Innovative Approaches to Scaffold-Free 3D Cell Culture
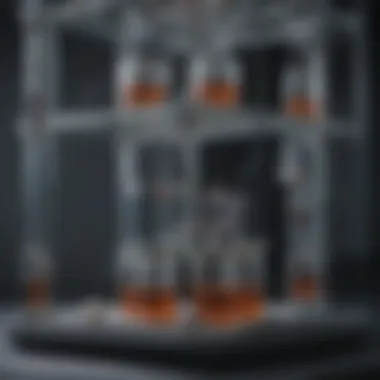
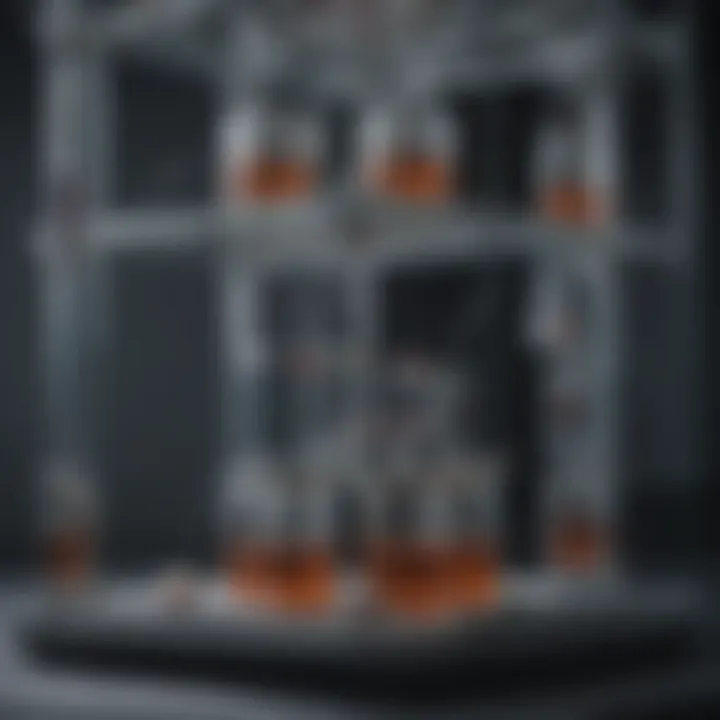
Intro
The push toward more realistic models in biological research has led scientists to explore scaffold-free 3D cell culture. This innovative approach holds the promise of mimicking the natural environment of cells more accurately than traditional methods, which often utilize artificial scaffolding. The importance of this transition canโt be overstated, as it potentially unlocks new avenues for understanding complex cellular behaviors and interactions.
In traditional 2D cultures, cells are often deprived of the necessary signaling and structural cues that influence their function in a living organism. This can lead to misleading results, particularly for studies focusing on drug response and disease modeling. By employing scaffold-free techniques, researchers aim to create a more physiologically relevant context that encourages cells to communicate, grow, and respond in a manner closer to that observed in vivo.
This article will explore the spectrum of scaffolding-free technologies, detailing methodologies ranging from bioprinting to microfluidic systems. We'll also shed light on its implications for drug discovery, tissue engineering, and regenerative medicine. Additionally, challenges that lie ahead in this field will be addressed. Through this exploration, we aim to provide a thorough overview of how scaffolding-free methods are transforming our understanding of cell biology and enhancing experimental outcomes.
Relevance
The shift towards scaffold-free cell culture is not just an academic exercise; it has real-world implications in numerous fields, including:
- Drug discovery: More accurate models lead to better predictions of how drugs will behave in humans.
- Tissue engineering: Improved methods can facilitate the creation of viable tissue for transplantation.
- Regenerative medicine: Insight into cell behavior paves the way for innovative therapies designed to repair damaged tissues.
"Scaffold-free 3D cell culture can revolutionize our comprehension of cell dynamics and the intricacies of tissue regeneration."
As we navigate this fascinating field, it is crucial to catch up with the latest trends, challenges, and breakthroughs that emerging technologies bring to the table.
Prelims to 3D Cell Culture
The field of 3D cell culture has emerged as a critical component in biological research, offering novel insights into cellular behavior and interactions that were previously overlooked in traditional methods. 3D cell culture models enable scientists to mimic the complex architecture of in vivo environments. This not only improves the physiological relevance of studies but also enhances the predictability of how cells respond to various stimuli and pharmacological interventions.
Given the increasing demand for more accurate models in drug testing and disease research, understanding how 3D cell culture works is paramount for several reasons. First, such cultures foster improved cell-cell interactions, a vital aspect when considering how cells communicate and function in real-life biological systems. This can lead to more representative data when testing therapeutic compounds.
Second, these models can better replicate the extracellular matrix of tissues, which is crucial in studying tissue development, regeneration, and repair. In essence, as research pushes forward, the relevance of 3D cell culture cannot be overstated.
Defining 3D Cell Culture
3D cell culture refers to techniques that allow cells to grow and interact in a three-dimensional environment, closely resembling their natural surroundings in vivo. This contrasts sharply with traditional 2D cultures, where cells are typically grown on flat surfaces, leading to altered cell behaviors due to the lack of spatial interaction and structural connectivity.
In scaffold-free cultures, methods such as self-organization or aggregation techniques are employed. Cells can cluster together and form spheroids or organoids, which promote more biologically relevant behaviors and physiological characteristics. The advent of various scaffold-free methodologies allows for greater flexibility and optimization in how researchers can develop and analyze these cell cultures.
Historical Context
The concept of cell culture began in the early 20th century, primarily using 2D cultures. Initial developments laid the groundwork for cellular studies, yet the limitations soon became apparent. Early researchers noted that cells grown in 2D did not resemble their natural environments, leading to a drive toward more complex models.
The evolution toward 3D cell culture gained momentum in the 1990s when researchers began exploring various techniques, including hydrogels and scaffold-based systems. As scientific understanding progressed, it became clear that harnessing the advantages of scaffold-free techniques could propel the field forward. This shift sparked a wave of innovation, culminating in todayโs diverse array of 3D culture methods.
Comparison with Two-Dimensional Cultures
When comparing 3D cell cultures to traditional 2D cultures, several key differences emerge.
- Cellular Behavior: Cells in 2D cultures tend to grow in layers and often exhibit modified behaviors, such as altered gene expression and reduced cell death. In 3D setups, interactions mimic those found in living organisms, enabling more relevant cellular responses.
- Microenvironment Mimicry: The arrangement in 3D cultures facilitates a more authentic microenvironment, influencing factors such as nutrient exchange, waste removal, and signaling pathways.
- Predictive Modeling: Data generated from 3D cultures can often provide greater predictability regarding human responses, especially in drug discovery and toxicology.
In light of these differences, itโs evident that 3D cell culture stands to offer significant advances over traditional methods, ultimately shaping the future of biological research.
The Concept of Scaffold-Free Cell Culture
In recent years, the concept of scaffold-free cell culture has emerged as a significant stride in the field of tissue engineering and regenerative medicine. Unlike traditional approaches that utilize various scaffolds to provide structural support to cells, scaffold-free techniques harness cellsโ innate ability to self-organize. This method aligns more closely with natural biological environments, enhancing the mimicry of in vivo conditions. This focus on cellular self-organization represents a paradigm shift, emphasizing not only the physical geometry but also the biochemical interactions required for optimal cell behavior.
Principles of Scaffold-Free Techniques
The principles underlying scaffold-free techniques hinge on the notion that cells can thrive when granted the freedom to form their own matrices. At the core of this methodology is the idea that cells naturally possess the capability to bind and communicate through secreted factors, leading to the formation of complex structures. Key elements to consider include:
- Cell Aggregation: In scaffold-free systems, cells often aggregate to form spheroids or organoids. These structures facilitate robust cell-cell interactions that are essential for functionality.
- Microenvironment Simulation: By removing scaffolds, researchers can better simulate the extracellular matrix. This allows for a more accurate representation of biomechanics and biochemical signals that cells encounter in their natural habitats.
- Cell Viability and Functionality: Studies have shown that scaffold-free cultures can enhance cellular viability and promote differentiated states resembling native tissue, which is crucial for applications in drug testing and regenerative therapies.
This liberation of cells to behave as they would in vivo not only fosters more authentic tissue architectures but also opens new doors for innovation in biomedical research.
Key Differences from Traditional Methods
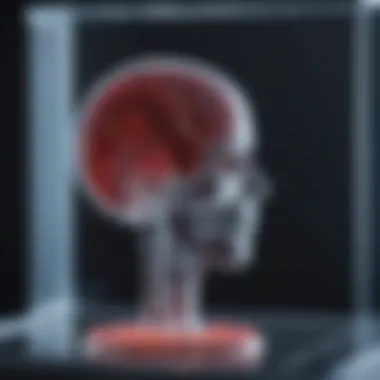
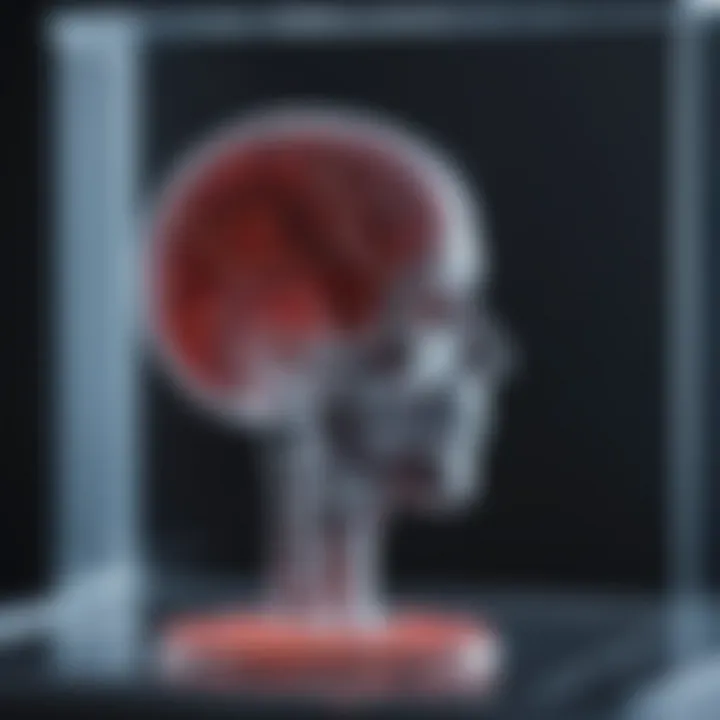
The distinctions between scaffold-free and traditional cell culture methods are as vast as the ocean. Traditional methods often involve using synthetic or natural materials to provide structure; however, this can sometimes limit the natural behavior of the cells. Key differences include:
- Artificial Constraints: In traditional methods, scaffolds may impose physical limitations, leading to a change in how cells communicate and interact. Scaffold-free techniques allow cells to self-regulate their shape and organization, promoting more natural behaviors.
- Variability in Response: With traditional scaffolds, the material properties can vary, leading to inconsistent cell responses. Scaffold-free cultures tend to produce more predictable outcomes due to their reliance on biological rather than synthetic materials.
- Application Range: While scaffold-based approaches are effective for some therapies, they can fall short in others, such as in complex tissue engineering or organoid development. Scaffold-free methods lend themselves more flexibly to a wider array of applications.
"Scaffold-free techniques are not just a trend but signify an essential evolution in how we think about cell behavior and interactions in culture."
Their capability to enhance modeling of significant biological processes makes them invaluable for contemporary research. In sum, the principles of scaffold-free techniques and their differentiation from traditional methods portray a compelling narrative of innovation tailored for researchers aiming to push the envelope in tissue engineering.
Technologies Enabling Scaffold-Free Cultures
Advancements in scaffold-free 3D cell culture rely heavily on various innovative technologies. These technologies have revolutionized how we approach cell growth and manipulate biological systems, greatly enhancing our ability to replicate the natural cellular environment. Ultimately, they provide significant benefits that help researchers probe deeper into cellular behavior, significantly influencing fields such as drug discovery and tissue engineering.
3D Bioprinting
Overview of 3D Bioprinting
3D bioprinting stands out as a game-changer, enabling the precise arrangement of cells and matrices in a desired configuration. This technique uses specialized printers to layer biomaterials and living cells, mimicking the intricate structures of natural tissues. One key characteristic of 3D bioprinting is its ability to customize the spatial distribution of cells, which is crucial for creating functional tissue models. This tailored approach has made it a popular option for researchers aiming to produce accurate representations of human tissues or organs. However, the quality of the printed structure can depend heavily on the printerโs resolution and the bio-inks used, creating challenges that must be navigated carefully.
Applications in Tissue Engineering
When it comes to tissue engineering, 3D bioprinting offers unprecedented potential. It allows for the creation of complex tissue structures that can potentially be implanted into patients for regeneration. The ability to fabricate organoidsโminiaturized and functional organ-like structuresโis a standout feature. This approach not only aids in drug testing but also opens up new possibilities for regenerative medicine. However, the scalability of producing these structures can present limitations, as the technology still faces hurdles related to vascularization and integration within the host organism.
Microfluidic Systems
Design and Implementation
Microfluidic systems represent another critical technology in scaffold-free 3D cell cultures. They involve the manipulation of fluids at the microscale, allowing for highly controlled environments where cellular interactions can be studied in real-time. A notable feature of these systems is their capacity to simulate the physiological flow of fluids, which can significantly affect how cells behave. The design of these systems can vary greatly based on the needs of the specific research, making it a highly adaptive choice for scientists. Nevertheless, complexities in fabrication and the need for precise controls can introduce challenges that require thoughtful consideration.
Cellular Behavior Insights
One of the profound benefits of integrating microfluidic systems in scaffold-free cultures is their capability to provide insights into cellular behaviors. By observing how cells respond in a controlled fluid environment, researchers can gain valuable information about signaling pathways and interactions, mirroring physiological conditions. This understanding is crucial for progressing research in areas such as cancer biology and stem cell differentiation. However, the multi-layered nature of microfluidic designs can sometimes lead to difficulties in data analysis and interpretation, presenting hurdles for researchers not accustomed to working with such sophisticated setups.
Self-Assembly Techniques
Mechanisms of Self-Assembly
Self-assembly techniques are foundational to scaffold-free cell cultures as they harness the natural ability of cells to organize themselves into structured tissues. The mechanisms of self-assembly can vary widely but generally rely on biochemical signals that guide cells' interactions. This method is particularly notable in 3D culturing, as it doesnโt rely on external scaffolding, allowing cells to create their architecture. This natural construction is often more representative of in vivo conditions, although it can be unpredictable, which challenges researchers in terms of consistency.
Examples in Current Research
Current research showcases various examples of self-assembly techniques applied in scaffold-free cultures. From the formation of spheroids to the generation of organoids, these methods are proving essential in studying diseases and testing drugs. A significant advantage is the ability to mimic native tissue surroundings more efficiently. However, the unpredictability of how cells will organize can complicate experiments, making reproducibility a critical concern. Ultimately, while self-assembly offers many avenues for research and application, keeping a close eye on cell behavior and outcomes remains vital to ensuring reliability.
"The advancements in scaffold-free technologies are not just transforming lab research; they're laying the groundwork for future medical therapies that leverage the natural behaviors of cells."
Whether through sophisticated 3D bioprinting, highly controlled microfluidic systems, or the inherent power of self-assembly, each technological advancement is vital to unlocking the full potential of scaffold-free 3D cell cultures. Understanding these technologies paves the way for innovative solutions in drug discovery, tissue engineering, and regenerative medicine.
Benefits of Scaffold-Free Cultures
Scaffold-free cell culture approaches present a refreshing alternative to traditional methods, providing unique advantages that align closely with the nuanced demands of modern biological research. As researchers delve into these methodologies, the benefits that emerge are both significant and multifaceted, touching upon aspects such as cellular interactions, environmental accuracy, and economic feasibility.
Enhanced Cell-Cell Interactions
One of the standout benefits of scaffold-free cultures is their capacity to promote enhanced cell-cell interactions. In traditional two-dimensional cultures, cells often behave unnaturally due to limitations in their spatial arrangements. When grown in scaffold-free conditions, cells can cluster and organize themselves in three-dimensional structures akin to their in vivo counterparts. This setup fosters a dynamic environment where cells communicate more effectively.
Such interactions are crucial for signaling pathways that continue to evolve, reflecting real biological conditions. The direct communication between cells enables better responses to stimuli and enhances the understanding of various pathologies. In essence, the natural clustering of cells created in scaffold-free environments leads to a more complex and relevant cell behavior.
Improved Modeling of the Microenvironment
Another pivotal aspect of scaffold-free cultures is the improved modeling of the microenvironment. Traditional cultures typically lack the intricate architecture and mechanical properties of actual tissues. In scaffold-free settings, cells grow in a way that more closely resembles their natural surroundingsโconsider aspects like nutrient gradients and waste management.
By recreating these essential components, researchers can achieve more accurate experimental outcomes. This is particularly valuable in areas such as drug development, where understanding how cells react in a microenvironment similar to the body can lead to better drug efficacy assessments. The adaptability of cells in these scaffold-free structures reflects their natural environments, allowing scientists to study complex biological processes that are otherwise difficult to observe.
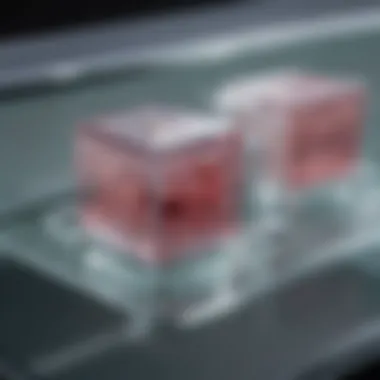
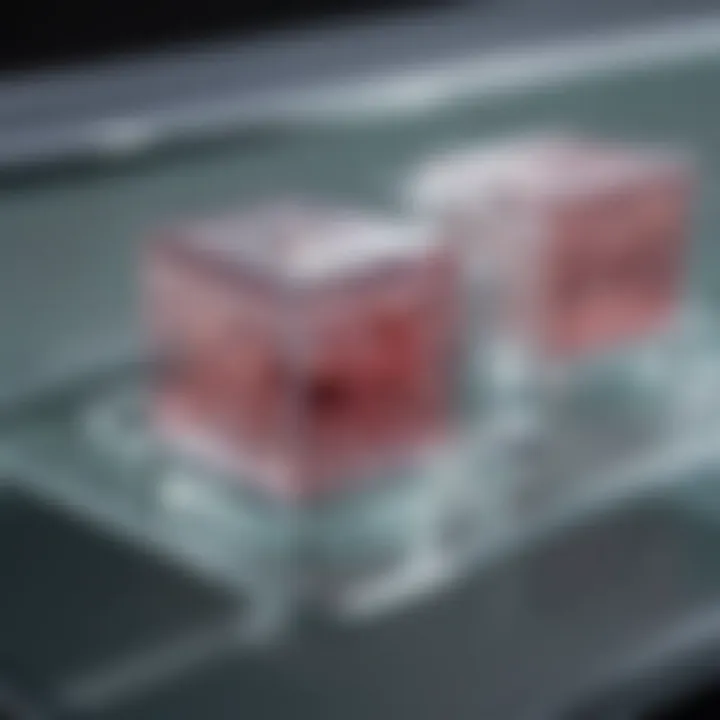
Cost-Effectiveness
Cost considerations often play a significant role in research, and scaffold-free cultures present a more economical alternative. Eliminating the need for scaffolding materials reduces expenses related to reagent costs and the complexity of setup. With growing budgets in research being a consistent challenge, leveraging scaffold-free methodologies can yield equivalent or superior results compared to traditional methodsโwithout breaking the bank.
This cost-effectiveness extends to the scalability of experiments as well. Itโs easier to scale up scaffold-free cultures for high-throughput screenings or larger sample sizes, enabling more extensive data collection while keeping financial constraints in check. This financial feasibility makes scaffold-free approaches an attractive option for laboratories navigating tight research budgets.
In summary, the benefits of scaffold-free cultures are undeniable, leading to more natural cell behaviors, realistic microenvironments, and a lower financial barrier for researchers. The ongoing exploration of these advantages underscored the relevance and necessity of integrating scaffold-free techniques into a myriad of research fields.
Applications of Scaffold-Free 3D Cell Cultures
The use of scaffold-free 3D cell cultures has revolutionized various sectors of biological research. This application allows for the recreation of more accurate in vivo-like environments, providing invaluable contributions in several fields such as drug discovery, tissue engineering, and cancer research. Key benefits include enhanced cellular interactions, better modeling of physiological conditions, and the reduction of costs often associated with traditional culture methods.
Drug Discovery and Testing
Case Studies and Success Rates
Research in drug discovery often emphasizes the need for reliable predictive models. In recent years, scaffold-free methods have emerged as effective platforms, yielding promising results in various case studies. For instance, studies involving stem cells demonstrated that scaffold-free cultures led to higher success rates in drug response predictions, which is a significant factor for pharmaceutical companies looking to shorten development times.
A striking characteristic of these case studies is the focus on using realistic cellular systems instead of simplistic models. This realism has proven beneficial as it mirrors the human body more closely. However, the unique feature of these studies lies in their adaptability. For instance, adjusting cell density and configurations can impact test outcomes dramatically. Nevertheless, the downside is that these cultures may still lack some complexities found in living organisms, which raises questions about their predictive validity in all scenarios.
Challenges in Drug Testing
While scaffold-free cell cultures are beneficial, they aren't devoid of challenges. One substantial aspect is the variability in responses among different cell lines. These discrepancies can make it hard to establish standardized protocols for drug testing. Moreover, researchers have found that in some cases, drug absorption and interactionโboth crucial in testingโmay not accurately reflect real-world scenarios when testing in a lab environment.
The uniqueness of this challenge lies in the balance between a convenient lab setting and the intricate nature of human biology. The unpredictability of drug behavior in these models can lead to disadvantages in developing universally applicable testing methods. Yet, researchers often profoundly appreciate the relevance of scaffold-free techniques, as they provide a more nuanced understanding of drug interactions than 2D cultures could ever offer.
Tissue Engineering
Organoid Development
Organoids represent a cutting-edge innovation in tissue engineering, formed by scaffold-free methods that allow cells to self-organize into miniaturized tissues. This process enhances the predictability of how cells interact and develop, which is critical in mimicking organ functionality. Organ cultures derived through these techniques have shown remarkable similarities to actual organs, demonstrating their significance for in vitro studies.
The notable aspect of organoid development is its ability to replicate complex biological processes, an invaluable resource for researchers. It opens doors to personalized medicine approaches without the ethical concerns often associated with traditional models. However, the downside is the time-consuming nature of both developing and maintaining these organoids, which can limit their practicality in some research settings.
Potential for Regenerative Medicine
Scaffold-free approaches are increasingly being considered pivotal in the field of regenerative medicine. They allow for the creation of tissues that can potentially restore function in damaged organs, thus highlighting their significance in therapeutic contexts. The unique aspect of this application is the way it provides a bridge between research and clinical applications, offering a window into future treatments.
However, one challenge remains: ensuring that the generated tissues maintain the same complex functionality as actual organs over time. Despite this drawback, the potential remains high, showing promise in various applications, from skin grafts to organ replacements.
Cancer Research
Modeling Tumor Microenvironments
Understanding how tumors interact with their environment is a growing necessity in cancer research. Scaffold-free 3D cultures have made it feasible to create tumor microenvironments that are more reflective of actual cancer behavior. These models allow scientists to study tumor characteristics and therapeutic responses in ways that two-dimensional cultures simply cannot.
A crucial characteristic of these models is their ability to not only support cell growth but also to mimic the interactions between cancer cells and surrounding stromal cells. This relevance is vital for generating accurate data that can guide treatment decisions. However, the complexity of constructing and validating these environments can pose significant challenges for researchers, making it clear that while this approach is promising, it also requires continual refinement to achieve optimal results.
Implications for Personalized Medicine
The incorporation of scaffold-free 3D cell cultures in cancer research holds vast implications for the future of personalized medicine. These cultures allow researchers to test different therapeutic agents against patient-specific tumor cells, leading to more tailored therapeutic strategies. The most vital element of this application is the shift toward individualized treatment plans based on accurate real-time results that reflect each patient's unique tumor characteristics.
Yet, a unique challenge here exists in ensuring the reproducibility of results across various labs and testing conditions. While scaffold-free cultures significantly improve understanding patient-specific therapies, variability can sometimes dampen the effectiveness of findings. As researchers continue to refine these methodologies, their potential for advancing personalized medicine will undoubtedly increase.
Challenges and Limitations
In the realm of scaffold-free 3D cell culture, practitioners face a myriad of challenges and limitations that can impact the success and practicality of this innovative approach. Understanding these issues is crucial, not only for researchers immersing themselves in this field but also for regulatory bodies and funding organizations looking to support groundbreaking work. Addressing potential hurdles is key to the broader adoption of scaffold-free techniques.
Technical Difficulties
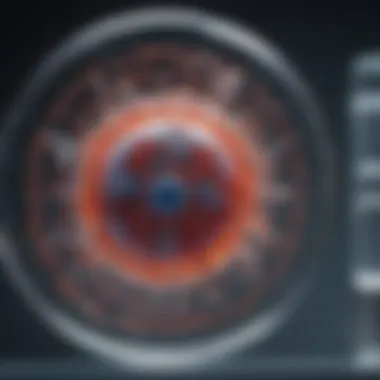
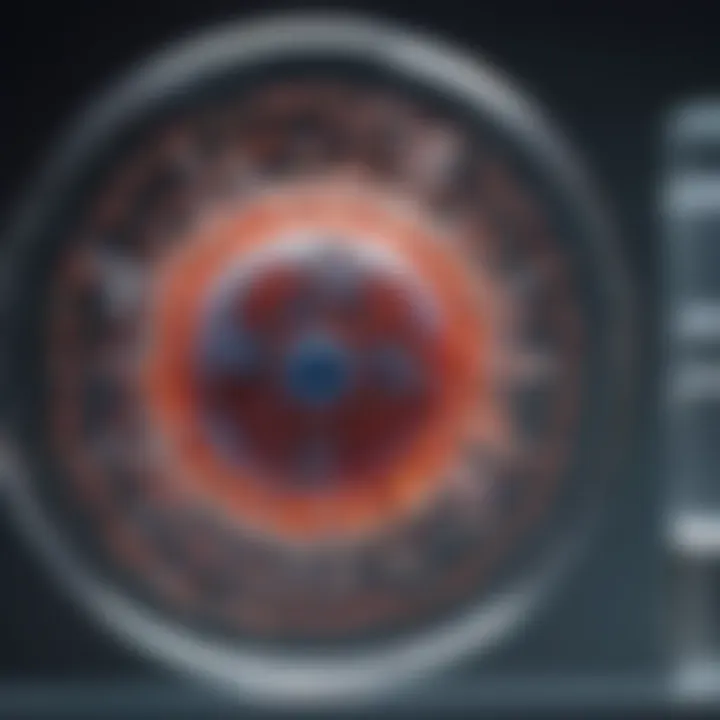
One of the foremost obstacles encountered in scaffold-free cell culture is the need for precise control over cellular behavior. Without a physical scaffold, researchers rely heavily on the intrinsic properties of the cells and their ability to self-organize. This presents significant technical challenges. For instance, achieving a consistent cellular morphology and maintaining viability during culture can be quite a juggling act. Some common technical difficulties include:
- Cell Aggregation: Cells may not aggregate in a reproducible manner, leading to variations in experimental outcomes.
- Nutrient Delivery: Ensuring that all cells receive adequate nutrients and oxygen presents complexities. In larger constructs, diffusion limitations can impede cell health.
- Mechanical Stability: Scaffold-free constructs may lack the stability found in traditional cell culture models, causing cells to break apart or destabilize.
Tackling these technical issues often involves trial and error, which can consume valuable time and resources. Researchers need sound methods for fine-tuning culture conditions, which may require extensive optimization before achieving any reliable cell behavior.
Scalability Issues
While the notion of scaffold-free techniques is promising, transitioning from small-scale laboratory experiments to larger, scalable applications poses its own distinct hurdles. The scalability aspect is particularly relevant for industries aiming to use these cultures for biomanufacturing or therapeutic applications. Some notable points of concern include:
- Uniformity and reproducibility in larger batches can introduce variability that isnโt present in smaller samples.
- Cost considerations come into play when scaling up production as scientists need to balance resources with the scale of experimentation.
- Automation challenges become significant when attempting to integrate high-throughput systems that can handle complex cellular constructs without compromising quality.
As a result, the leap from bench-scale research to real-world applications is often fraught with complications that can dampen enthusiasm for scaffold-free methods.
Regulatory Considerations
Navigating the regulatory landscape is a critical yet complex part of advancing scaffold-free 3D cell culture techniques. Regulatory bodies like the FDA impose strict guidelines to ensure safety and efficacy in therapeutic applications. When it comes to scaffold-free cultures, specific regulatory considerations include:
- Standardization of protocols: There is currently a lack of universally accepted protocols for scaffold-free techniques, leading to potential inconsistencies and reliability issues.
- Long-term effects: Regulatory bodies may require comprehensive studies on the long-term effects of treatments developed from these cultures, which can significantly lengthen the timeline for approval.
- Ethical implications: As with any biological research, ethical considerations must also be addressed, particularly when dealing with stem cells or human-related tissues.
Getting through these regulatory hurdles often requires a comprehensive understanding of both the science involved and the regulatory expectations. Researchers need to be well-prepared to back their methodologies with robust data while engaging with regulatory agencies effectively.
In summary, while scaffold-free 3D cell cultures offer groundbreaking possibilities, a host of technical difficulties, scalability issues, and regulatory considerations must be addressed to unlock their full potential.
By tackling these challenges head-on, researchers can pave the way for the more widespread adoption of scaffold-free techniques, ultimately enhancing their work in drug discovery, tissue engineering, and beyond.
Future Directions in Scaffold-Free Research
Research in scaffold-free 3D cell culture stands on the brink of significant advancements. This area is gaining traction due to its potential to enhance our understanding of cellular behaviors in a more life-like environment. With the evolution of technology and methodologies, the future appears bright, teeming with possibilities that promise to elevate both basic and applied biological research.
Moving forward, we can expect improvements in both the technology and collaboration across diverse disciplines. Each element plays a crucial role in pushing the boundaries of what we currently achieve with scaffold-free techniques. With a landscape shifting toward increased complexity in cell interactions and functionality, unpacking the potential of this research avenue is pivotal.
Technological Innovations on the Horizon
Technological advancements will continue to be pivotal. Cutting-edge innovations are likely to emerge from areas such as AI-driven modeling, allowing researchers to simulate complex biological interactions dynamically. These simulators can offer predictive insights into how cells behave under different conditions, aiding in the design of experiments that more accurately approximate in vivo environments.
Another promising frontier is the enhancement of microfluidic systems. By refining these systems, we can envision more precise manipulation of cell environments, enabling researchers to explore how minute changes affect cell behavior. This capability not only helps in better understanding fundamental biology but also can reflect on the development of personalized medicine approaches.
Moreover, the integration of nanotechnology in scaffold-free cell cultures holds a great promise. The use of nanoscale materials could potentially provide new ways to observe and stimulate cellular responses. Implementing these innovations will allow for more dramatic explorations in fields ranging from drug delivery systems to advanced tissue engineering solutions.
"The integration of new technologies and methods will pave the road for significant advancements in scaffold-free 3D cell culture, fundamentally altering the paradigms of biological research."
Interdisciplinary Collaboration
The intricacies of scaffold-free cell culture warrant a multidisciplinary approach. Collaborations between biologists, engineers, material scientists, and data analysts are essential. Each discipline brings unique skills that can contribute to a more integrated understanding of cell behavior. For instance, biologists can inform engineers about the nuances of cell dynamics, which can help in the design of better experimental setups.
Additionally, interdisciplinary collaboration can lead to novel applications of scaffold-free cultures across various fields. For example, joint efforts in bioinformatics could help in interpreting large datasets generated through experiments. This fusion of insights is likely to culminate in breakthroughs that we haven't yet imagined, perhaps changing the landscape of regenerative medicine altogether.
Finale
The conclusion serves as the final brushstroke on the canvas of this discussion about scaffold-free 3D cell culture. Reiterating what has been examined throughout the article enriches the audience's understanding while emphasizing the profound implications of this innovative approach to cell culture. The scaffold-free methodologies not only mimic in vivo conditions more faithfully but also pave pathways toward breakthroughs in various scientific domains.
Summary of Findings
In dissecting the core components of scaffold-free 3D cell culture, several key takeaways emerge:
- Cellular Interactions: The absence of scaffolds enhances cell-cell interactions, allowing for more representative biological behaviors.
- Modeling Accuracy: This approach improves the modeling of tumor microenvironments, proving crucial for cancer studies and drug discovery.
- Cost-effective Alternatives: Researchers have noted a marked decrease in materials needed, thus streamlining budgets without sacrificing quality.
These factors contribute significantly to the growing interest and application of scaffold-free cultures in research and industry.
Continuing Importance of Scaffold-Free Techniques
As technology progresses, scaffold-free 3D cell culture is expected to play an increasingly vital role. The ability to create environments as close to human physiology as possible cannot be overstated, especially as we navigate through the complexities of diseases and biological processes that impact human health.
- Regenerative Medicine: With its promise to spawn practical applications in tissue engineering and regenerative medicine, researchers are poised to revolutionize patient treatment options.
- Collaboration Across Disciplines: The future will depend on interdisciplinary collaboration. This exchange of ideas and techniques between fields can lead to significant innovations.
In summary, the continued evolution of scaffold-free techniques points towards a future of more effective research outcomes and medical applications, affirming its place at the forefront of biological research.