scientific world."
In summary, the role of microscopes in science is profound and multifaceted. As the foundation for dissecting smaller details of the universe, their impact extends across various domains of study. The next sections will further delve into specific types of microscopes and their applications in various scientific fields.
Prelims to Microscopy
Microscopy is a pivotal science that underpins much of modern research. It allows scientists to observe and analyze structures that are too small to be seen by the naked eye. This section serves as a gateway to understanding what microscopy is and its crucial role in scientific discovery.
Definition and Importance
Microscopy can be defined as the technical field that involves the use of microscopes to view samples and objects that are otherwise invisible. The importance of microscopy lies in its ability to reveal the complex architecture of cells, tissues, and materials. Without microscopy, many discoveries in biology, materials science, and chemistry might not have been possible.
Microscopy has transformative potential across numerous disciplines:
- In biology, it enables researchers to study cellular structures, leading to insights about function and pathology.
- In materials science, it assists in the exploration of nanoscale phenomena, guiding advancements in nanotechnology.
- In chemistry, it provides critical information about chemical reactions and interactions on a microscopic scale.
Thus, microscopy serves as an essential tool in advancing knowledge and fostering innovation.
Historical Context
The history of microscopy dates back to the late 16th century with the invention of the compound microscope by Zacharias Janssen. This early device laid the foundation by enhancing the visibility of small objects, sparking interest in the microscopic world. Over the centuries, improvements in lens crafting and illumination techniques propelled microscopy into new realms.
In the 19th century, notable advancements were made by scientists like Robert Hooke and Antonie van Leeuwenhoek. Hookeβs work "Micrographia" introduced the term "cell" and showcased the intricate details of various specimens. Leeuwenhoek, often called the "father of microbiology," was the first to observe and describe single-celled organisms with his simple microscope.
As science progressed into the 20th century, new types of microscopes emerged, including electron and scanning tunneling microscopes. These innovations have increased magnification and resolution dramatically, further opening opportunities for exploration in various fields of study. The evolution of microscopy is a testament to human curiosity and ingenuity, and it continues to evolve today.
Types of Microscopes
Microscopes are essential instruments in scientific inquiry, enabling researchers to observe and analyze structures that are otherwise invisible to the naked eye. This section explores the primary types of microscopes used in contemporary science, discussing their unique characteristics, applications, and the vital role they play in advancing our understanding of various scientific fields.
Optical Microscopes
Optical microscopes are perhaps the most well-known type of microscope, utilizing visible light and lenses to magnify samples. They are capable of magnifying objects several hundred times. The benefits of optical microscopes lie in their simple setup and operation, making them accessible for educational and research purposes.
Bright Field Microscopy
Bright field microscopy is a widely used technique, where light passes directly through the specimen, allowing for a clear, illuminated image. It is particularly beneficial for viewing stained or naturally pigmented samples. One key characteristic is its straightforward method of preparation and observation, which makes it suitable for beginner researchers in laboratories. However, the limitations include difficulty in visualizing transparent specimens without staining, which can alter the sample's natural state.
Dark Field Microscopy
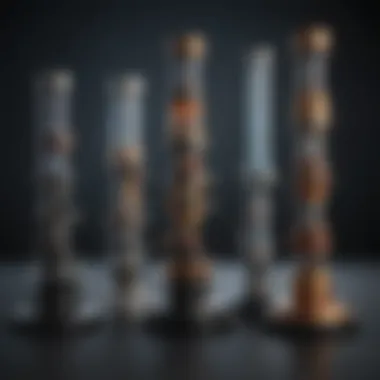
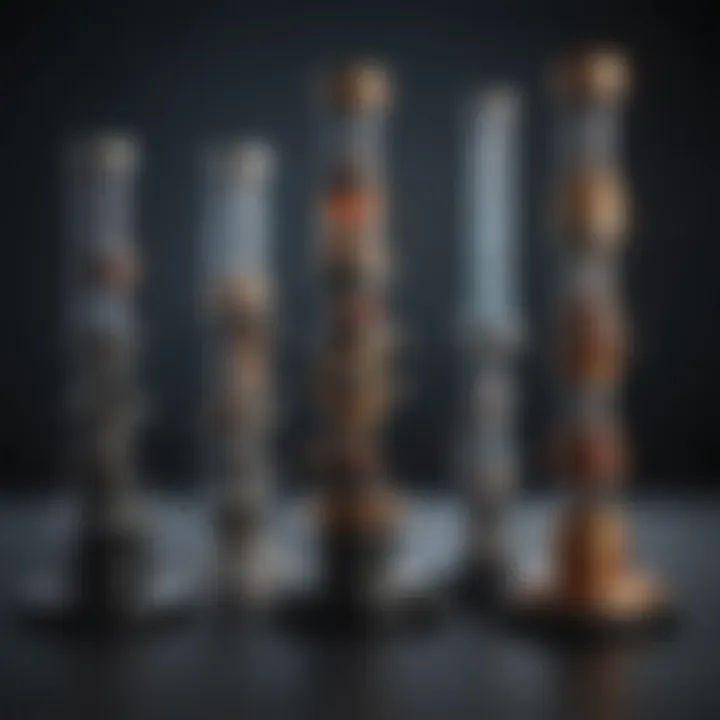
Dark field microscopy enhances contrast by illuminating the sample at an angle, making the background dark while the specimen appears bright. This technique is valuable for observing live, unstained cells and fine details not visible in bright field microscopy. Its unique feature of high contrast allows for the visualization of small structures and particles without the need for staining. The downside, however, is that it may produce artifacts that can mislead interpretation.
Phase Contrast Microscopy
Phase contrast microscopy allows researchers to view transparent specimens without staining by converting phase shifts in light passing through the specimen into variations in brightness. This method excels in observing living cells and microorganisms in their natural states. The primary advantage is the ability to see fine details of cells, such as their organelles. Yet, one disadvantage is that it can sometimes produce halos around specimens, which may complicate analysis.
Electron Microscopes
Electron microscopes utilize beams of electrons instead of light to achieve much higher resolutions, allowing for the observation of very small structures at the nanometer scale. This category of microscopes plays a crucial role in detailed material analysis and biological studies at the cellular level.
Transmission Electron Microscopy
Transmission electron microscopy (TEM) involves transmitting electrons through a thin specimen to form an image. This technique is essential in various fields, including biology, for high-resolution imaging of cell structures. Its key characteristic is the remarkable resolution achieved, often reaching a few nanometers. However, sample preparation can be complex and time-consuming, requiring thin sections of specimens, which may limit some applications.
Scanning Electron Microscopy
Scanning electron microscopy (SEM) offers a different approach by scanning a focused electron beam over the surface of a sample, thus providing a three-dimensional image. SEM is advantageous for studying the topography and composition of surfaces. Its unique feature is the ability to produce images with great depth of field, allowing for detailed three-dimensional representations of structures. On the downside, it typically requires samples to be coated with a conductive material, which may interfere with some properties of biological samples.
Scanning Probe Microscopes
Scanning probe microscopes provide high-resolution images by scanning a probe over the specimen's surface, measuring interactions at the atomic or molecular level. These microscopes have transformed areas such as nanotechnology and materials science by enabling measurements that were previously unachievable.
Atomic Force Microscopy
Atomic force microscopy (AFM) employs a cantilever with a sharp tip that interacts with the surface of the sample. It is advantageous for imaging surfaces at the molecular and atomic levels, providing detailed topographical maps. The key characteristic of AFM is its ability to analyze mechanical properties, such as stiffness and adhesion, of materials at the nanoscale. However, one limitation is the slower scanning speed compared to other techniques, which may hinder the observation of dynamic processes.
Scanning Tunneling Microscopy
Scanning tunneling microscopy (STM) uses a sharp tip that scans very close to the surface and measures the tunneling current between the tip and the sample. This technique is particularly important in studying conductive materials and surfaces at the atomic level. Its significant advantage is that it provides atomic resolution images and can be used for manipulating atoms directly. However, STM is limited to conductive materials, which restricts its use for many biological samples.
Digital Microscopy
Digital microscopy combines traditional microscopy techniques with digital imaging technology. This integration allows for faster image capture, manipulation, and analysis, which enhances overall productivity in research settings. One key aspect is the accessibility of high-quality imaging and analysis software that aids in data collection and interpretation. Nonetheless, the reliance on digital technologies may introduce issues related to calibration and image processing, affecting measurement accuracy.
Applications of Microscopy in Science
Microscopy is central to many scientific fields, allowing researchers to explore beyond the limits of the naked eye. Each application of microscopy brings unique insights and advantages that enhance our understanding of natural phenomena. This section discusses various applications of microscopy in science, focusing on biological research, materials science, and chemistry.
Biological Research
Biological research is one of the most critical applications of microscopy. It allows scientists to delve into the complexities of life at microscopic levels.
Cell Biology
Cell biology investigates the structure and function of cells, the fundamental units of life. Microscopy has revolutionized this field by providing detailed insights into cellular components like organelles. The use of techniques such as fluorescence microscopy enables researchers to tag specific cells and visualize dynamic processes in real time. The ability to observe cells has made microscopy a pivotal tool for understanding cellular behavior, disease pathology, and treatment efficacy.
Microbial Studies
Microbial studies hinge on the ability to observe microorganisms such as bacteria, viruses, and fungi. Techniques like scanning electron microscopy provide high-resolution images that allow for detailed examination of microbial structures. This observation aids in pathogenicity research, contributing to our understanding of infectious diseases. Microscopy allows for real-time monitoring and provides insights into microbial interactions, making it an invaluable tool for microbiology.
Histology
Histology involves the study of tissues at a microscopic level. It is essential for understanding the organization and function of various biological systems. Microscopy allows for the examination of tissue samples, revealing vital information about cellular composition and pathology. Techniques such as tissue staining enhance visibility and contrast, highlighting specific structures within tissues. Histology is crucial in medical and biological research, offering insights into health and disease.
Materials Science
The field of materials science benefits greatly from microscopy, as it allows for examination at the atomic and molecular levels.
Nanotechnology
Nanotechnology focuses on manipulating matter at the nanoscale, where properties differ significantly from bulk materials. Microscopy techniques such as atomic force microscopy provide detailed surface topographies, allowing researchers to study materials with precision. Understanding these nanoscale structures can enhance performance in various applications, from electronics to medicine. The unique feature of nanotechnology is its ability to tailor materials for specific functions, presenting both advantages and challenges in terms of safety and usability.
Metallurgy
Metallurgy studies the physical and chemical behavior of metallic elements and their alloys. Electron microscopy provides insights into microstructural properties that influence the mechanical performance of metals. This capability is critical for applications such as aerospace and automotive industries, where material properties directly impact safety and efficiency. The challenge lies in the interpretive skills required to correlate microstructure with performance.
Polymer Studies
Polymer studies focus on the properties and behaviors of polymers, which are vital in manufacturing and product design. Microscopy techniques can reveal how polymers interact at molecular levels, influencing properties like strength, flexibility, and thermal stability. For instance, scanning electron microscopy can illustrate the morphology of polymer blends. Although microscopy provides critical insights, challenges include the need for careful sample preparation to avoid artifacts that can misrepresent polymer behaviors.
Chemistry
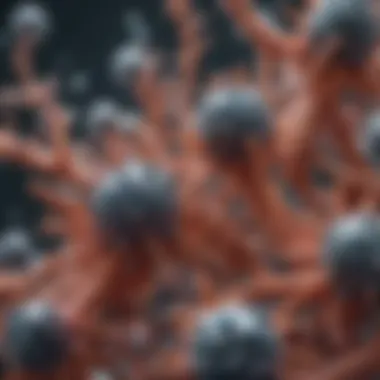
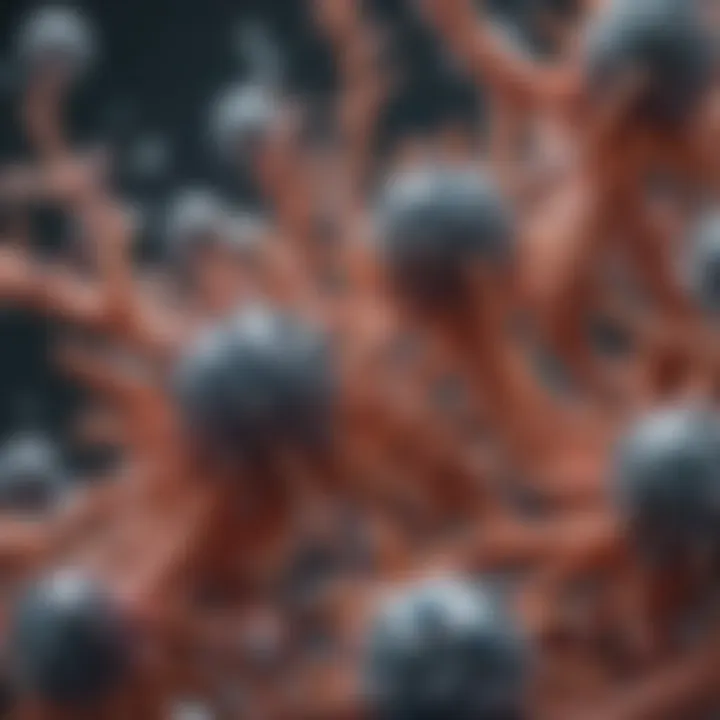
Microscopy plays a significant role in advancing chemical research, particularly in understanding molecular structures and reactions.
Chemical Analysis
Chemical analysis involves techniques that determine the composition and structure of substances. Advanced microscopy allows for high-resolution imaging of chemical compounds within a sample. Such details enable chemists to develop and optimize reactions more effectively. The challenge remains in correlating microscopic observations with macroscopic properties in chemical systems.
Crystallography
Crystallography studies the arrangement of atoms in crystalline solids. Microscopy aids in determining crystal structures, which are vital for understanding material properties. Techniques like X-ray microscopy can visualize the internal structure and morphology of crystals, enhancing our understanding of phase transitions and material functions. One disadvantage is the technical complexity involved in analyzing and interpreting the resulting data.
Surface Chemistry
Surface chemistry focuses on chemical reactions occurring on surfaces, significantly impacting catalysis and sensor development. Microscopy allows for the examination of surface characteristics and interactions, which is essential for innovations in chemical and material sciences. This insights can lead to advances in energy storage and environmental applications, although variability in surface behavior can complicate results.
Microscopy continues to be a transformative tool across scientific fields, providing critical insights that reshape our understanding of various phenomena. Its applications serve as testament to the vast possibilities of scientific exploration.
In summary, microscopy extends beyond just observation; it facilitates discovery and innovation in biological research, materials science, and chemistry.
The Role of Microscopy in Education
Education plays a pivotal role in shaping future researchers and professionals in various scientific fields. Microscopy serves as a fundamental tool in this process, offering students hands-on experiences that bridge theoretical knowledge with practical application. Understanding the intricate details of microscopy enhances critical thinking and analytical skills among learners. It opens pathways to explore the microscopic world, leading to discoveries that are essential in science and technology.
Learning Tools
Microscopes act as vital learning tools in educational settings. They provide a tangible way for students to engage with concepts they study in textbooks. For instance, in biology classes, using a microscope allows students to observe cell structures directly, reinforcing lessons on cell theory and functions. Through these observations, they see cell division, the organization of tissues, and other intricate processes firsthand.
Also, various types of microscopes can be integrated into the curriculum based on the educational level and subject matter. Optical microscopes are typically used in primary and secondary education, while advanced students might utilize electron or atomic force microscopes in specialized labs. This graduated exposure enables students to build upon their knowledge progressively.
Incorporating digital microscopy further enhances the learning experience. Students can capture images and analyze data more effectively, promoting a deeper understanding of their experiments. The ability to document findings digitally encourages engagement and fosters a collaborative learning environment where students can share insights and results easily.
Laboratory Practices
Laboratory practices are an essential component of scientific education, and microscopy is central to many experimental protocols. Students learn not only how to operate various microscopes but also how to prepare samples and observe phenomena accurately. This hands-on training is crucial, as it cultivates laboratory skills that are vital for future research.
Moreover, engaging with microscopy in lab environments instills a sense of precision and attention to detail. Students develop skills in documenting observations, drawing conclusions based on data, and troubleshooting potential issues with samples or equipment. These experiences are intertwined with safety protocols and ethical responsibility in scientific practice, making them an integral part of the educational journey.
Recent Advances in Microscopy
The field of microscopy has witnessed significant advancements that enhance its utility in scientific research. These developments not only improve the quality of images but also enable greater insight into complex biological and materials science questions. Recent innovations are pivotal in refining research methodologies, making it essential to understand their implications on scientific discovery.
Super-Resolution Microscopy
Super-resolution microscopy has emerged as a groundbreaking technique, allowing scientists to visualize structures at the molecular level. Traditional optical microscopy has limitations imposed by diffraction, making it challenging to resolve features smaller than approximately 200 nanometers. This is where super-resolution techniques, such as STED (Stimulated Emission Depletion) and PALM (Photoactivated Localization Microscopy), step in.
These methods bypass diffraction limits through various clever mechanisms, thus offering unparalleled resolution. For example, STED uses fluorescent molecules to control the emission of light and enhance image detail significantly.
The benefits of super-resolution microscopy are numerous:
- Detailed Visualization: It makes it possible to observe cellular structures and processes in fine detail, which is crucial in fields like cell biology and neuroscience.
- Tracking Dynamics: Super-resolution allows for real-time observation of proteins within cells, providing insights into their interactions and functions.
- Wide Applications: Researchers can employ this technology in cancer research, understanding protein interactions, and even examining viral structures.
However, these advancements do come with considerations. The need for complex setups and potentially expensive equipment may limit accessibility for some research institutions. Additionally, the interpretation of images requires specialized knowledge, as the results may sometimes lead to misinterpretation if not analyzed thoroughly.
Live Cell Imaging
Live cell imaging is another major advancement in microscopy that has transformed how scientists study dynamic biological processes. This technique enables researchers to visualize living cells in real-time, observing processes such as cell division, movement, and interaction with their environment.
The significance of live cell imaging lies in its ability to capture the dynamic nature of life. With conventional microscopy methods, researchers often relied on fixed cells, limiting their understanding of cellular behavior under physiological conditions. Live cell imaging eliminates this barrier by providing:
- Real-Time Data: This technique allows researchers to see how cells change and respond over time, offering insight into processes such as migration and signaling.
- Enhanced Interactivity: Researchers can manipulate environmental conditions while observing cell responses, leading to richer experimental designs.
- Applications in Various Fields: This method is extensively used in fields like cancer research, developmental biology, and pharmacology, helping to understand disease mechanisms and drug effects.
While live cell imaging provides substantial advantages, there are challenges associated with it, too. The need for specialized fluorescent markers can complicate experiments, and maintaining optimal conditions for cells can be demanding. Furthermore, the phototoxicity caused by prolonged exposure to light can alter cellular behavior and affect data integrity.
"The importance of these recent advances in microscopy cannot be overstated, as they enable us to ask new questions and explore biological phenomena with unprecedented detail."
The convergence of these innovative methods highlights a promising horizon for future research in various scientific fields. By integrating advanced microscopy techniques, researchers can push the boundaries of knowledge, making significant strides in the understanding of the microscopic world.
Challenges and Limitations of Microscopy
Microscopes play a crucial role in scientific discovery, but they are not without their challenges. Understanding these difficulties helps researchers and educators to better navigate the limitations while using this critical tool. Recognizing the constraints assists researchers in designing efficient experiments and aids educators in teaching effective microscopy techniques.
Technical Limitations
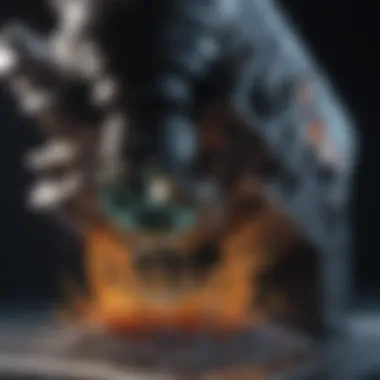
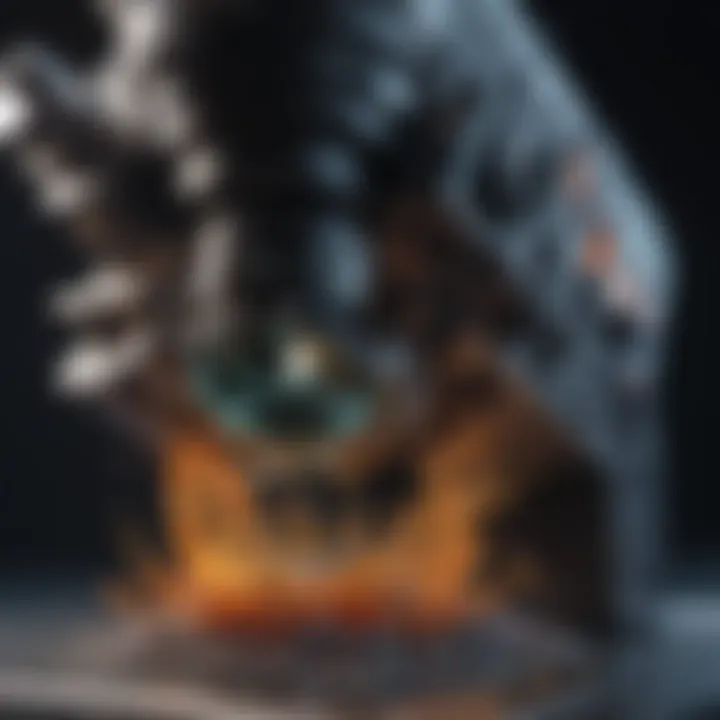
Every type of microscope has specific technical limitations that can impact the results obtained from experiments. For example, optical microscopes require visible light, which restricts the resolution to about 200 nanometers. Such a limit can prevent the observation of smaller structures, such as proteins and viruses. Electron microscopes, while powerful, come with their own issues. They often require samples to be in a vacuum and may damage delicate specimens.
Additionally, imaging techniques like Confocal Microscopy offer improved resolution, but at the cost of increased complexity and necessary expertise to analyze the results. The setup process can often be time-consuming, necessitating a considerable amount of skill. Some limitations include:
- Sample Thickness: Thick samples can lead to light scattering, resulting in unclear images.
- Field of View: The field of view may be restricted in high-magnification setups.
- Image Artifacts: Artifacts can distort images, complicating analysis and interpretation.
Despite these technical challenges, advancements in microscopy technology continue to emerge, addressing some of these limitations.
Interpretation Challenges
Interpretation of microscopic images presents another significant challenge. The clarity of the image is only one aspect of the analytical process. Researchers must often deal with complex data sets that require an in-depth understanding of both the visual material and the biological context. Misinterpretations can lead to incorrect conclusions about cellular structure or interactions.
Furthermore, quantifying information from microscopic images can be subjective. Different researchers might derive different meanings from the same image. Machine learning and artificial intelligence can assist in image analysis, but they can also introduce their own biases and limitations. Considerations include:
- Subjectivity: Interpretation can vary among scientists, affecting repeatability.
- Biological Variability: Biological samples may vary between experiments, complicating comparisons.
- Software Dependence: Reliance on specific software tools for analysis can create discrepancies in data interpretation.
"Addressing the challenges of interpretation is as vital as improving the technical aspects of microscopy itself. Accurate data analysis can lead to better discoveries."
Future Trends in Microscopy
The future of microscopy is poised to introduce significant advances that will shape scientific disciplines further. As research demands grow, the necessity for enhanced imaging techniques becomes more critical. Current trends indicate a shift towards integrating artificial intelligence and improving access to microscopy. These innovations are not merely technical advancements; they hold the potential to revolutionize research methodologies and outcomes, allowing scientists to analyze data with greater efficiency and accuracy.
Integration with AI
The incorporation of artificial intelligence in microscopy represents a pivotal shift in scientific research. AI algorithms can analyze vast amounts of imaging data far quicker than humans can. This capability enhances the interpretation of complex images, such as those produced in live cell imaging or high-resolution electron microscopy. AI assists in segmenting images, identifying patterns, and even predicting biological outcomes based on observed data.
For instance, machine learning models can rapidly classify cells in a sample, facilitating a quicker understanding of biological processes. This integration not only speeds up research but also enables more precise results. The tools designed to interface with microscopy systems include neural networks, which are becoming popular for their ability to learn from datasets and improve their analysis over time.
Moreover, AI can also help to reduce human error in data interpretation. The accuracy of scientific research often hinges on the minutiae visible only under a microscope, and AI provides a safeguard against misjudgments that may arise from fatigue or oversight.
Expanding Accessibility
Another prominent trend is the expansion of accessibility to microscopy technology. Historically, advanced microscopes came with high costs and complex operating procedures, limiting their use to well-funded laboratories. However, there is a push towards creating more user-friendly and affordable systems.
Open-source projects and initiatives aim to democratize access to microscopy tools. This change means that educational institutions and smaller research labs can utilize high-quality imaging capabilities previously unavailable to them. By collaborating through platforms such as Reddit and various educational forums, researchers share resources and knowledge, thereby fostering an inclusive scientific community.
Innovation is also seen in mobile microscopy, allowing researchers to capture and analyze samples on-site rather than relying solely on centralized labs. This flexibility can lead to significant advancements in fields like environmental science, where immediate results are essential for timely decision-making.
"The future of microscopy is not just in the advancement of technology, but in making that technology accessible to all who wish to advance scientific understanding."
By bridging the gap in accessibility and integrating AI, the field of microscopy is on the brink of a transformative era that promises to enhance discovery and innovation across various scientific realms.
Culmination
Recap of Microscopy's Impact
Microscopes have revolutionized the field of science by enabling researchers to observe structures that were previously beyond reach. The introduction of various microscopy techniques has facilitated significant breakthroughs in disciplines ranging from biology to material science. For instance, optical microscopes allow for the exploration of cellular structures, while electron microscopes provide insights into atomic arrangements. Each advancement in microscopy has opened new avenues for research and has led to discoveries that have shaped scientific paradigms.
Key impacts to consider:
- Enhanced resolution and magnification have placed previously unknown details within view.
- A variety of microscopy methods cater to specific research needs, maximizing exploration potential.
- The integration of digital technologies has transformed data collection and sharing, promoting collaborative research.
The Ongoing Importance of Microscopy in Science
The significance of microscopy in science is not waning; rather, it is transforming in the face of emerging technologies. As research questions become more complex, the demand for innovative microscopy techniques grows. These instruments remain vital in understanding diseases, materials properties, and the fundamental nature of substances.
Moreover, the incorporation of artificial intelligence presents opportunities for image analysis and data interpretation that were not feasible before, increasing efficiency and accuracy.
In summary, the absence of microscopy would leave a considerable gap in scientific research. Thus, its ongoing importance cannot be overstated as it continues to be a driving force behind significant advancements in various scientific disciplines.
Importance of References
Including well-curated references enhances the credibility and reliability of the article. It allows authors to back up their assertions with empirical evidence, ensuring that the assertions are based on scientifically verified information. This is vital in an area such as microscopy, where techniques and interpretations can rapidly evolve with new technology and findings. Key references might include seminal papers in microscopy, current research studies, and reviews published in respected scientific journals.
Benefits of Accurate Referencing
- Academic Rigor: It demonstrates adherence to scholarly standards, attracting an audience that values precision and validation in scientific literature.
- Resource for Further Reading: References act as a foundation for continued exploration. Readers can follow available literature to gain deeper insights into specific aspects of microscopy.
- Facilitate Collaboration: Quoting existing literature fosters an environment for future research, encouraging professionals and students alike to engage with past work while contributing new perspectives.
Considerations on Citations
When referencing in the document, specific attention must be given to recent studies reflecting advancements in the field. The citation style should remain consistent throughout the article, allowing for clarity in recognizing sources.
In the context of the present discussion, references such as articles from Nature, Science, and Microscopy Research and Technique provide essential insights. Using integrated databases like PubMed or resources from Wikipedia can also lend valuable information to support the narrative.
"References are the spine of scholarly work, giving form and structure to the claims that fill its pages."
In summary, the references section is integral for fostering trust, enhancing scholarly communication, and enriching the educational aspect of the article. They not only substantiate discussions but also inspire further inquiry into the multifaceted world of microscopy.