Functions and Regulation of Beta-Actin Sigma Protein
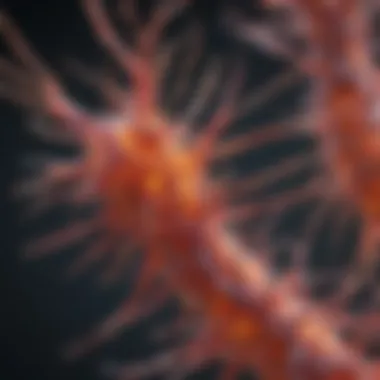
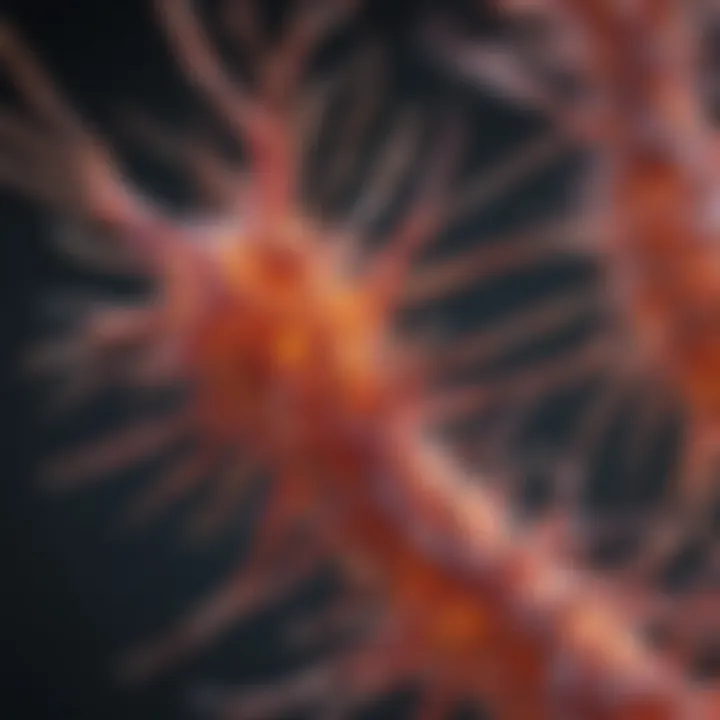
Intro
Beta-actin sigma is a significant protein that plays a crucial role in multiple cellular functions. Its importance goes beyond simple structure; it’s integral to processes like muscle contraction and cell motility. Understanding how beta-actin sigma works is vital, particularly within the context of cellular biology.
Research Overview
Summary of Key Findings
The examination of beta-actin sigma reveals several key insights:
- Cytoskeletal Structure: Beta-actin is a fundamental component of the cytoskeleton, contributing to the cell’s shape and mechanical properties.
- Muscle Function: It is essential in muscle contraction processes, facilitating movement at the cellular level.
- Cellular Motility: The ability of a cell to move, grow, and divide is heavily influenced by beta-actin dynamics.
- Regulatory Mechanisms: Various pathways and signals regulate the expression and activity of beta-actin sigma, impacting overall cellular behavior.
Research Objectives and Hypotheses
The objective of this research is to systematically investigate the functional roles and regulatory pathways of beta-actin sigma. The key hypotheses include:
- Beta-actin sigma is critical for maintaining cellular integrity and functionality.
- Variations in beta-actin sigma expression correlate with specific diseases and health conditions.
- Understanding the regulatory mechanisms can offer insights into potential therapeutic approaches in treating related ailments.
Methodology
Study Design and Approach
The study was designed to utilize both qualitative and quantitative methodologies to explore beta-actin sigma. The approach involved detailed literature review combined with laboratory experiments, focusing on:
- The effects of manipulating beta-actin sigma levels on muscle cells.
- Observing the cellular responses under varying conditions of expression.
Data Collection Techniques
Data was collected through various means:
- Experimental Data: Using techniques like Western blotting to measure beta-actin levels in muscle tissues.
- Observational Data: Tracking changes in cell movement and response in different contexts.
- Literature Analysis: Comprehensive review of existing research articles to consolidate findings.
Understanding beta-actin sigma's functions and its regulatory mechanisms can significantly enhance our insight into cellular biology and its implications in health and disease.
Through a detailed exploration of these areas, this research aims to deepen the understanding and appreciation for beta-actin sigma, propelling forward the discourse in cellular biology while providing a solid foundation for future research.
Prolusion to Beta-Actin Sigma
Beta-actin sigma plays a pivotal role in the intricate web of cellular structures. Understanding this protein is not just a pursuit for biological enthusiasts – it has real-world implications in fields such as medicine, biotechnology, and molecular biology. Its influence on processes like cell motility, muscle contraction, and intracellular transport simply cannot be overstated.
To grasp why beta-actin sigma is so significant, one must consider its place in the larger context of the cytoskeleton. This framework of filaments and tubules supports cellular shape and function. Beta-actin sigma specifically is known for its ability to polymerize and form filamentous structures. These properties are crucial not only for maintaining integrity within cells but also for enabling dynamic movements.
Among the intriguing aspects of beta-actin sigma is its evolutionary relationship with other actins. While some proteins evolve primarily through mutation and environmental adaptation, beta-actin sigma has emerged as a cornerstone for many cellular functions. From cell division to neuron signaling, its functionalities spill into multiple realms of cellular activity.
When we approach the deeper layers of understanding beta-actin sigma, we also unveil its regulatory mechanisms, which govern how it behaves in various cellular environments. This includes not only the influence of genetic factors but also epigenetic modifications and external stimuli.
In essence, delving into beta-actin sigma provides not just a glimpse into the workings of a critical cellular protein, but also bridges gaps in understanding complex biological systems. Grasping both its fundamental and specialized roles prepares researchers and practitioners in the field to address broader biomedical questions.
Definition and Structure
Beta-actin sigma is part of the actin protein family, characterized by its globular structure that can polymerize to form filamentous actin (F-actin). The protein has a molecular weight of approximately 42 kDa, with a highly conserved sequence among species, suggesting its essential roles have endured through evolutionary time.
The protein's three-dimensional conformation facilitates its interactions with other proteins and cellular components. These interactions are often crucial for the organization of the cytoskeleton, a network that provides cells with mechanical support and shape.
Historical Significance
The historical significance of beta-actin sigma traces back to the early days of cellular biology when researchers first elucidated the cytoskeletal architecture. Initially, actins were thought to be merely structural components, but subsequent discoveries revealed their dynamic nature, indicative of deep-seated biological functions.
Over the years, scientists have isolated various isoforms, including the beta variant. This research has paved the way to understanding diseases linked with cytoskeletal dysfunction, such as various types of muscular dystrophies and cancer. It is through these historical lens that we can recognize how foundational knowledge in cytoskeletal biology continues to inform therapeutic strategies in modern medicine.
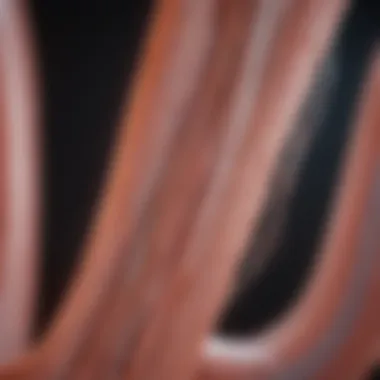
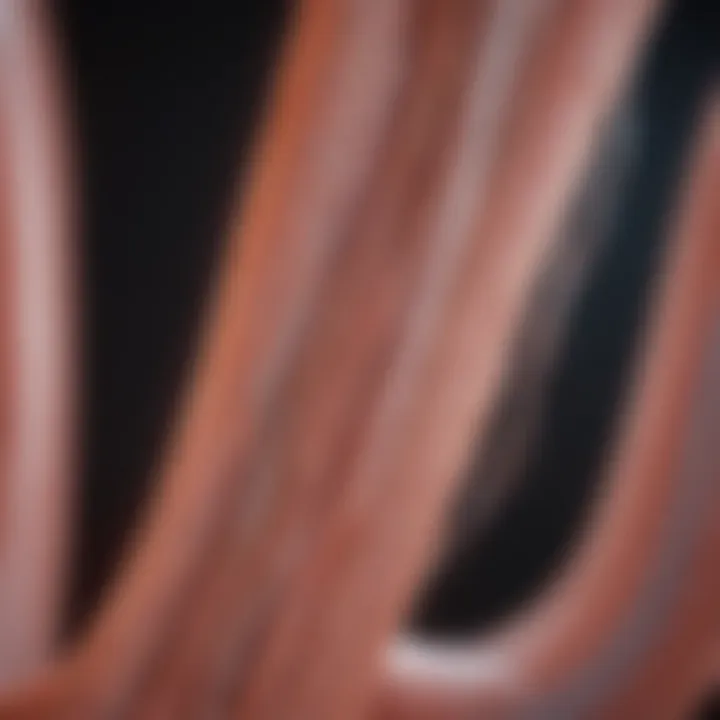
The evolving narrative around beta-actin sigma underscores its invaluable role in cellular function and highlights the interconnectedness of structure, function, and regulation.
The Role of Beta-Actin Sigma in the Cytoskeleton
Beta-actin sigma sits at the heart of the cytoskeletal framework, a true cornerstone that supports not just cellular shape, but also complex functionalities. The cytoskeleton, often regarded as the cell’s skeletal system, comprises intermediate filaments, microtubules, and actin filaments, with beta-actin sigma playing a pivotal role in these dynamics. Understanding its contributions can shed light on critical biological processes, from cellular division to responsiveness during environmental changes.
A primary function of beta-actin sigma in the cytoskeleton is to maintain and manipulate the shape of the cell. It allows cells to adapt to their environments, essentially acting as a scaffold that provides support. Cellular structure isn't simply about holding everything together; it’s also about enabling movement. This facet makes beta-actin sigma indispensable in motile processes, such as wound healing or immune responses, where swift action translates to survival.
Additionally, the implications of beta-actin sigma in cellular mechanics, influence cellular signaling pathways. This position makes it a key player that governs various cellular activities, highlighting its importance not only in quantitative terms but also regarding the qualitative landscape of cellular health and function.
Cytoskeletal Dynamics
Diving deeper into cytoskeletal dynamics, beta-actin sigma is involved in actin polymerization and depolymerization, which are vital for many cellular functions. This dynamic aspect means that beta-actin can rapidly assemble into filaments in response to signals or disassemble when not needed. This plasticity allows cells to not only respond to stimuli but also to organize their interiors for optimal performance.
- Assembly and Disassembly: The ability to swiftly rearrange actin filaments enables cellular movements such as migration. Cells can extend their membrane to form lamellipodia and filopodia, dragging the rest of the cell behind, effectively traveling through tissue spaces.
- Response to Signals: Growth factors or injury signals incite beta-actin sigma to rearrange, allowing for quick responses to changes in the local environment. This responsiveness is fundamental during processes such as tissue repair, where swift migration is paramount.
One notable pathway regulating the dynamics includes several signaling cascades that activate nucleating proteins, prompting the initial formation of actin filaments. This balance between stability and mobility is crucial; it’s this ebb and flow that keeps a cell agile yet grounded, ready to adapt to a changing world.
Interactions with Other Cytoskeletal Components
The interactions between beta-actin sigma and other components of the cytoskeleton are complex yet fascinating. Beta-actin doesn't work in isolation; rather, it collaborates closely with intermediate filaments and microtubules, creating a support network that enhances cellular integrity and functionality.
For instance, connections to microtubules, the rigid part of the cytoskeleton, allow the positioning of organelles throughout the cell. This interplay plays a central role in intracellular transport, ensuring that essential materials reach their destinations efficiently. Furthermore, intermediate filaments, which provide tensile strength, protect against mechanical stress. Beta-actin's fluidity can complement these more stable structures, creating a balanced environment where the cell can stretch without breaking.
The benefits of these interactions are manifold:
- Structural Integrity: The collaborative efforts of these components ensure that the cell maintains its shape while managing various internal and external pressures.
- Cell Motility: The coordination between beta-actin sigma and other filaments facilitates cell movement, crucial for processes like development and immune response.
"Here, we see beta-actin sigma not just as a soloist in the orchestra of cellular structure, but as a foundational player among a symphony of interactions, each supporting the next in a complex performance of life."
Beta-Actin Sigma and Cellular Processes
Beta-actin sigma plays a significant role in numerous cellular processes, primarily due to its involvement in the dynamic architecture of the cytoskeleton. The cytoskeleton is not merely a structural framework; it is integral to various vital functions, such as maintaining cell shape, facilitating intracellular transport, and enabling cell movement. Understanding how beta-actin sigma contributes to these processes reveals insights into fundamental biological mechanisms that govern cell behavior. This comprehension can unveil potential therapeutic targets in diseases where these processes are disrupted, thus emphasizing the importance of studying this particular protein.
Role in Cell Division
In the context of cell division, beta-actin sigma is pivotal. It helps drive the formation of the contractile ring during cytokinesis, enabling the cell to split into two daughter cells. The actin filaments, along with myosin, generate the contractile force needed for this division. If beta-actin sigma is not functioning correctly, cells may experience cytokinetic failure, leading to multinucleation or other division abnormalities. Research indicates that variations in the expression levels of beta-actin sigma during different phases of the cell cycle are crucial, suggesting a tightly regulated process. This regulatory aspect highlights the need for precise molecular timing and interaction among various proteins involved in cell division.
Facilitating Cell Motility
Cell motility is fundamentally influenced by beta-actin sigma. It is essential for processes such as migration and directional movement, which are crucial during development, wound healing, and immune responses. When a cell needs to move, it often extends projections called lamellipodia and filopodia. These structures are rich in actin filaments, primarily made up of beta-actin sigma. The ability of cells to migrate is controlled by the dynamic polymerization and depolymerization of these actin filaments, helping the cell to extend forward and retract its rear. In studying cancer metastasis, for instance, the regulatory mechanisms governing beta-actin sigma's activity become particularly relevant, as they can provide insights into how cancer cells invade other tissues.
Influence on Intracellular Transport
Another critical function of beta-actin sigma is its impact on intracellular transport. It contributes to the movement of organelles, vesicles, and other essential materials within the cell. For example, myosin motors rely on actin filaments for transporting cargo along tracks within the cell. Beta-actin sigma acts as a rail for these molecular machines. Disruptions in this transport system can lead to diseases where the distribution of cellular components becomes uncoordinated. In neurons, beta-actin sigma is crucial for synaptic transport, showcasing its importance in cell communication and signaling. Its role in these processes emphasizes how protein intricacies underpin the complex organization and function of cells.
The unique functionalities of beta-actin sigma underscore its significance in maintaining the lively dynamics of cell life, reinforcing the interconnected nature of cellular processes.
Regulatory Mechanisms of Beta-Actin Sigma
The regulatory mechanisms surrounding beta-actin sigma hold significant importance in understanding its multifunctional roles within cellular environments. These mechanisms not only govern the expression and activity of beta-actin sigma but also influence its interactions with various cellular components. Given the protein's involvement in critical processes like cell division, motility, and intracellular transport, comprehending these regulations can provide valuable insights into cellular functionality and abnormalities.
Transcriptional Controls
Transcriptional control is a primary regulatory mechanism that dictates the level of beta-actin sigma expression within a cell. Gene expression is the first regulatory roadblock that proteins encounter; if transcription doesn't kick off, then that protein doesn't ever see the light of day, right? So how does this happen for beta-actin sigma?
Several elements play a vital role in this transcriptional landscape:
- Promoter regions: The promoter of the beta-actin sigma gene contains specific sequences where transcription factors bind and initiate transcription.
- Transcription factors: Proteins such as serum response factor (SRF) and myocardin play essential roles by binding to these promoter regions and facilitating the transcription process. The presence or absence of such factors can adjust the levels of beta-actin sigma in response to different cellular cues.
- Epigenetic modifications: Changes in the chromatin structure, often mediated through methylation or histone modifications, can have profound effects. When chromatin is tightly packed, it can hinder transcription. On the flip side, more relaxed structures might allow for easier access for transcription machinery.
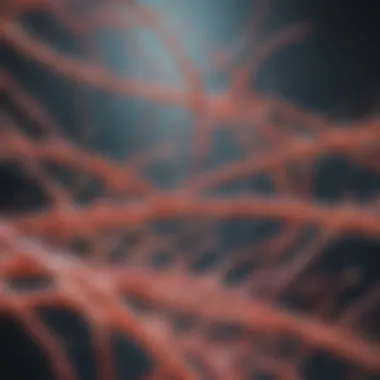
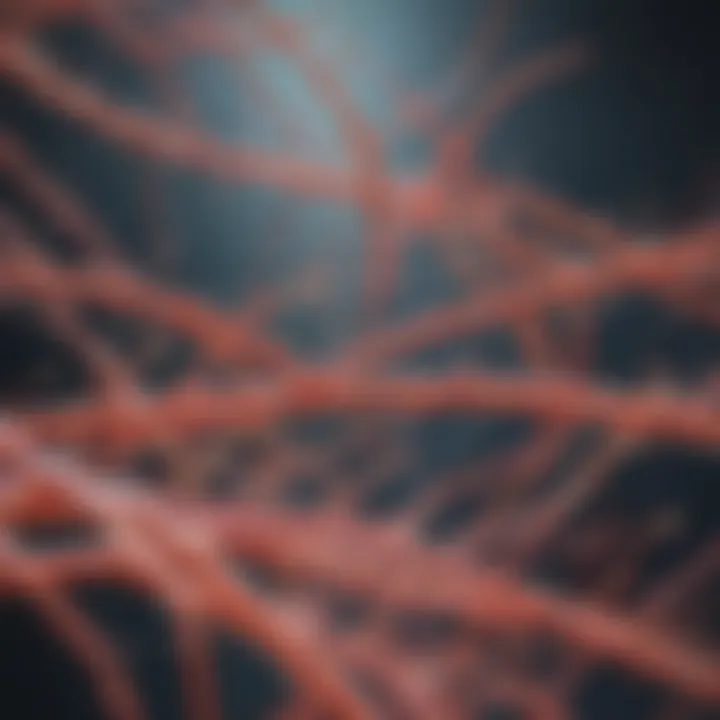
In highly active cells, such as muscle tissue, beta-actin sigma genes are kept on a tight leash. This leads to higher transcription levels during situations where quick response is needed, such as growth or repair. Understanding these transcriptional controls can illuminate how disruptions might contribute to diseases where beta-actin sigma is implicated.
Post-Translational Modifications
Once the protein is synthesized, it still isn't smooth sailing for beta-actin sigma. Post-translational modifications come into play, fine-tuning the protein's functionality, setting it up for success or failure. These modifications are numerous and diverse, and they add layers of complexity to the regulation of beta-actin sigma.
- Phosphorylation: This is one of the most common post-translational modifications. Specific kinases add phosphate groups, which can change protein activity or interaction patterns. For instance, the phosphorylation of beta-actin sigma has been linked to better motility in certain contexts.
- Acetylation: This modification affects the protein’s stability and its ability to bind to other partners, influencing the overall dynamics of the cytoskeletal network.
- Ubiquitination: This process tags the protein for degradation, thus regulating its levels within the cell. If a cell senses that beta-actin sigma has become less functional or surplus, ubiquitination signals "out with the old!"
Post-translational modifications can serve as a finely tuned control system that dynamically adjusts beta-actin levels based on cellular needs and environmental signals.
In summary, the regulatory mechanisms of beta-actin sigma are intricate and crucial for cellular homeostasis. They determine not just how much of this protein is available in a cell, but also its precise functional role through transcriptional controls and post-translational modifications. Understanding these aspects provides deeper insights into both normal cellular operations and the pathological states associated with beta-actin sigma abnormalities.
Variation and Isoforms of Beta-Actin Sigma
Understanding the variation and isoforms of beta-actin sigma is critical to grasping the complete picture of its biological functions. These variants create a landscape of functional diversity that impacts several cellular processes. Each isoform showcases distinct biochemical properties, which can influence how cells respond to external stimuli and maintain structural integrity. Given the protein's role in critical cellular activities, appreciating these variations can unveil insights into abnormalities in cell behavior, as seen in various diseases.
Differences Between Isoforms
Isoforms of beta-actin sigma arise from differences in their amino acid sequences, which are the direct result of gene expression regulation and alternative splicing. This means that just like you can customize a sandwich with various toppings, cells can express different isoforms of beta-actin sigma, effectively customizing their cytoskeletal structure to meet specific functional needs.
While these isoforms typically share much of their sequence, minute disparities in single amino acids can lead to significant differences in properties such as binding affinity with other proteins, structural stability, and even interaction with cellular membranes.
- Higher Flexibility
- Binding Sites
- Performance Under Stress
- Some isoforms present enhanced flexibility, allowing them to adapt better during specific cellular conditions.
- Variations in isoforms can affect binding sites, modifying how they interact with other proteins or signaling molecules.
- Specific isoforms may show resilience against mechanical stress, essential during muscle contraction or cell movement.
The emergence of novel isoforms can also be linked to cellular demands in distinctly regulated pathways. For instance, in muscle cells, variations may adapt to provide the necessary mechanical strength, while in neurons, different isoforms assist in maintaining intricate dendritic structures.
Functional Implications of Variants
The functional implications of beta-actin sigma variants are profoundly significant. Each isoform might play a specialized role in various cellular contexts, influencing overall cell behavior.
For example, one variant could enhance adhesion properties in epithelial cells, which is crucial for tissue integrity, whereas another may promote mobility during developmental stages. The presence of different isoforms in specific tissues can serve as a mechanism for cells to fine-tune their functions in response to environmental cues.
- Cellular Motility
- Signal Transduction
- Disease Associations
- Variations can affect how efficiently cells migrate, impacting wound healing and immune responses.
- Certain isoforms may assist in transferring signals from the extracellular environment to the cell’s interior, enabling timely cellular responses.
- Altered expression levels of one or multiple isoforms are often found in pathologies like cancer, where they might promote invasive characteristics.
The nuanced roles of these isoforms underline the importance of studying beta-actin sigma in varied biological contexts. The variances serve as a conversation starter for researchers keen on unraveling the intricacies of cellular mechanics.
"Understanding the specific roles of different isoforms can elucidate the pathways involved in health and disease, paving the way for targeted therapies."
In summary, exploring the diverse isoforms of beta-actin sigma is not just an academic exercise; it's a gateway to understanding how cells adapt to their environments and respond to various physiological demands. Whether one is engaged in fundamental research or applied medical studies, knowledge of these differences holds implications for advancements in health care and biological science.
Beta-Actin Sigma in Development
Beta-actin sigma plays a pivotal role in the myriad processes that underpin development in organisms, from the earliest stages of embryonic formation to the intricate tissue structures that define adult anatomy. Understanding its function is essential, as it lays the groundwork for comprehending how cellular structures evolve and how misregulation can lead to developmental anomalies.
Role in Embryonic Development
In the sphere of embryonic development, beta-actin sigma is indispensable. It is a fundamental component in creating the cytoskeletal framework, which influences cell shape, motility, and division. During early embryogenesis, precise cell movements are crucial, and beta-actin sigma is like the glue holding everything together. It helps to convert biochemical signals into cellular actions, a process that is vital in establishing the embryonic layer called the ectoderm, which later differentiates into skin and nervous tissue.
One of the significant aspects of beta-actin sigma in embryonic development is its involvement in the formation of cellular structures known as lamellipodia. These protrusions are crucial for cell migration, enabling cells to navigate and position themselves properly during the critical early stages of life. If beta-actin sigma is inhibited or its expression altered, it can lead to improper positioning of cells, resulting in developmental defects or even embryonic lethalities.
Moreover, > "The manifestation of structural and functional integrity in early development reflects the orchestrated effort of multiple cytoskeletal players, with beta-actin sigma at the helm." This assertion signifies how vital beta-actin sigma is in not only facilitating cell movement but also ensuring that cells communicate and interact correctly within the developing embryo.
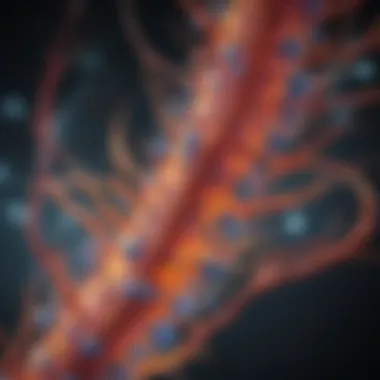
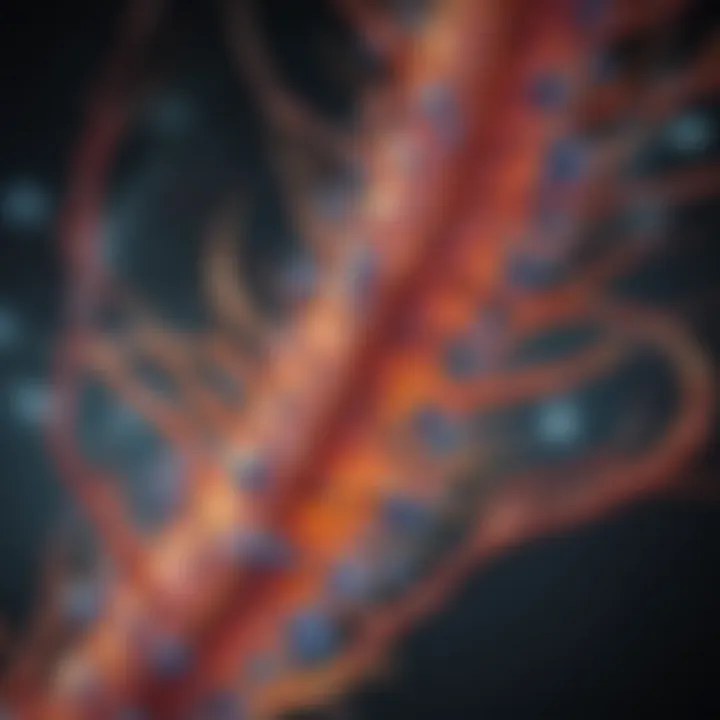
Effects on Tissue Morphogenesis
As development progresses, tissue morphogenesis becomes essential—a process whereby cells organize into tissues and organs. Beta-actin sigma's role in this stage is paramount. It facilitates not only cell adhesion but also the intricate changes in cell shape that are critical for developing arm parts, legs, and even organs.
Here, the regulation of beta-actin sigma is tightly woven with other cellular pathways, such as those governing cell signaling and gene expression. Consider the intricate dance where beta-actin sigma interacts with cadherins and integrins—proteins that mediate cell-cell and cell-matrix adhesion. The cooperation among these proteins allows for the establishment of tight junctions between cells, a necessary step for cohesive tissue formation.
Additionally, changes in the expression levels of beta-actin sigma can have profound effects on the overall morphology of the developing tissue. Overexpression might lead to hyperplastic growth, while underexpression can result in insufficient tissue formation. This delicate balance is tightly regulated and reflects the essential role of beta-actin sigma in ensuring that tissues form correctly, demonstrating the high level of precision required in development.
Pathophysiological Contexts Involving Beta-Actin Sigma
In contemporary cellular biology, the study of beta-actin sigma has unveiled its crucial implications in diverse pathophysiological contexts. Understanding this relationship is a significant aspect of ongoing research, especially in areas like cancer and infectious diseases. This section aims to dissect the multifaceted roles that beta-actin sigma plays, particularly when circumstances in cellular environments deviate from the norm.
Implications in Cancer Biology
Beta-actin sigma emerges as a critical player in cancer biology, where its functions blend intricate cellular dynamics with tumor progression.
- Cell Motility: Cancer cells often exhibit enhanced motility, enabling them to metastasize. Beta-actin sigma facilitates this movement through the remodeling of the cytoskeleton, allowing cancer cells to invade adjacent tissues. This aggressive behavior can lead to the establishment of secondary tumors, making understanding its role imperative.
- Regulation of Cell Proliferation: Research indicates that aberrant expression of beta-actin sigma correlates with altered signaling pathways associated with cell proliferation. When beta-actin sigma is overexpressed, it may contribute to uncontrolled cell division, a hallmark of cancer.
- Interaction with Signaling Pathways: This protein interacts with key oncogenic pathways, such as the PI3K/Akt and MAPK/ERK pathways, which are crucial for cell survival and growth. By influencing these pathways, beta-actin sigma may either facilitate or hinder tumorigenesis.
"Beta-actin sigma does not merely serve a structural purpose; its involvement in signaling pathways can dramatically alter the fate of cancer cells."
Role in Viral Infections
The role of beta-actin sigma is not confined to cancer alone; it has also shown a remarkable involvement in the response to viral infections. Its dynamics can shift in the presence of pathogens, indicating a tightly regulated interplay.
- Viral Entry and Replication: Some viruses exploit the cytoskeletal components to enhance their entry into host cells. Beta-actin sigma can participate in this process, influencing the membrane dynamics and enabling viral particles to breach cellular barriers more efficiently.
- Cellular Defense Responses: Conversely, beta-actin sigma is also implicated in the host's immune response. It contributes to the formation of structures necessary for recognizing and eliminating infected cells. In this light, its regulatory mechanisms become vital in understanding how cells respond to viral threats.
- Cytokine Release: The rearrangement of beta-actin sigma is essential for the release of pro-inflammatory cytokines, which play a role in the systemic response to infections. Understanding its pathophysiological role can illuminate new therapeutic avenues for managing viral diseases.
Consideration of beta-actin sigma within these pathophysiological frameworks underscores its significance not just as a structural element but as a pivotal feature in cellular signaling and responses to disease. Addressing its role can open doors for innovative therapeutic strategies, especially in cancer and infectious disease management.
Experimental Approaches to Study Beta-Actin Sigma
To effectively explore beta-actin sigma, various experimental approaches are pivotal. They help elucidate the molecular dynamics and functional roles of this critical protein within cells. Understanding these methods provides insight into how researchers can manipulate and observe beta-actin sigma under different conditions.
One significant aspect of studying beta-actin sigma involves appreciating not just what it does, but how researchers gather evidence of its functions. Techniques in molecular biology and imaging are especially useful in dissecting the nuances of its roles and regulatory mechanisms.
Molecular Techniques
Molecular techniques are at the forefront of studying beta-actin sigma. These methods help in understanding the intricate processes involving gene expression, protein interactions, and cellular pathways.
- Gene Silencing: This can be achieved through siRNA or shRNA techniques aimed at silencing or knocking down the expression of beta-actin sigma. By examining the resultant cellular behavior, researchers can infer the importance of beta-actin sigma in various physiological processes.
- Western Blotting: This technique allows for the detection and quantification of beta-actin sigma protein levels after suitable extraction and separation. Knowing fluctuations in protein amounts under various conditions helps connect its presence to cellular functions.
- PCR Techniques: Polymerase chain reaction techniques, including quantitative PCR, enable researchers to measure changes in mRNA levels of beta-actin sigma. This can provide insights into how expression levels vary in response to different stimuli or in disease contexts.
- Co-immunoprecipitation: This is utilized to study interactions between beta-actin sigma and other proteins. Unraveling these interactions can highlight how beta-actin sigma might influence cellular architecture or activities.
Using these molecular techniques sheds light on beta-actin sigma’s multifaceted roles, supporting its importance across different cellular processes.
Imaging Strategies
Imaging strategies complement molecular techniques beautifully. They allow for the visual examination of beta-actin sigma within cells, providing a dynamic perspective that static molecular data cannot fully convey.
- Fluorescence Microscopy: Employing fluorescent tagging of beta-actin sigma permits observation of its localization in live cells. Researchers can track how it moves or changes in abundance in real-time under various conditions. Understanding spatiotemporal dynamics can reveal a lot about its functional roles.
- Live Cell Imaging: This approach allows prolonged examination of beta-actin sigma activities as cells undergo different stages of morphogenesis or response to external stressors. It can demonstrate how cells adapt and reorganize their cytoskeletal components in a live setting.
- Electron Microscopy: For ultra-structural insights, electron microscopy can provide resolution down to the molecular level. This can reveal how beta-actin sigma contributes to the overall architecture of the cytoskeleton and its interactions with other proteins and cellular structures.
Key takeaway: Integrating molecular techniques and imaging strategies allows researchers to gain a complete picture of beta-actin sigma's functions and regulatory mechanisms.
By employing these diverse experimental approaches, a more comprehensive understanding of beta-actin sigma can be achieved. This is crucial not only for academic pursuits but also for potential clinical applications, as insights gained could be vital in addressing pathophysiological conditions where beta-actin sigma is implicated.
The Ends and Future Directions
In summarizing the exploration of beta-actin sigma, we acknowledge its indispensable role in numerous cellular functions and how its precise regulation can significantly influence cellular behavior. As we have traversed the molecular landscape of beta-actin sigma, it is essential to recognize that while we stand on solid ground with established knowledge, uncharted territories remain. The interplay between beta-actin sigma and cellular processes is a finely tuned mechanism, prompting continued investigation into its roles across various biological systems.
Key Takeaways
- Integral Component: Beta-actin sigma serves as a key player in maintaining the structural integrity of the cytoskeleton, thus facilitating vital cellular functions like movement, division, and transport.
- Regulatory Nuances: The intricate regulatory mechanisms, involving transcriptional controls and post-translational modifications, underline its versatile roles, adapting to different cellular demands.
- Health Implications: Its significance transcends basic biology, impacting pathophysiological conditions such as cancer and viral infections, making it a potential target for therapeutic interventions.
- Isoform Diversity: Understanding the various isoforms and their functional implications can lead to enhanced insights into disease mechanisms and therapeutic strategies.
Research Gaps and Opportunities
Despite the thorough exploration conducted thus far, several research gaps beckon deeper inquiry:
- Understudied Isoforms: Many isoforms of beta-actin sigma remain poorly characterized. Future studies could elucidate their specific functions and regulatory pathways.
- Interactions Beyond the Cytoskeleton: There is a pressing need to examine how beta-actin sigma might interact with non-cytoskeletal proteins and systems, potentially impacting signaling pathways that govern cellular health.
- Pathological Mechanisms: An in-depth understanding of how dysregulation of beta-actin sigma contributes to diseases could open avenues for novel biomarker identification and therapeutic intervention.
- Technological Advancement: As imaging techniques and molecular biology improve, they hold promise for the visualization and manipulation of beta-actin sigma within live cells, providing real-time insights into its dynamic roles.
The journey through the facets of beta-actin sigma thus not only underlines its critical contributions to cellular dynamics but also highlights the horizon of intriguing research possibilities. With advancing technologies and interdisciplinary approaches, the future promises enhanced understandings that could significantly impact health sciences.