Innovations in Biomaterials and Tissue Engineering
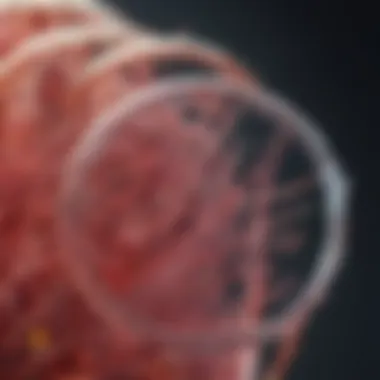
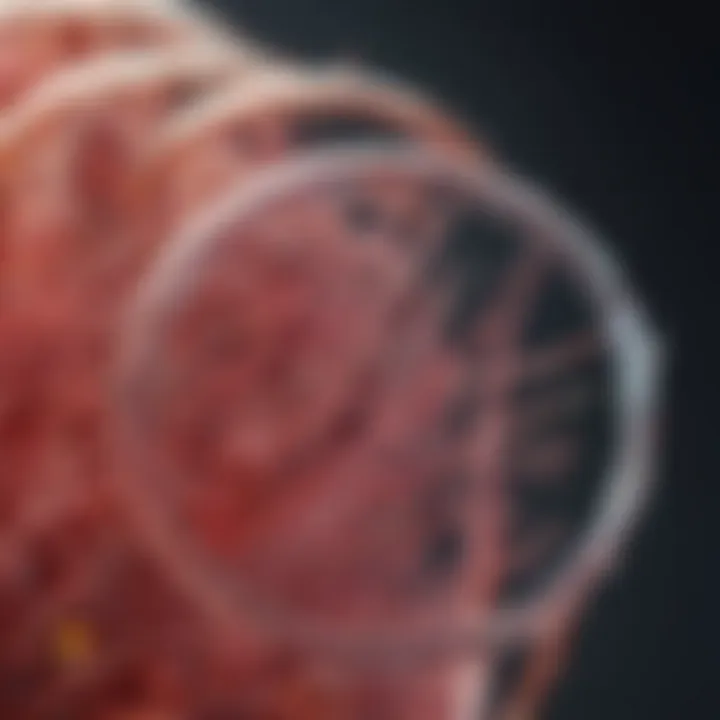
Intro
As the realms of medicine and material science converge, the emergence of tissuetech is reshaping our understanding of regenerative medicine. This innovative field focuses on the wow-factor of biomaterials and tissue engineering, opening doors to possibilities that were once relegated to science fiction. In recent years, researchers and scientists have been delving deeper into how these materials can mimic or support natural tissues, influencing everything from surgical techniques to the development of artificial organs.
Tissuetech focuses not just on isolated advancements but the entire ecosystem of materials, methods, and applications. By integrating data, technology, and biological insights, this field crafts solutions intended to overcome challenges in tissue restoration and regeneration. Through rigorous studies and creative thinking, tissue engineering is evolving into a discipline that’s as much about science as it is about art.
In exploring this topic, we aim to provide an enriched narrative that goes beyond the jargon to elucidate what tissuetech truly means for scientists, healthcare professionals, and patients alike. Whether it’s the potential to regenerate damaged organs or the creation of bespoke materials for specific surgical applications, the implications are vast. Let's journey together through the myriad pathways of this exciting field.
Prolusion to Tissuetech
Tissuetech stands at the intriguing intersection of biomaterials and tissue engineering, where hope meets innovation. As the medical field pushes the boundaries of what is possible, the importance of this topic cannot be overstated. With increasing occurrences of chronic diseases and injuries, the demand for more advanced treatment options is growing, creating a fertile ground for research and development in this field. Tissuetech opens doors to creating biological substitutes that can repair, replace, or restore damaged tissues, making a profound impact on regenerative medicine.
One key benefit of Tissuetech is its capacity to enable more personalized healthcare solutions. Unlike traditional treatments that often employ a one-size-fits-all approach, innovations in tissue engineering facilitate the creation of biomaterials tailored to individual patient needs. For instance, customizing scaffolds to enhance cell growth or adjusting drug delivery systems can significantly improve healing outcomes. This is especially beneficial for conditions that require intricate repairs, such as complex bone fractures or extensive soft tissue injuries.
However, the path to realizing the full potential of Tissuetech is not without challenges. The integration of complex biological systems with engineered materials requires a deep understanding of both fields. Researchers must consider aspects like biocompatibility, which determines how well a material interacts with the living body. Additionally, the regulatory landscape poses hurdles; navigating through the myriad of guidelines can slow down the introduction of new technologies to the market.
Overall, exploring Tissuetech is not just about the innovations themselves but also understanding their implications for both healthcare providers and patients. As we delve deeper into this article, the revelations about biomaterials and tissue engineering will illustrate how these advancements can transform treatment methodologies.
"The journey of tissue engineering is akin to composing a symphony, where every element, from cells to scaffolds, plays an integral role in creating a harmonious solution for healing."
In summary, the introduction of Tissuetech highlights the commitment to pushing forward with creativity in addressing medical challenges while simultaneously navigating the complexities and responsibilities that come along with it.
Understanding Biomaterials
Biomaterials form a cornerstone of the tissuetech landscape, acting as the vital link between biological systems and engineered constructs. They play an instrumental role in how we approach complex issues in medicine, such as regenerative therapies and custom implants. Understanding these materials can shed light on their potential, limitations, and the technical considerations that influence their application.
Definition and Importance
Biomaterials are broadly defined as materials that, either alone or in combination, are used to treat, enhance, or replace any tissue, organ, or function of the body. The importance of these materials cannot be overstated, given their crucial role in medical innovations and therapies.
Biomaterials must be biocompatible, meaning they should not provoke an adverse reaction when introduced into the body. In other words, they need to seamlessly integrate into the host system, allowing for functionality without causing harm. This characteristic is fundamental, as the success of implants and tissue constructs largely hinges on this compatibility.
Types of Biomaterials
Different types of biomaterials serve various purposes, and understanding these distinctions helps inform their application in modern medicine.
Natural Biomaterials
Natural biomaterials come from biological sources like collagen, chitosan, and hyaluronic acid. The key characteristic of these materials is their inherent compatibility with the human body. Because they mimic the body’s own tissues, they are often favored in regenerative medicine.
One unique feature of natural biomaterials is their bioactivity; they can promote cellular growth and tissue healing in ways synthetic counterparts may struggle to achieve. However, they tend to possess lower mechanical strength compared to synthetic options, which can pose challenges in load-bearing applications.
Synthetic Biomaterials
Synthetic biomaterials like polylactic acid (PLA) and polycaprolactone (PCL) are crafted through chemical processes. The most significant advantage of these materials is the ability to engineer specific properties tailored to meet particular clinical needs. For instance, adjusting the polymer's molecular weight affects its degradation rate and mechanical strength.
Nevertheless, a possible downside lies in their biocompatibility issues. Synthetic materials may sometimes lead to a foreign body response, although advancements in surface modification have been enhancing their compatibility.
Composite Biomaterials
Composite biomaterials combine natural and synthetic elements to capitalize on the strengths of both types. These materials often demonstrate improved mechanical properties while maintaining some degree of biocompatibility. A key characteristic of composite biomaterials is their versatility in design, allowing them to be tailored for specific applications like bone scaffolds or cardiovascular devices.
On the downside, the complexity in manufacturing and potential interactions between constituents can lead to variable performance, making consistency a concern in their use.
Applications in Medicine
The application of biomaterials in medicine is expansive and key to many contemporary treatments.
Implants
Implants like hip joints or dental fixtures are perhaps the most recognized use of biomaterials. The critical aspect of implants is their need for integration with surrounding tissue. This must be carefully managed to ensure the durability and longevity of the implant.
A unique feature of modern implants is the use of bioactive coatings, which can enhance osseointegration. However, complications such as loosening or infection still pose risks, necessitating ongoing innovation in material design.
Drug Delivery Systems
Biomaterials also play a significant role in drug delivery systems. These systems allow for the controlled release of therapeutics at targeted sites within the body. The characteristic of sustained release profiles is particularly attractive, as it minimizes the need for repeated dosing.
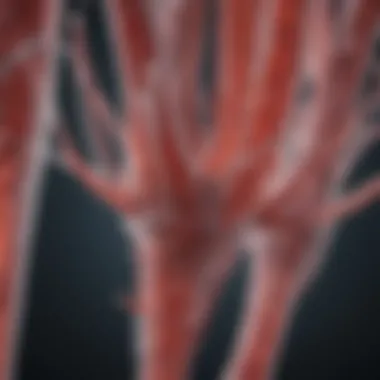
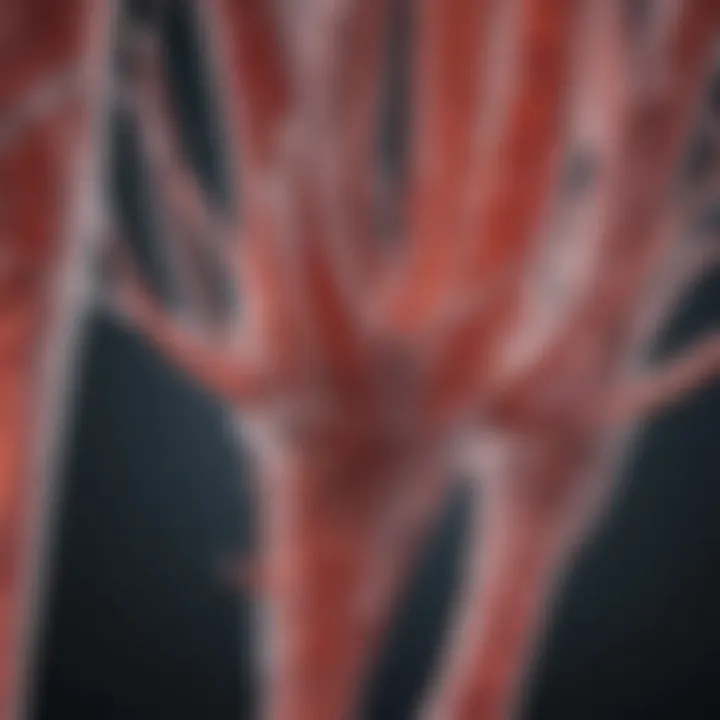
A unique aspect of these systems is their ability to improve drug solubility and stability. Nonetheless, achieving precision in dosing can still be a challenge, and there’s a risk of patient noncompliance if delivery methods are cumbersome.
Scaffolds for Tissue Engineering
Scaffolds are temporary structures that provide a framework for cells to grow and develop into functional tissues. The significance of scaffolds lies in their ability to facilitate tissue regeneration, serving as a platform for cellular infiltration and proliferation.
Unique to scaffolds is their design flexibility, which can be tailored to mimic natural extracellular matrices. However, ensuring adequate vascularization while preventing structural collapse remains a crucial challenge in scaffold design.
In summary, understanding biomaterials is essential, not just for researchers, but for all professionals engaged in executing or evaluating medical applications. It is the bedrock upon which future innovations are constructed.
Technological Foundations of Tissuetech
The realm of tissuetech is firmly rooted in its technological foundations, which provide the essential building blocks for innovations in biomaterials and tissue engineering. Understanding these technological elements is crucial for grasping how tissuetech is reshaping medical science. Advances in technology have paved the way for developing more effective, functional, and biocompatible materials that can mimic the natural properties of human tissues. This contributes not only to improving existing treatments but also to developing new therapeutic strategies.
3D Printing in Tissuetech
Overview of 3D Printing Techniques
3D printing is a key player in the tissuetech landscape, enabling the precise creation of three-dimensional structures that can closely replicate the intricate architecture of biological tissues. One notable aspect of 3D printing techniques is their ability to customize objects based on specific patient needs. The most popular techniques include Fused Deposition Modeling and Stereolithography, which allow researchers to build layers of material with pinpoint accuracy.
The main advantage of 3D printing in tissuetech lies in its versatility. It can produce complex geometries that traditional manufacturing methods cannot achieve. This adaptability makes it a go-to choice for creating implants, scaffolds, and even entire organs for regenerative purposes.
Materials Used in 3D Bioprinting
When talking about materials for 3D bioprinting, focus often shifts to biocompatibility and functionality. Common materials like hydrogels, ceramics, and certain polymers play significant roles in creating supportive environments for cell growth. Hydrogels, for instance, are designed to maintain a wet environment akin to human tissue, which is pivotal for cell survival.
However, this choice of materials does come with challenges. Not all materials can support complex cell structures for an extended period, which limits long-term applications. Still, the ongoing research is promising, pushing boundaries every day.
Case Studies and Applications
Analyzing specific case studies can provide insight into the vast potential of 3D printing in tissuetech. For instance, a recent application involved creating a bioprinted lung model that researchers used to test the effects of medications on lung cells. This practical, real-world usage enhances our understanding not just of the technology but also of patient care.
Unique to these applications is their ability to streamline testing processes and reduce the need for animal models, aligning with ethical standards in research. Yet, while promising, the limited scalability and cost factors can pose challenges to wider adoption in clinical settings.
Bioprinting Advances
Cellular Structures
Cellular structures created through bioprinting represent a significant leap forward in tissue engineering. The ability to print viable cell layers that function similarly to native tissues makes this an area of intense study. Moreover, these printed structures can be tailored to specific types of cells, enabling a more targeted approach to tissue regeneration.
One characteristic of these structures is their ability to improve integration with surrounding biological tissues. This is especially important for applications like skin grafts, where the integration process can dictate the success of treatment. On the downside, the complexity of cellular interactions makes it difficult to forecast outcomes in all cases, necessitating further research and refinement.
Vascularization Techniques
Vascularization is a hot topic when it comes to bioprinting advancements. Effective vascular networks are essential for tissue survival, as they supply nutrients and facilitate waste removal. Techniques devised for embedding vascular channels during the printing process are changing the game. Researchers can now create more robust and functional tissue constructs that stand a higher chance of thriving in a human body.
However, the intricacy of creating functional vascular networks poses limitations, primarily because successful integration with the host's vascular system requires an understanding of complex biological dynamics.
Integration with Native Tissue
Focusing on integration with native tissue, this area examines how bioprinted tissues interact with existing biological structures. This integration is crucial for developing successful implants that the body can accept and incorporate. One noteworthy approach is the use of bioactive materials that promote healing and integration.
The challenge here remains: mimicking the natural signaling pathways that facilitate integration in the body can be incredibly complex. While techniques are improving, achieving seamless integration with existing tissues remains a hurdle.
Stem Cell Technologies
Types of Stem Cells
Stem cells lie at the heart of tissue regeneration, offering the potential to develop new tissues and even organs. Various types, including embryonic and induced pluripotent stem cells, present unique avenues for research and application. Each type has its benefits, such as the high proliferation rates of induced pluripotent stem cells, making them particularly attractive for tissue engineering.
Nonetheless, the variability in stem cell differentiation can create unpredictability in results, necessitating rigorous research and quality control to guide their application in healing.
Role in Tissue Regeneration
Examining the role of stem cells in tissue regeneration opens up exciting avenues in medicina. These cells can stimulate healing and encourage tissue growth, making them essential in regenerative therapies. Understanding how stem cells operate at the molecular level can unlock numerous possibilities for treating chronic injuries and degenerative diseases.
Despite their promising roles, challenges around sourcing and ethical considerations—particularly concerning embryonic stem cells—remain hot-button issues within the scientific community.
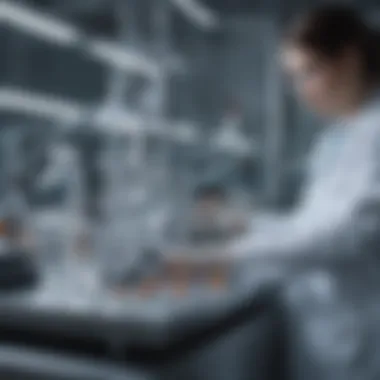
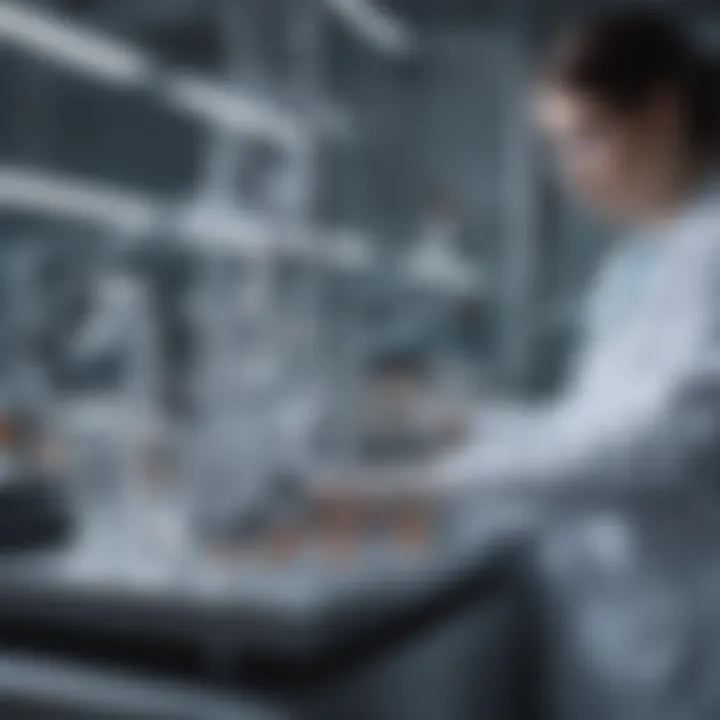
Ethical Considerations
As with any innovative technology, ethical considerations are paramount in the discussion surrounding tissuetech, especially when it involves stem cells. The moral implications of using embryonic stem cells and the potential consequences of genetic modifications elicit heated debate. Making informed choices that balance scientific advancement with ethical responsibility is crucial for fostering public trust.
Moreover, as technologies evolve, so too must the ethical frameworks that govern their use. Engaging diverse voices in this dialogue is essential to ensure equitable advancements in medicine that are broadly beneficial.
Challenges and Considerations
Navigating the world of tissuetech brings with it a set of challenges that cannot be overlooked. These considerations are not merely footnotes in the larger conversation about biomaterials and tissue engineering; they are central to its future and application in medicine. It's important to recognize that while the innovations in this field are exciting, they also come with hurdles that can slow progress or complicate the landscape for researchers, clinicians, and patients alike.
Regulatory Hurdles
FDA Regulations
The FDA regulations are a linchpin in ensuring that products emerging from tissuetech are safe and effective for human use. This regulatory framework is designed to scrutinize every angle—from biocompatibility to functionality—before any new material can make its way into clinical settings. The key characteristic of these regulations is their thoroughness. They place a strong emphasis on rigorous testing protocols that can take years to complete. For this reason, innovations can often feel as if they are stuck in a bureaucratic quagmire. However, this diligence is beneficial as it assures that any biomaterials used in patient treatments will not only function as intended but also minimize harm.
One unique feature of FDA regulations is the premarket approval pathway, which serves as a double-edged sword. On one side, it serves to protect public health by enforcing high standards; on the other, it poses challenges that can delay the entry of groundbreaking solutions into the market.
Global Regulatory Landscape
Looking beyond the borders of the U.S., the global regulatory landscape adds another layer of complexity. Different countries have unique standards and approval processes, which can sometimes be more lenient or more stringent than those imposed by the FDA. The diverse nature of these regulations is a key consideration for companies seeking to market their products internationally. It allows firms to adapt their strategies and tailor their submissions based on regional requirements.
Nonetheless, navigating these varied frameworks can create confusion or lead to missed opportunities. Moreover, harmonizing regulations across regions remains a challenge that puts small companies at a disadvantage compared to well-established corporations with ample resources.
Future of Compliance
The future of compliance in tissuetech likely involves an evolving relationship between innovators and regulatory bodies. One key aspect is the push for more streamlined processes, possibly incorporating digital tools to enhance transparency in testing and approval stages. This shift might make it easier for new ideas to cross the chasm from research to realization, promoting faster time-to-market.
However, this evolution also necessitates caution. Greater efficiency must not come at the cost of patient safety or product quality. As the industry develops, striking the right balance between innovation and oversight will be essential for fostering trust and ensuring long-term success.
Material Limitations
Biocompatibility Issues
Biocompatibility stands as a critical component in the success of any biomaterial in medical applications. These materials must interact favorably with biological systems without eliciting an adverse immune response. The crucial aspect of biocompatibility is that it's not a one-size-fits-all equation; each application may require distinct considerations. For instance, a material that works brilliantly in a dental application may not perform adequately as a vascular graft.
This variability often leads researchers down a winding road as they test and refine materials, ultimately delaying clinical application. A unique feature in this context is the ongoing refinement of testing methodologies, which is crucial for identifying issues early. The downside, however, is the resource-intensive nature of this issue, requiring both time and funding that could be directed toward other innovative pursuits.
Mechanical Properties
Mechanical properties are equally pivotal in ensuring that biomaterials can withstand the rigors of their intended environment. From tensile strength to elasticity, the functional reliability of a material is often directly proportionate to its mechanical characteristics. The key characteristic here is also context-dependent. For instance, orthopedic implants need to support significant loads over time, whereas soft tissue scaffolds may prioritize flexibility.
Though developments in materials science are promising, there’s still a long way to go in optimizing mechanical performance. A unique feature in this domain is the emergence of advanced composites that could marry the strengths of both natural and synthetic materials. Yet, achieving this ideal blend can introduce other complications, such as increased production costs or complicated manufacturing processes that challenge scalability.
Durability Concerns
Durability raises its own set of issues. Biomaterials should maintain their integrity over extended periods, particularly in load-bearing applications. The important aspect of durability is that it often intertwines with aging and degradation processes. It’s crucial to understand how materials age within the human body.
The unique feature of durability concerns is that while initial testing might display promising results, unforeseen issues could arise after long-term implantation. This reality can lead to failures that compromise the effectiveness of tissuetech solutions. As such, long-term studies remain critical to establish confidence in a material's life span, which inherently runs counter to the fast-paced nature of technological advancement in the sector.
Ethical Dilemmas in Tissuetech
Human Trials
When it comes to human trials, the stakes couldn't be higher. It’s undeniably the bridge between theoretical research and real-world application. This key characteristic is significant because it involves navigating ethical considerations regarding consent and the potential risks to participants. Each trial must demonstrate that the potential benefits outweigh the risks involved.
The unique feature of human trials in this field is that they often demand a multi-phased approach, where initial trials focus on safety before progressively examining efficacy. While this phased process aims to protect participants, it also delays the availability of beneficial treatments to wider populations.
Societal Impacts
The societal impacts of advancements in tissuetech cannot be underestimated. The key characteristic here involves examining how new technologies alter healthcare dynamics, including issues of access and affordability. As cutting-edge therapies become available, there’s the looming concern about who gets access to these innovations.
One unique feature of this societal impact is the growing dialogue surrounding equity in medicine. As technological disparities may arise, it invites critical discussions about how to bridge these gaps. Failure to do so can result in a widening chasm between those who can afford innovative care and those left behind, making it a pressing ethical concern.
Long-term Monitoring
Long-term monitoring represents a significant ethical issue that researchers must grapple with after a new treatment is approved. The important aspect of this monitoring is that it’s not merely about assessing the initial efficacy but being vigilant about potential long-term consequences. This oversight can be a double-edged sword; while it ensures patient safety, it also requires ongoing resources and systemic support that can sometimes be lacking.
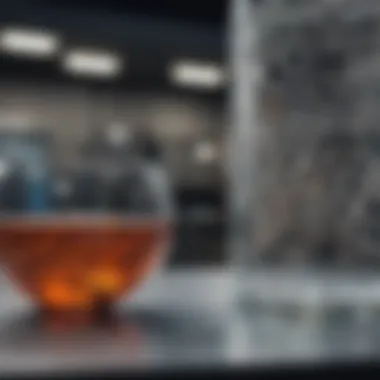
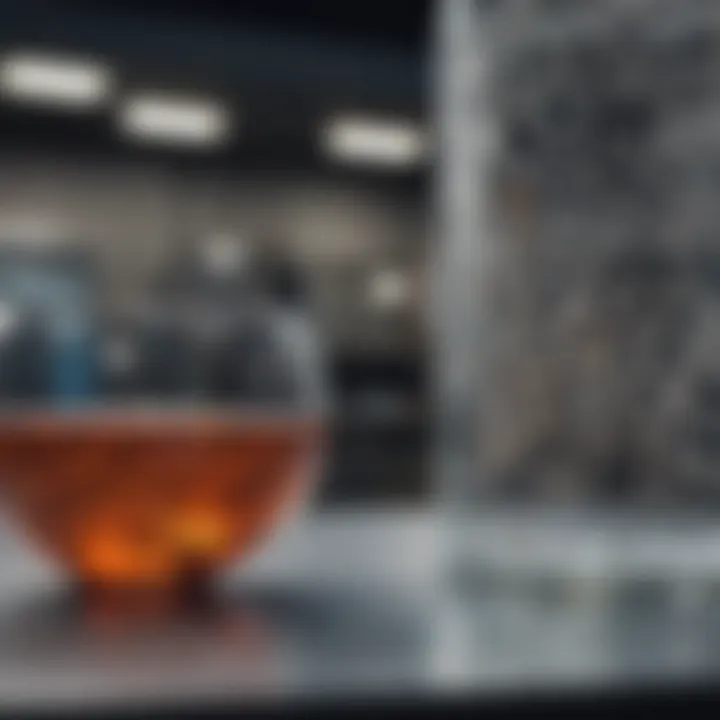
The unique feature is that in many cases, patients may not always be tracked adequately post-approval, leading to potential oversights or delayed responses to complications. As advancements in technology make real-time monitoring possible, it presents an opportunity to improve patient outcomes through continuous assessment, though it requires infrastructure that can support such initiatives.
In navigating these challenges, the tissuetech landscape offers a complex interplay of opportunities and hurdles, holding both the promise of innovation and the weight of responsibility. Each concern outlines a rich tapestry of variables that must be considered as the field advances, ensuring that every step taken serves both science and society.
Future Directions in Tissuetech
The future of tissuetech holds a transformational promise, acting as a beacon for advancements in biomaterials and tissue engineering. As science inches closer to achieving life-like tissue replicas and integrated medical solutions, the implications are vast. This section will discuss several innovative research approaches and market trends that signify shifts in how we conceive of regenerative medicine and its applications.
In understanding tissuetech's evolving landscape, it is essential to highlight the key elements driving these changes: personalized medicine, nanotechnology, and the integration of artificial intelligence. All these advancements are aimed at revolutionizing patient care, optimizing treatment outcomes, and streamlining production processes in biotech and healthcare.
Innovative Research Approaches
Personalized Medicine
Personalized medicine stands at the forefront of modern medical practice, aiming to tailor treatment plans to individual patient needs rather than adopting a one-size-fits-all approach. This methodology considers a person's unique genetic makeup, environmental factors, and lifestyle. A key characteristic of personalized medicine is its focus on improving specific patient outcomes. By integrating data from diverse sources, researchers can create custom biomaterials or cell therapies that align with the biological and chemical markers of each patient.
The advantage of such an approach is clear: improved efficacy with minimized side effects, creating a more favorable healing environment. Yet, challenges persist including the need for extensive biomarker databases and the complexities involved in tissue engineering.
Nanotechnology in Tissuetech
Nanotechnology has emerged as a game-changer in tissuetech, enhancing the design and functionality of biomaterials. The ability to manipulate materials at the nanoscale allows for the creation of scaffolds that mimic the natural environment of cells more accurately. A prominent aspect of nanotechnology is its capacity to facilitate controlled drug delivery systems, which can fine-tune the release of medications over time, reducing the risk of toxicity while maximizing therapeutic effects.
However, while the prospects are promising, one must consider the unique feature of nanotechnology: its complexity and potential health risks associated with nanocomponents. Balancing the benefits against these concerns is crucial in ongoing research.
Integration with Artificial Intelligence
Artificial intelligence (AI) is carving out a substantial niche in tissuetech innovation, primarily through data analysis, modeling, and predictive analytics. This integration helps researchers to process vast amounts of biological data to identify trends that human analysis might miss. A cornerstone of AI's application lies in its ability to simulate biological systems, aiding the design of biomaterials that closely replicate human tissue characteristics.
One advantage of using AI is the accelerated pace of research and potential for breakthroughs stemming from data insights. Nevertheless, relying heavily on AI could pose risks concerning data privacy and ethical considerations surrounding patient information.
Market Trends and Future Outlook
Commercialization Strategies
Commercialization strategies play a pivotal role in translating lab discoveries into market-ready products. The biomaterials industry is particularly buoyed by the establishment of clear pathways that streamline this transformation. A notable feature is cross-industry collaborations, where biotechnological firms partner with healthcare providers to bridge gaps between research and application.
Such strategies are beneficial because they create a more viable approach to market entry, though they also carry risks related to shared intellectual property and differing organizational goals.
Investment in R&
Investment in research and development is crucial for the growth of tissuetech. Companies and institutions channel funds toward innovative biomaterial designs and effective application methods. This trend is fueled by the recognition of tissuetech's potential to address significant health challenges.
While bolstering innovation, heavy investment can also strain budgets, especially for smaller companies, necessitating strategic financial planning.
Global Market Potential
The global market potential for tissuetech is substantial and expanding, driven by increasing demands for organ transplants, personalized therapies, and effective treatment solutions for chronic diseases. Notably, emerging markets are beginning to invest in tissuetech, recognizing its potential for enhancing national healthcare frameworks.
The key characteristic of this growth is its scalability across different regions. While there are promising signs, the global aspect also requires navigating complex regulatory environments across various countries, creating both opportunities and hurdles in market expansion.
Ending
As we draw the curtain on our exploration of tissuetech and its role in biomaterials and tissue engineering, it’s clear this field is not just a passing fad; it’s a cornerstone of modern medicine and material science. The advancements we've delved into underline how crucial innovation is in shaping the future of health care.
Biomaterials and tissue engineering are intertwined with the quest for improved health solutions. They serve as the bedrock for a variety of applications, from implants that can replace failing organs to drug delivery systems that ensure precise medication timing. The ability to tailor materials to meet patient-specific needs makes these innovations especially compelling.
Key highlights from our discussion include the essence of 3D printing and bioprinting in creating complex tissue structures, the importance of ethical considerations in human trials, and the ongoing challenges of regulatory efficacy. As industry stakeholders grapple with these implications, the horizon looks promising.
The future of tissuetech is bright, lined with opportunities for personalized medicine and integration of sophisticated technologies like artificial intelligence. This lays the foundation for a world where healing is both efficient and humane. We need to stay vigilant, however, as with any advancement, the balance between innovation and ethical responsibility remains paramount.
In essence, understanding these dynamics equips students, researchers, educators, and professionals with critical insights into how tissuetech is evolving. As we step into the next phase of biomedicine, let us embrace the intersection of technology and biology, which holds the potential to transform lives profoundly.
"Innovation is the ability to see change as an opportunity - not a threat."
Tissuetech exemplifies this tenet, propelling us toward a future filled with hope and possibilities.
Benefits of A Well-Curated Reference List
- Credibility: References establish trustworthiness in the content.
- Depth: They provide avenues for deeper exploration of specific topics.
- Context: Offer contextual understanding of evolving technologies.
Additionally, harvesting knowledge from general platforms like Wikipedia, Britannica, Reddit, and Facebook can also complement academic sources. While these sources may not be peer-reviewed, they can yield contemporary insights and highlight the societal discourse surrounding tissue engineering innovations. Fact-checking and cross-referencing is recommended to ascertain the accuracy of information obtained from these platforms.
Ultimately, the importance of references in this article extends far beyond number crunching and empirical studies. They play a crucial role in shaping the narrative of the evolving landscape of tissuetech, allowing students, researchers, and professionals to engage with the material effectively. By fostering a well-rounded understanding through diverse sources, the next generation of innovators and practitioners can effectively contribute to the ongoing discourse in biomaterials and tissue engineering.