Exploring Light Quantum Theory: Foundations and Implications
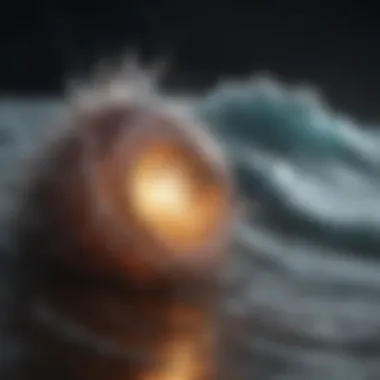
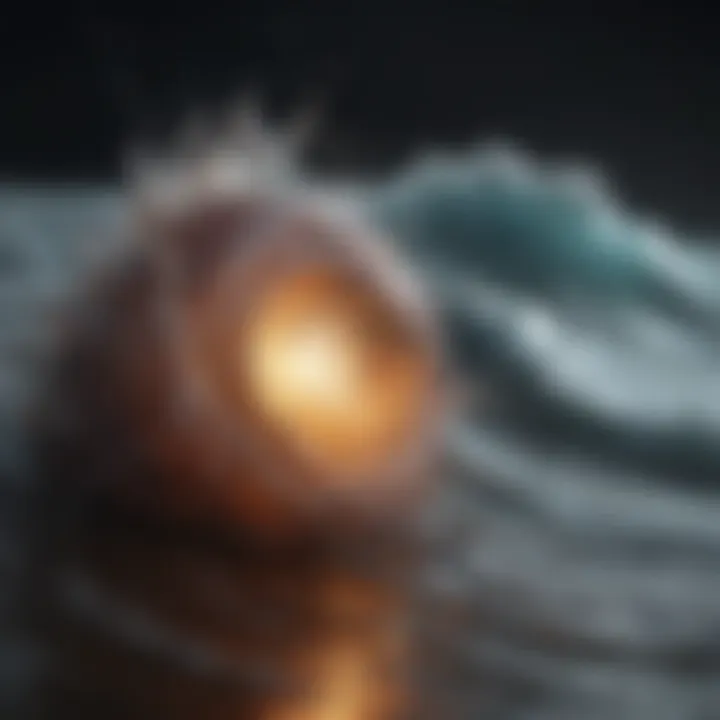
Intro
Light, often seen as a mere source of illumination, carries secrets that go beyond its visible spectrum. Ever since ancient philosophers contemplated what light was made of, it has sparked curiosity and debate. However, it wasn't until the early 20th century that the nature of light was cast under a new lens – quantum mechanics. The emergence of light quantum theory brought forth a paradigm shift, challenging the classical understanding of light as a wave or particle alone. Instead, it revealed that light possesses a dual nature, demonstrating characteristics of both waves and particles.
This article embarks on a thorough exploration of light quantum theory, peeling back the layers of history, principles, and implications. We will traverse the historical pathways that led to its development, delve into core experiments that brought key concepts to light, and discuss how these principles revolutionize modern physics.
The implications of light quantum theory extend far beyond theoretical musings; they resonate through contemporary research and advance technology in ways that impact daily life. From the development of lasers to quantum computing, the insights provided by this theory continue to influence a diverse array of fields. By examining how light interacts with matter, we highlight its foundational relevance to the fabric of the universe.
Prepare for a dive into the world where light behaves unexpectedly, affording a glimpse into a reality far stranger than we might initially presume.
Preamble to Light Quantum Theory
The realm of quantum mechanics often appears as a labyrinth, and within this maze lies the fascinating terrain of light quantum theory. Understanding this concept is not only crucial for physicists but also for anyone intrigued by the nature of reality. Light quantum theory bridges diverse fields, from fundamental physics to cutting-edge technology. Grasping its principles provides insight into how matter and energy interact, fundamentally transforming our understanding of the universe.
Defining Light Quantum Theory
Light quantum theory fundamentally posits that light exhibits both particle and wave characteristics, a dual nature that challenges our classical intuitions. At its core is the concept of the photon, a discrete packet of energy that travels at the speed of light. By viewing light in quantum terms, scientists can explore its behaviors in ways that classical theories fail to address.
For instance, say you're shining a flashlight in a dark room. In classical physics, one might simply view this as a ray emanating from a source. But with light quantum theory, that same flashlight beam is analyzed as a stream of photons, each behaving independently while collectively creating a coherent wavefront. This distinction is not just academic; it leads to real-world explanations and predictions across a multitude of disciplines—from optics to telecommunications.
Historical Context and Development
The genesis of light quantum theory traces back to the early 20th century when the implications of electromagnetic theory began to clash with experimental observations. Albert Einstein's revolutionary work on the photoelectric effect in 1905 marked a turning point. He proposed that light could be thought of as quantized, effectively laying the groundwork for quantum mechanics.
In the following decades, contributions from other physicists, including Max Planck and Niels Bohr, helped to shape our understanding further. Planck's introduction of energy quanta in 1900 and Bohr's model of the atom showcased how light interacted with matter in unexpected ways, relying on this quantum view.
"The theory of quanta is more important than the atomic theory itself."
— Max Planck
As these ideas matured, they opened new avenues for exploration. The idea that light could exist both as a wave and as a particle—itself a concept still debated—gave rise to numerous experiments and applications, from lasers to quantum computing.
Thus, the historical evolution of light quantum theory not only sets the stage for modern physics but also signifies a paradigm shift in how we comprehend the universe, blending together elements of mystique and clarity alike.
Fundamental Concepts of Light Quantum Theory
The discussion of light quantum theory hinges on a few essential concepts that not only deepen our understanding o f the nature of light but also lay the groundwork for its implications in various fields such as technology, philosophy, and foundational physics. These core ideas serve as the building blocks for comprehending how light exhibits both particle-like and wave-like behaviors, as well as the implications of these behaviors in the quantum domain.
There are several crucial elements to explore:
- Wave-Particle Duality
- Photons and Their Properties
- Quantum States of Light
Together, these ideas craft a rich tapestry that illustrates the duality and complexity inherent in the study of light, drawing attention to how these elements converge in leading theories of quantum mechanics. Understanding these foundations is essential for appreciating advanced topics, including technological applications and philosophical inquiries that stem from light quantum theory.
Wave-Particle Duality
Wave-particle duality is a cornerstone of quantum mechanics and plays a pivotal role in light quantum theory. This concept illustrates how light is not limited to being just a wave or just a particle; rather, it embodies characteristics of both depending on the experimentation context.
To visualize this, think about tossing a pebble into a pond. The ripples that form exemplify the wave nature, while a pinprick of light shone through a prism displays its particle-like characteristics. This dual nature is necessary for explaining phenomena such as interference patterns seen in diffraction experiments and the discrete impacts of photons in the photoelectric effect.

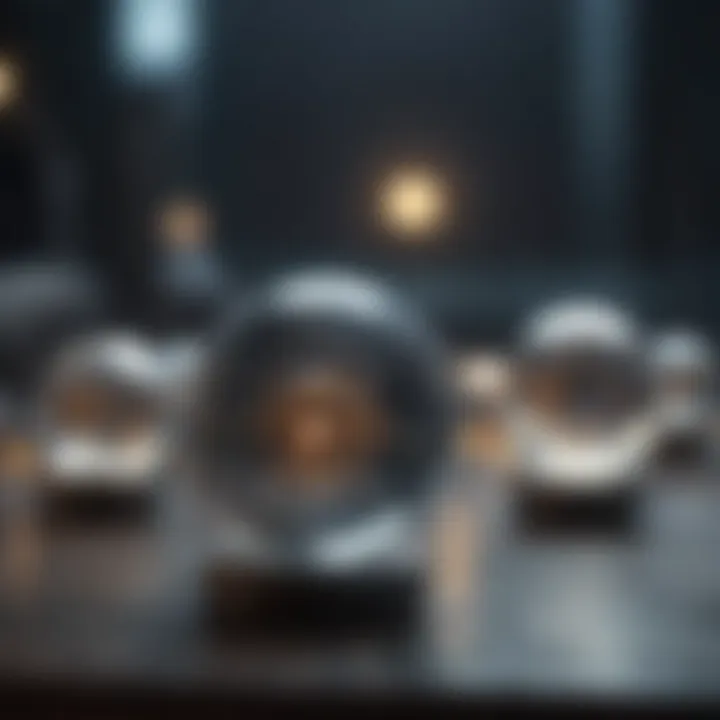
Importantly, it forces a reevaluation of how we perceive physical reality. Is it possible that light, and other quantum entities, exist in a state that does not conform to classical definitions? The answer, as numerous experiments indicate, leans towards a profoundly nuanced understanding of physical behavior—one that intertwines certainty with uncertainty.
Photons and Their Properties
Photons—those elementary particles of light—are unique entities with properties that set them apart from other particles.
- Massless: Photons possess zero resting mass, allowing them to travel at the fastest speed in the universe: that of light.
- Charge Neutral: They are electrically neutral, which helps maintain electromagnetic harmony in the cosmos.
- Quantum Behavior: As quantum objects, photons can exist in overlapping states and can interact with matter in both expected and unexpected ways.
These characteristics are paramount in fields like telecommunications, where photons are harnessed to transmit information through fiber optics at astonishing speeds. The idea that photons can behave like particles while simultaneously exhibiting wave properties forms a basis for various technologies, such as lasers and quantum cryptography.
Quantum States of Light
In light quantum theory, quantum states describe the probabilities of a photon's position, momentum, and other attributes. Each photon can exist in a multitude of possible states until it is measured. This notion of superposition—that a light particle can be in many states at once—captures the complex, highly non-intuitive world of quantum phenomena.
Furthermore, phenomena such as entanglement arise from these quantum states, linking photons in ways that challenge classical separateness. Consider a pair of entangled photons. Observing one instantly informs you about the state of the other, no matter the distance separating them. This intertwining relationship adds layers to the philosophical implications of quantum mechanics, hinting at a more interconnected universe.
In summary, grasping these fundamental concepts forms the bedrock of light quantum theory. They unveil layers of scientific understanding that influence multiple domains while encouraging a reevaluation of established principles in physics, technology, and even philosophy.
Key Experiments in Light Quantum Theory
Understanding the pivotal experiments that shaped light quantum theory is crucial. These experiments are the bedrock upon which much of modern physics is built. Through them, we gain insights into the fundamental principles of light, a varied and complex entity that behaves like both a wave and a particle. Each experiment sheds light, pun intended, on different aspects of this duality, providing substantial evidence that has altered our perspective on reality itself.
The Photoelectric Effect
The photoelectric effect is a phenomenon where electrons are emitted from a material when it is exposed to light. This was a real eye-opener for the physics community in the early 20th century. Albert Einstein's explanation of this effect in 1905 was monumental. He proposed that light exists in quantized packets called photons, each possessing energy directly proportional to its frequency. To put it simply, not all light has the same energy.
To understand its significance:
- Evidence of Particle Nature: This phenomenon demonstrated that light exhibits particle-like properties. Before this, light was mostly viewed as a continuous wave.
- Threshold Frequency: There exists a minimum frequency (or threshold) of light needed to cause electron emission, showing that frequency, not intensity, matters.
- Implications for Quantum Theory: The photoelectric effect helped cement the idea of light quantum theory and gave rise to further research in quantum mechanics. It encouraged scientists to explore more about the interrelationship between light and matter.
As noted in the initial studies: > "Each photon can dislodge an electron, changing the way we understand energy interactions at Quantum levels."
Young's Double-Slit Experiment
Young’s double-slit experiment is a classic test that reveals the wave-particle duality of light. Conducted by Thomas Young in the early 1800s, it showed that light could produce an interference pattern, indicating its wave-like behavior. The setup is simple in theory—shine light through two closely spaced slits and observe the pattern on a screen behind the slits.
The key takeaways from this experiment include:
- Interference Pattern: When light passes through the two slits, it creates alternating bright and dark bands on the screen, characteristic of wave interference.
- Observation Effect: When detectors were placed on the slits to observe which slit the photons traveled through, the interference pattern disappeared. This suggests that the act of observation collapses the wave function, leading to particle-like behavior.
- Fundamental Questions: This experiment raises questions regarding reality and observation in quantum mechanics—whether particles exist in a definite state independent of observation.
Compton Scattering
Discovered in 1923 by Arthur Compton, Compton scattering pertains to the behavior of X-rays when they collide with electrons. This experiment not only validated the particle nature of light but also showcased how photons transfer energy and momentum.
Essential points about Compton scattering:
- Photon-Electron Interaction: It demonstrated that when X-rays collide with electrons, they impart some energy to electrons, resulting in longer wavelength X-rays after the collision.
- The Concept of Momentum Transfer: Compton's findings supported the theory that light behaves as a particle, possessing momentum.
- Quantitative Analysis: The experiment led to Compton's formula, offering a mathematical framework to predict these changes, making it a critical point for further quantum research.
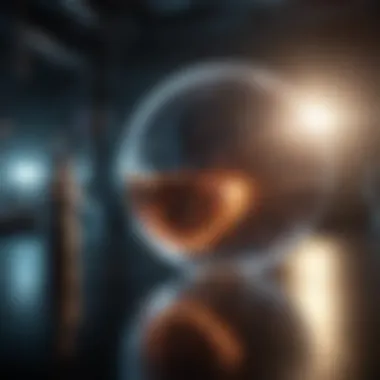
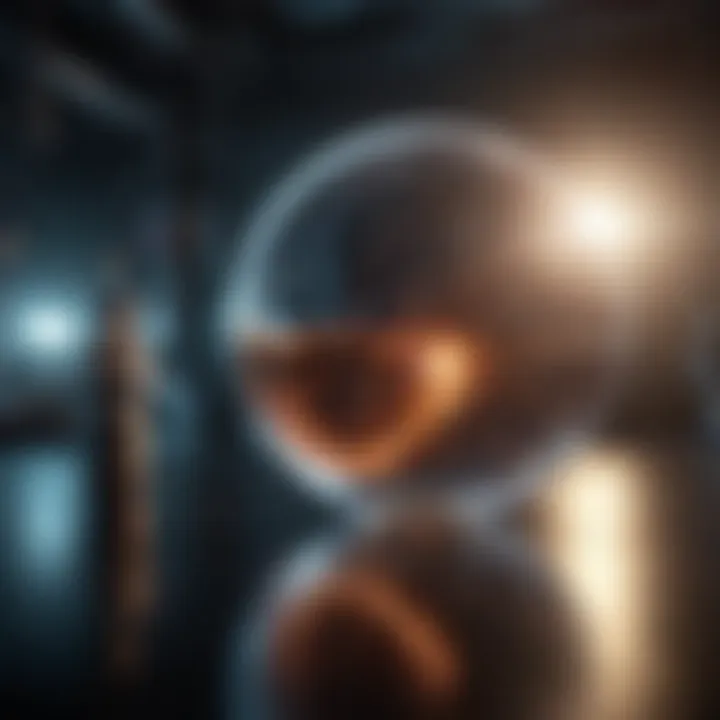
Bell's Theorem Experiments
Bell's theorem marks a monumental chapter in light quantum theory, especially in the realm of quantum entanglement. Formulated by physicist John Bell in the 1960s, the theorem presents an inequality that tests the predictions of classical physics against those of quantum mechanics.
Important insights include:
- Test of Local Realism: Experiments testing Bell's inequalities often reveal that particles can be correlated in a way that seems to contradict local realism, suggesting an inherent 'spookiness' of quantum mechanics.
- Choice of Measurement: The results depend on the choice of measurement settings, which creates a debate about causality in quantum physics.
- Technological Implications: These findings have implications for quantum computing and cryptography, pushing the boundaries of how we utilize quantum entanglement in modern technology.
In summary, the key experiments within light quantum theory present compelling evidence that challenges our traditional understanding of physics. From the simple yet profound photoelectric effect to the mind-bending implications of Bell's theorem, each experiment not only enriches our scientific understanding but also prompts deeper philosophical inquiries about nature and observation.
Mathematical Framework of Light Quantum Theory
In the realm of quantum mechanics, mathematics serves as a bedrock, providing a robust structure for understanding the perplexing behaviors of light. The mathematical framework of light quantum theory is essential, as it allows scientists and researchers to effectively describe phenomena that challenge classical physics. This framework encompasses several vital components, which articulate how light interacts with matter and embodies its dual wave-particle nature.
Wave Functions and Superposition
A fundamental component of this framework is the concept of wave functions. Essentially, a wave function is a mathematical description that encapsulates the probabilistic state of a quantum system, which in this case refers to light. It conveys the likelihood of finding a photon at a specific location and time. The superposition principle goes hand in hand with wave functions. It posits that quantum states can exist simultaneously in multiple configurations. For example, a photon can be in several pathways before it's measured or observed, leading to the intriguing phenomenon known as interference. This duality fundamentally shifts our understanding of reality, highlighting that light does not merely occupy a single space; it can be everywhere at once until observed.
"In quantum mechanics, observing a phenomenon actually alters the state being observed. This is as baffling as it gets, yet it lays the groundwork for much of modern physics."
Quantum Mechanics Equations
The mathematical formulation of light quantum theory introduces various quantum mechanics equations, which mathematically illustrate the behavior of light. The Schrödinger equation, for instance, is central. It describes how the quantum state of a physical system changes over time. In the context of light, these equations help predict what happens under different conditions, thus offering insights into complex interactions, such as those found in lasers and optical communication.
Moreover, the Maxwell’s equations relate to how electromagnetic waves propagate. By successfully marrying these two sets of equations, researchers can comprehensively model how light behaves under various scenarios, paving the way for advanced technological applications.
Density Matrices and Quantum States
The use of density matrices has transformed how we interpret quantum states over time. Unlike pure states, which offer complete information, density matrices allow for the representation of mixed states, thereby providing a statistical description of systems that cannot be adequately defined by a single wave function. This approach becomes especially significant in understanding the phenomena of entanglement and decoherence, where light interactions create intertwined quantum states. By employing density matrices, researchers can delve deeper into the complexity of these interactions, identifying practical implications such as in quantum computing or secure communication techniques.
In summary, the mathematical framework of light quantum theory not only supports the theoretical analysis of light’s behavior but also lays down the groundwork for practical innovations. By embracing wave functions, quantum mechanics equations, and density matrices, we can begin to fathom the intricate tapestry of space, time, and light itself.
Implications of Light Quantum Theory
Light quantum theory stands as a cornerstone of modern physics, echoing through various fields and laying foundational stones for cutting-edge technology and thought. The implications of this theory stretch far beyond mere academic curiosity; they manifest in real-world applications, influence philosophical debates, and shape the trajectory of scientific inquiry.
Technological Applications
One of the most striking aspects of light quantum theory is its transformative impact on technology. The principles derived from this theory have lead to practical applications that define and expand our technological horizons. Let's examine a few of the key areas:
- Lasers: These devices rely on the principles of stimulated emission, fundamentally tied to quantum mechanics. From cutting-edge surgery to data storage in DVDs, lasers have permeated multiple arenas.
- Photovoltaics: Solar panels, harnessing the photoelectric effect, convert sunlight directly into electricity. This technology not only promotes sustainable energy but also minimizes our carbon footprint.
- Quantum Cryptography: Here, the properties of light are exploited to create ultra-secure communication channels. The unique behavior of photons enables encryption that is theoretically unbreakable.
- Medical Imaging: Techniques like positron emission tomography (PET) use quantum phenomena for precise diagnostics, illustrating how deeply intertwined light theory is with human health.
As we navigate through these applications, it's crystal clear that light quantum theory doesn't remain confined to textbooks; it is a living, breathing concept that drives innovation and change.
Impact on Modern Physics
The philosophical and quantitative inquiries detailed in light quantum theory have profound implications in modern physics. Here's how it reshapes our understanding:
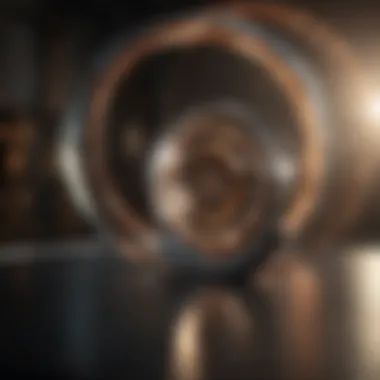
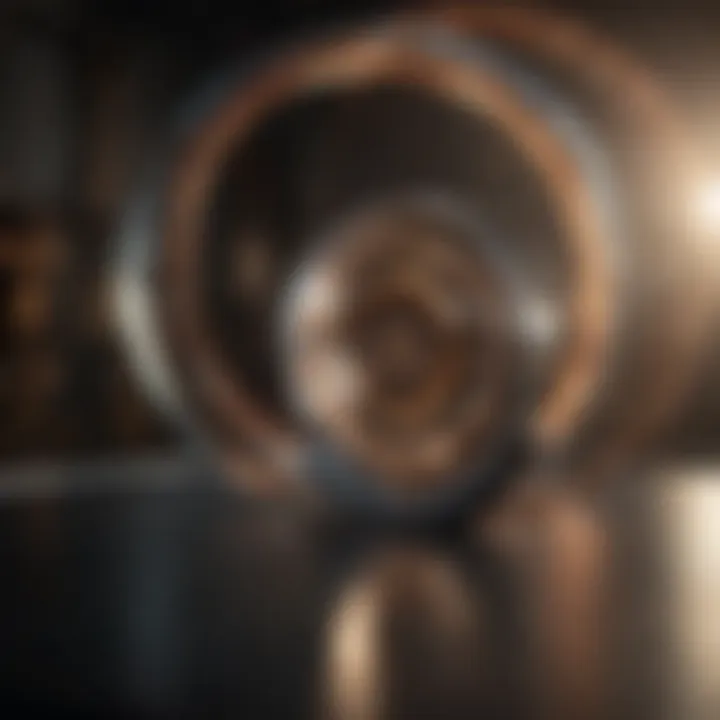
- Quantum Mechanics: The theory demands a reconceptualization of reality itself. Models of physical phenomena now incorporate both wave and particle characteristics, challenging classical notions.
- Uncertainty Principle: Heisenberg's principle, which arises from quantum mechanics, posits that certain pairs of physical properties cannot be simultaneously known to arbitrary precision, introducing a layer of complexity to scientific measurement.
- Quantum Entanglement: This phenomenon suggests that particles can be interconnected across vast distances, leading to new interpretations of causality and locality. Such phenomena not only challenge our understanding of philosophy but also pose groundbreaking potential for future technologies.
With these facets, it becomes evident that light quantum theory serves as a compass, guiding contemporary physicists through new realms of discovery and understanding.
Philosophical Considerations
The implications of light quantum theory also encroach upon the realm of philosophy, posing questions that challenge our longstanding assumptions about the universe:
- Nature of Reality: The dual nature of light as both wave and particle raises ontological questions. Is reality a fixed state, or is it reliant on observation, as suggested by aspects of quantum mechanics?
- Free Will: If the universe operates on probabilities rather than certainties, one may ponder how that interjects into the concept of free will. Are we merely players in a cosmic game where outcomes hinge on quantum fluctuations?
- Knowledge and Perception: The complexity introduced by quantum phenomena prompts dialogues about human understanding. How much can we truly know about the universe when uncertainty is etched into the very fabric of reality?
Current Research and Future Directions
The exploration of light quantum theory isn't just a historical journey; it is an ongoing adventure that unfolds in contemporary research. As we look ahead, the avenues emerging from this field reveal potential that could fundamentally alter technology and deepen our understanding of the universe. This section highlights prominent areas of investigation in light quantum theory, emphasizing their relevance for today and what they might mean for tomorrow.
Emerging Fields: Quantum Optics
Quantum optics stands as a cutting-edge arena where the principles of light quantum theory take an intriguing turn. It’s not merely about understanding light; it’s about manipulating it at the most elemental level. Researchers are honing techniques to generate and control quantum states of light, opening doors to previously unimaginable applications.
One fascinating aspect of quantum optics is the concept of entangled photons. These are pairs of photons that remain interconnected no matter the distance apart. The implications for quantum communication are monumental. Imagine a world where secure communication is guaranteed through the principles of quantum entanglement. This could revolutionize both digital security and information technology.
Furthermore, advancements in laser technology, specific to quantum optics, are pioneering developments in medical imaging and diagnostic tools. Techniques such as quantum-enhanced imaging are encouraging scientists to rethink conventional methods. This means clearer images, closer looks into biological systems, and more precise diagnostics. As various industries latch on to these advancements, the future of quantum optics stands as a beacon of possibility.
Light in Quantum Computing
The intersection of light and quantum computing is fraught with remarkable potential. Traditional computing, operating on binary systems, may soon be complemented or even replaced by quantum systems that leverage the properties of light. At the heart of this shift is the shift from classical bits to qubits, which encode complex states of information in subatomic particles.
In quantum computing, light plays several key roles. For instance, photonic qubits can be manipulated through optical gates and circuits to perform computations at extraordinarily high speeds. This not only offers faster processing capabilities but also enhances energy efficiency, a critical consideration in the modern era of massive data processing.
Moreover, ongoing research into quantum algorithms that utilize the unique features of light might lead to breakthroughs in solving complex problems unmanageable by classical computers. Notable examples include optimization problems in logistics, cryptography, and even complex simulations of molecular structures in pharmaceuticals.
Challenges and Open Questions
While the realms of quantum optics and quantum computing paint an exciting picture, tackling the underlying challenges is fundamental. One of the pressing questions is scalability. As researchers aim to develop more complex systems, the challenge lies in maintaining coherence in qubit states, particularly in light sources like lasers which may introduce noise.
Another significant area of inquiry involves the ethical ramifications of quantum technologies. The implications of quantum communication, especially in the context of privacy and security, require careful consideration. Moreover, as quantum technology becomes more ubiquitous, addressing issues such as equitable access and potential misuse will be crucial.
The road ahead is lined with questions, but they are avenues for exploration rather than obstacles. What kind of advancements can we expect in the next decade? How can we ensure that technology serves humanity? These ponderings can ignite fresh discussions across disciplines, ultimately guiding future research and innovation.
"The dialogue around light and its quantum applications showcases the intricate dance between technology and philosophy; each step revealing more about our universe and ourselves."
Finale
In wrapping up our exploration of light quantum theory, it’s essential to emphasize the significance of what we have covered. This intricate subject isn't just for physicists in lab coats peering through high-tech machinery; it's foundational to our understanding of the universe. Each concept presented in earlier sections builds a narrative that connects the past to the present while looking into the future.
Summarizing Key Insights
In the preceding sections, we have mapped out crucial insights into light quantum theory. Let’s break down these insights:
- Wave-Particle Duality: This foundational concept showcases the dual nature of light, illustrating how it can display characteristics of both waves and particles. Understanding this duality paves the way for grasping more complex interactions in quantum mechanics.
- Photons and Their Properties: The essence of photons being the quantum units of light complications yet enriches our understanding of light's nature. Their behavior hints at greater principles of energy and momentum, with implications across various technological frameworks.
- Key Experiments: Each groundbreaking experiment, from the photoelectric effect to Young's double-slit experiment, has not only validated theoretical viewpoints but also opened pathways toward modern technological advancements, such as fiber optics.
- Mathematical Framework: The incorporation of mathematical principles helps consolidate the theory's validity. Wave functions and quantum mechanics equations frame our understanding, allowing precision in describing quantum behaviors.
- Modern Implications: The ripple effect of light quantum theory in today's technology cannot be overstated. It underpins innovations in quantum optics and computing, spearheading drastic shifts in communication systems and computational power.
- Future Directions: Reflecting on current research steers us into discussions about emerging fields. The challenges faced in quantum experiments not only drive inquiry but also present opportunities to deepen our understanding of light and, by extension, the universe.
As we digest these key insights, it’s prudent to reflect on how light quantum theory reshapes not only scientific paradigms but also our philosophical approach towards physical reality. Quantum principles resonate in our everyday lives, manifesting in technologies we often take for granted.
"The greatest challenge is not to understand quantum theory, but to understand how it can change our understanding of everything."
In summarizing, light quantum theory stands as a testament to human curiosity and intellectual resilience. The interplay between theory and practice will likely continue to forge new pathways as we grapple with the seemingly paradoxical nature of existence.