Exploring Different Types of Power Electronic Converters
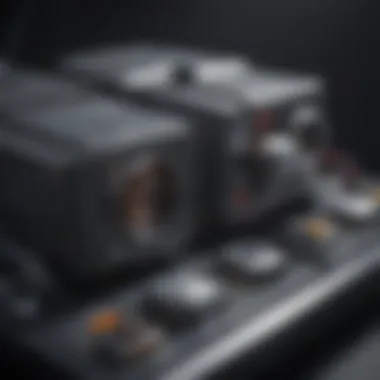
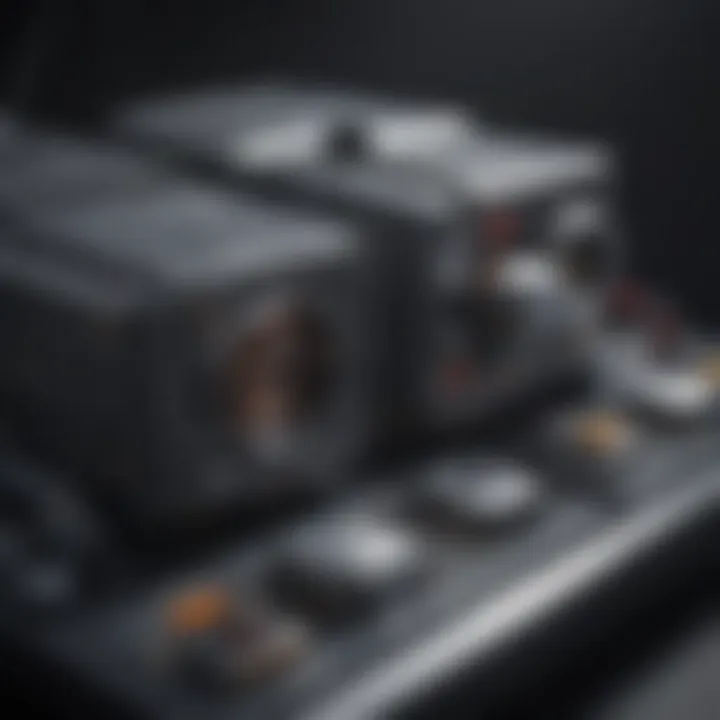
Intro
Power electronic converters are essential components in modern electrical systems. They bridge the gap between different types of energy sources, control energy flow, and enhance efficiency across a variety of applications. Understanding the types of these converters is crucial for students, researchers, and professionals engaged in the field.
The world of power electronics constantly evolves with new technologies emerging. As they become more complex, recognizing the operational principles and applications of these converters aids in their effective utilization. From simple classifications to intricate topologies, this exploration captures a wide spectrum of power electronic converters, their significance, and performance characteristics.
Research Overview
Summary of Key Findings
This section will help elucidate the core aspects of power electronic converters. Several significant findings emerge:
- Diverse Classifications: The converters can be categorized based on functionality, topology, and energy source.
- Application Versatility: They find use in renewable energy systems, power grids, electric vehicles, and consumer electronics.
- Technological Advancements: Innovations in semiconductor materials and control strategies enhance their efficiency and reduce costs.
Research Objectives and Hypotheses
- Analyzing the various classifications of converters.
- Discussing operational principles, application areas, and performance metrics.
- Evaluating recent advancements and their implications for future developments.
The primary hypothesis is that understanding these converters better will lead to improved design choices and innovations in the power electronics sector.
Methodology
Study Design and Approach
This research takes a qualitative approach by synthesizing existing literature, case studies, and technical papers within the field. It involves a comprehensive review of methodologies employed in power electronic innovations.
Data Collection Techniques
Data were gathered from multiple reputable sources, including academic journals and industry reports. This ensures a well-rounded perspective of the current landscape in power electronic converters.
Prelims to Power Electronics
Definition and Overview
Power electronics primarily deals with the study of devices that manage and convert electrical energy in different forms. The converters can modify voltage, current, or frequency while leveraging semiconductor components such as diodes, transistors, and thyristors. These devices are pivotal in enhancing the overall efficiency of power systems. In simpler terms, power electronic converters transform energy from one form to another, adapting it as per the system requirements.
Importance in Modern Applications
The importance of power electronics cannot be overstated in todayβs rapidly advancing technological landscape.
- Efficiency: Power electronic converters allow for high-efficiency energy conversion. They minimize losses during transmission and usage of power, which is crucial in applications such as electric vehicles and renewable energy.
- Control: They offer precise control of electrical parameters, which is important for applications like motor drives and power supply systems.
- Integration: With the rise of smart grids and IoT devices, power electronics helps integrate renewable sources like solar and wind into existing grids, making them more reliable and efficient.
In essence, as electrical systems continue to become more advanced, the relevance of power electronics intensifies, establishing itself as a cornerstone in both current and future technologies.
Basic Classification of Power Electronic Converters
Understanding the classification of power electronic converters is crucial in appreciating their diverse applications and operational principles. The basic classification delineates the converters based on their input and output types of electrical power. This structure not only simplifies the complex realm of power electronics but also paves the way for deeper insights into the workings of advanced converters. By categorizing the converters, one can discern their roles in various applications, from renewable energy solutions to electric vehicle drives. This classification serves as a fundamental framework for both academic studies and practical implementations in the field.
AC to Converters
AC to DC converters, also known as rectifiers, are pivotal in converting alternating current (AC) to direct current (DC). This conversion is essential for applications requiring stable DC power, such as battery charging and powering electronic devices. These converters use diodes to allow current to flow in one direction only, thus converting AC voltage into DC voltage.
There are different types of rectifiers, including:
- Half-wave rectifiers: These utilize a single diode, allowing only one half of the AC cycle to pass through. They are simple but inefficient due to their low output.
- Full-wave rectifiers: These use two or more diodes, effectively utilizing both halves of the AC cycle. This results in higher efficiency and reduced ripple in the output voltage.
AC to DC conversion is vital in daily life, impacting everything from household appliances to large industrial operations. Understanding this conversion process is foundational for further exploration of power electronic systems.
to AC Converters
DC to AC converters, commonly referred to as inverters, facilitate the transformation of direct current into alternating current. This conversion is especially important for renewable energy applications, such as solar power systems, where solar panels generate DC power that must be converted for grid compatibility.
Inverters can be classified into:
- Square wave inverters: These produce a simple square wave output, suitable for less sensitive loads but not ideal for many household appliances.
- Sine wave inverters: These generate a smoother and more desirable output waveform, which is preferable for sensitive electronic equipment.
DC to AC conversion plays a significant role in power quality, ensuring that the electrical supply meets the required standards for various applications.
to Converters
DC to DC converters are designed to adjust the voltage level of a direct current source. These converters are critical in applications where a specific voltage level is necessary, such as in battery management systems or electronic circuits that require different voltage levels.
Common types of DC to DC converters include:
- Buck converters: These step down the input voltage while stepping up the current, making them efficient for battery-powered devices.
- Boost converters: Conversely, these step up the input voltage, essential for applications requiring higher voltage outputs.
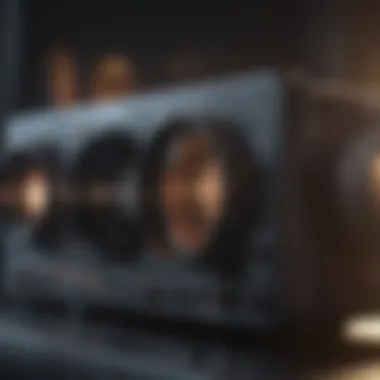
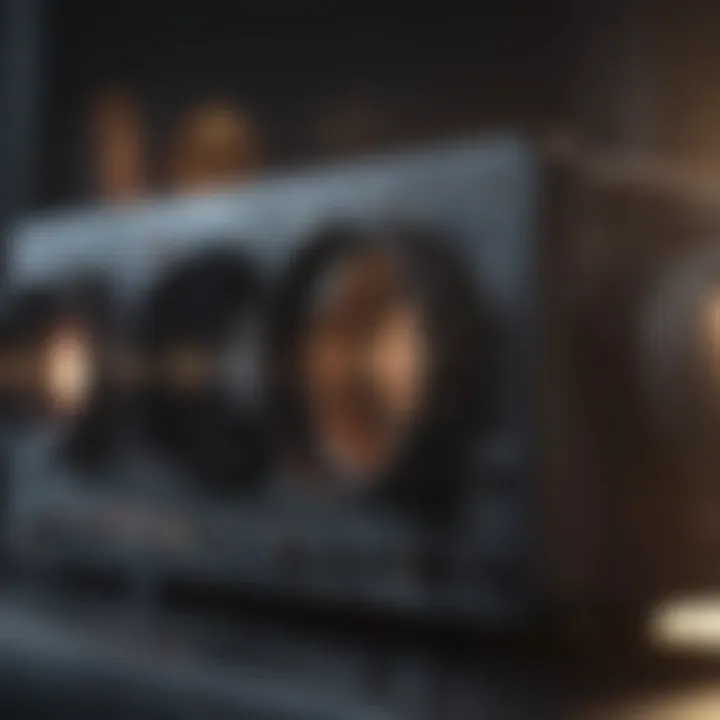
Understanding DC to DC conversion is essential for optimizing power supply systems, enhancing energy efficiency, and improving overall performance.
AC to AC Converters
AC to AC converters modify the characteristics of an alternating current without changing its form between AC and DC. They achieve this transformation through various methods, making it possible to control voltage and frequency in electrical systems.
Key types include:
- AC voltage controllers: These enable control over AC voltage by switching on and off, managing the output effectively.
- Cycloconverters: These directly convert AC power of one frequency to another without an intermediate DC stage, useful in applications like industrial motor drives.
This classification of AC to AC converters highlights their flexible applications, especially in industrial settings where control over AC power is necessary to meet specific requirements.
Detailed Examination of Converter Types
The examination of converter types is critical in understanding the nuances of power electronics. Each category of converter serves a unique purpose and plays a significant role in energy conversion, which is fundamental to various applications in modern technology. By dissecting the various mechanisms, one can appreciate not just their functionalities, but also their efficiencies and limitations. This section aims to provide a thorough exploration of the different types of converters, with a focus on their operational principles and the advantages they bring. Grasping these details is especially beneficial for students and professionals who aim to delve deeper into the field.
AC to Rectifiers
AC to DC rectifiers are essential devices that convert alternating current (AC) into direct current (DC). This conversion is vital for most electronic circuits that require a stable DC supply. The rectification process involves using diodes, which allow current to flow in one direction only, thus blocking the reverse current.
There are different types of rectifiers, including half-wave and full-wave rectifiers. Half-wave rectifiers utilize a single diode, allowing only half of the AC waveform to pass through. This method is simple but inefficient, resulting in increased ripple voltage. In contrast, full-wave rectifiers utilize multiple diodes in a bridge configuration to harness both halves of the AC cycle, leading to better performance and reduced ripple.
"Rectifiers are the backbone of power supply systems, facilitating the needs of electronic devices across the spectrum."
The output of rectifiers can be improved through additional filtering stages, usually involving capacitors. This helps in smoothing out the voltage and providing a more stable output. Key applications include power supplies for consumer electronics and industrial machines.
Inverters for to AC Conversion
Inverters are pivotal in converting DC back to AC, a process necessary for feeding energy into AC grids or powering AC loads such as motors and appliances. The basic function of an inverter is to switch the DC input on and off rapidly, creating a square wave output that can be further smoothed into a sinusoidal output.
There are several types of inverters, including string inverters, microinverters, and central inverters. Each type has its specific applications and advantages. For example, string inverters are commonly used in solar power systems, as they are cost-effective and efficient. Microinverters, on the other hand, are attached to individual solar panels, allowing for maximum energy harvest and flexibility in system design.
Inverters also play a significant role in renewable energy integration and electric vehicle technology, where efficient energy conversion is crucial.
Buck and Boost Converters
Buck and boost converters are types of DC-DC converters that allow for the stepping down or stepping up of voltage levels respectively. Buck converters reduce voltage, making them ideal for applications that require lower voltage outputs. They use a switching element, usually a transistor, and an inductor to store energy, which is discharged at a controlled rate to provide a lower voltage output.
Boost converters work oppositely by increasing voltage levels. These are beneficial in applications where a higher voltage is needed from a lower input supply. Both types of converters utilize pulse width modulation (PWM) control techniques for regulation, ensuring efficiency and reliability across different loads.
These converters find widespread use in battery-operated devices, allowing for longer run times and better performance.
Charge Pumps and Voltage Doublers
Charge pumps and voltage doublers are specialized circuits used to increase voltage levels without the need for inductors. Charge pumps operate by sequentially charging and discharging capacitors through switching components. By controlling the switching frequency, these circuits can achieve higher voltages effectively.
Voltage doublers are a specific type of charge pump that doubles the input voltage. This is particularly useful in low-power applications where space and weight are a concern, such as in mobile devices and portable electronics. Their simplicity and compact size make them an attractive choice for designers looking to optimize circuit performance in constrained environments.
Advanced Converter Technologies
Advanced converter technologies are central to the ongoing evolution of power electronics. These technologies push the boundaries of converter design and increase efficiency, performance, and flexibility in various applications. In this section, we will explore three significant types: multilevel converters, resonant converters, and matrix converters. Each plays a vital role in modern electrical systems, fulfilling specific requirements, and adapting to new challenges.
Multilevel Converters
Multilevel converters have emerged as a prominent solution to the demand for high power and voltage applications. Their architecture allows multiple voltage levels to be synthesized, which leads to several advantages. One of the key benefits is the reduction of harmonic distortion. By utilizing multiple levels, the output waveform more closely resembles a sinusoidal shape. This is crucial in applications where power quality is paramount.
Moreover, multilevel converters can provide higher voltage capabilities with lower device ratings, which reduces component stress and improves reliability. Applications include renewable energy systems, motor drives, and industrial power conversions.
Some common types of multilevel converters include:
- Diode-Clamped Multilevel Converter
- Flying Capacitor Multilevel Converter
- Cascaded H-Bridge Multilevel Converter
These configurations allow engineers to choose the best approach based on specific application needs and constraints. The technological advancements in semiconductors have further enhanced the capabilities of these converters, leading to improved performance and efficiency.
Resonant Converters
Resonant converters offer a unique approach to power conversion by leveraging resonance in their operation. This allows for soft-switching techniques, which significantly minimize switching losses. These converters operate at high frequencies, making them attractive for applications that require compact designs.
The primary types of resonant converters include:
- Series Resonant Converters
- Parallel Resonant Converters
- Series-Parallel Resonant Converters
Their ability to provide high efficiency and minimized electromagnetic interference makes them suitable for applications like telecom power supplies and electric vehicle chargers. However, understanding their operational behavior, such as the load dependence on resonant frequency, is crucial for effective implementation.
Matrix Converters
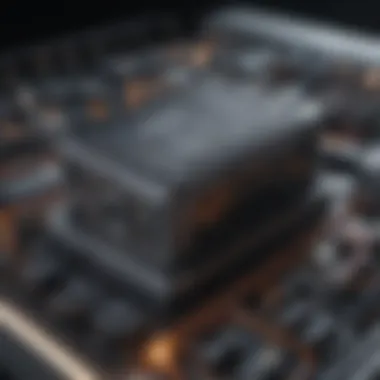
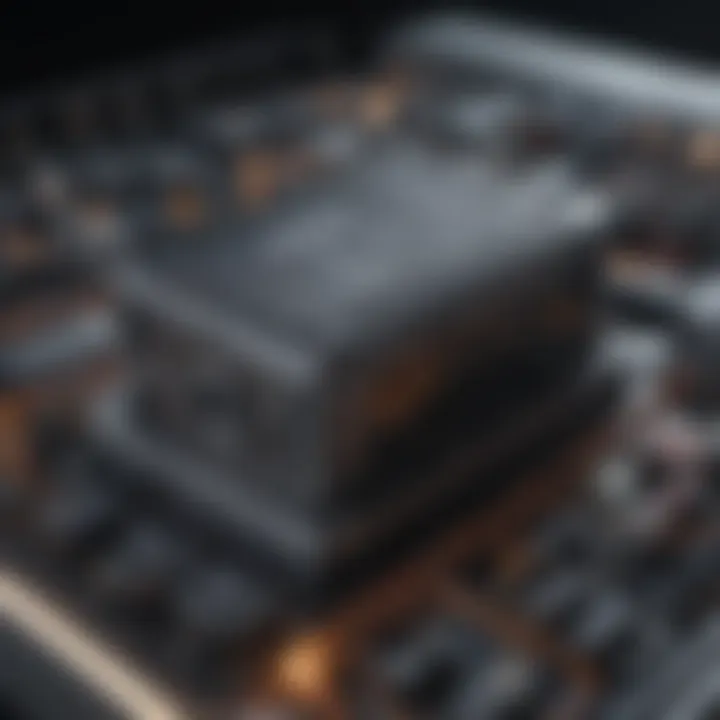
Matrix converters are an innovative converter topology that allows for direct AC to AC conversion without the need for an intermediate DC link. This unique characteristic provides distinct benefits, such as reduced system size and increased efficiency. These converters can handle bidirectional power flow, making them ideal for applications like renewable energy integration.
Despite the advantages, matrix converters also present challenges. They require sophisticated control strategies to manage their non-linear behavior and to ensure proper output regulation. However, advancements in control algorithms have improved the viability of matrix converters in demanding applications such as adjustable-speed drives and power quality enhancements.
"The integration of advanced converter technologies is key to the future of power electronics, enabling new applications and improving existing systems."
In summary, advanced converter technologies significantly enhance the performance and capability of power electronic systems. By employing techniques such as multilevel, resonant, and matrix conversion, engineers can address modern challenges and drive innovation in power applications. Understanding these technologies provides a foundation for future advancements in this essential field.
Control Strategies for Power Converters
Control strategies for power converters are essential for optimizing performance, ensuring stability, and enhancing efficiency in various applications. These approaches help engineers manage the conversion of electrical energy in a more effective manner. They allow for desired output characteristics, improve power quality, and extend the components' operational lifetimes. Understanding these strategies is crucial not just for advanced applications but also for basic designs.
Pulse Width Modulation Techniques
Pulse Width Modulation (PWM) is a widely used technique in power electronics for controlling the output voltage and current. This method involves turning the switch on and off at a high frequency, creating a series of pulses. The width of these pulses determines the average voltage applied to the load, thus allowing for precise control over power delivery.
Some key benefits of PWM include:
- Improved Efficiency: Since the switching components can operate in either fully on or fully off states, losses can be minimized.
- Better Thermal Management: High-frequency switching reduces heat generation compared to linear regulation.
- Flexibility: PWM can be easily adapted for different types of loads and requirements.
PWM can also be implemented in various forms, such as sine PWM or space vector PWM. Each of these has its own advantages based on application requirements. With the advancement of microcontroller technology, implementing PWM in converter designs has become more accessible and efficient.
Sliding Mode Control
Sliding Mode Control (SMC) is another powerful control method used in power electronics which enhances system robustness against uncertainties. This approach manipulates system dynamics by forcing the state trajectory to slide along a predefined surface. Once the system reaches this sliding surface, it behaves predictably despite external disturbances.
Some notable characteristics of SMC are:
- Robustness: It excels in environments with parameter variations or disturbances, making it suitable for real-time applications.
- Simplicity: The implementation of SMC can be straightforward, especially for systems where the state dynamics are known.
- Quick Response: SMC provides fast control action, which is beneficial for applications requiring rapid changes.
However, SMC can lead to high-frequency oscillations, known as chattering. This requires careful design to mitigate unwanted behavior while still utilizing the benefits of sliding control.
Fuzzy Logic Control
Fuzzy Logic Control (FLC) provides another alternative to traditional control methods, using logic that resembles human reasoning. FLC handles imprecise inputs and can operate in environments with uncertainty. It does this by employing a system of rules that evaluate different conditions and produce outputs based on degrees of truth.
The primary advantages of FLC include:
- Non-linear Control: It can handle non-linear systems effectively without needing a precise model.
- Adaptability: FLC can adjust to changes in system dynamics or environmental conditions, maintaining performance.
- Ease of Design: Developing a fuzzy logic controller can often be simpler than deriving a conventional control strategy.
FLC is particularly appropriate for complex systems found in renewable energy systems or electric vehicle drives, where traditional control techniques may struggle. Its ability to manage uncertainties provides engineers with a robust tool in their design arsenal.
The understanding of control strategies for power converters is fundamental in navigating the complexities of power electronics today.
These control methods are just a few examples of the strategies employed in power converters. Each has specific implications for design and application, highlighting the importance of selecting the right approach based on system requirements. As technology progresses, these strategies will continue to evolve, making power electronics an exciting area of exploration.
Applications of Power Electronic Converters
Power electronic converters play a central role in a wide range of applications that drive modern electrical systems. Their ability to efficiently manage and convert electrical energy is critical. This section covers some significant areas where these converters are instrumental. Each application offers unique benefits, highlights specific challenges, and shows how advancements in power electronics technology continue to evolve.
Renewable Energy Systems
Renewable energy systems rely heavily on power electronic converters for the efficient transformation and integration of energy sources such as solar and wind. In photovoltaic systems, DC to AC converters, known as inverters, are essential for converting the generated solar energy into electricity usable by the grid.
Similarly, in wind energy systems, converters manage the variable nature of wind input, maximizing energy capture from turbines. The efficiency of these converters impacts the overall performance of renewable energy systems significantly, making ongoing improvements in converter design, control strategies, and material technologies crucial for wide-scale adoption.
Electric Vehicle Drives
As the shift towards electric vehicles (EVs) accelerates, the role of power electronic converters is becoming increasingly vital. They facilitate the conversion of battery-stored DC power to AC power required for electric motors. Modern electric vehicles utilize sophisticated inverters that enhance energy efficiency and power control.
Additionally, regenerative braking systems in EVs harness energy typically lost during braking, converting it back to usable power. Effective management of these processes optimizes vehicle range and performance. Continuous enhancements in converter technologies are essential for improving the functionality and reliability of electric drive systems.
Industrial Motor Drives
Power electronic converters dominate industrial applications, particularly in motor drives. These converters are employed to adjust the speed and torque of electric motors based on specific operational needs. Variable Frequency Drives (VFDs) exemplify this, controlling the frequency of the electrical supply to the motor.
This capability allows for energy savings and performance optimization in various industries including manufacturing, HVAC systems, and pumping applications. Properly designed motor drives improve energy efficiency, contribute to extended equipment life, and minimize environmental impacts. Ongoing technological advancements are vital to meeting the growing demand for energy-efficient industrial solutions.
Power Quality Improvement
Power quality issues manifest in various forms, affecting reliability and stability in electrical systems. Power electronic converters help maintain power quality by providing solutions such as active power filtering and voltage regulation. These solutions mitigate harmonics, voltage sags, and other disturbances.
For instance, Static Synchronous Compensators (STATCOMs) use power electronics to enhance reactive power support and stabilize voltage levels in transmission systems. By improving power quality, converters contribute to the overall reliability of electrical infrastructure, making them integral to both existing and emerging energy grids.
Challenges and Limitations
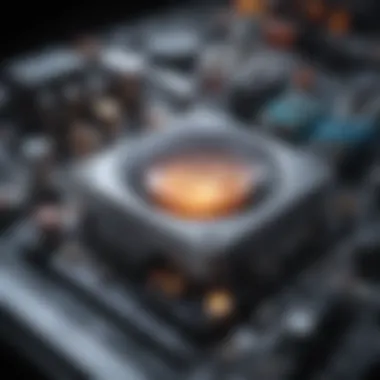
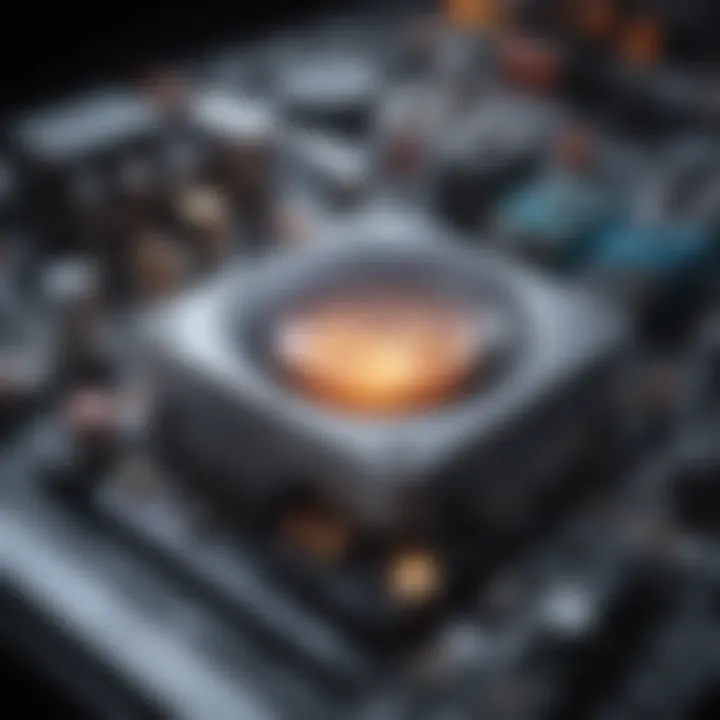
Power electronic converters play a critical role in modern electrical systems, but they are not without their challenges and limitations. Understanding these issues is necessary to improve their designs and applications. From efficiency losses to thermal management, these challenges can significantly affect performance, reliability, and overall effectiveness. Recognizing and addressing these problems allow designers and engineers to enhance converter performance and ensure their integration into various applications.
Efficiency and Losses
Efficiency is a significant concern for power electronic converters. Generally, power converters should operate well above 90% efficiency. However, several factors contribute to energy losses.
Some primary sources of efficiency losses include:
- Conduction losses: Resistive elements in power switches contribute to energy losses when current flows through them.
- Switching losses: Rapid transitions between on and off states result in power loss during the switching processes, especially at high frequencies.
- Magnetic losses: Core losses in inductors and transformers occur due to hysteresis and eddy currents.
To mitigate these losses, engineers often optimize designs by using advanced materials, improving cooling systems, and employing sophisticated control strategies. Maximizing efficiency is crucial because every increment in efficiency translates to reduced energy consumption and better performance.
Thermal Management Issues
Thermal management has become increasingly important due to the high power densities in modern converters. As devices handle larger amounts of power, they generate heat that can degrade performance and shorten lifespan. Effective thermal management strategies include:
- Heat sinks: These are designed to dissipate heat away from the device into the surrounding environment.
- Active cooling: In some applications, fans or liquid cooling systems help manage heat generation, ensuring the optimal operating temperature.
- Thermal insulation: This technique prevents heat loss, ensuring that critical components remain at their required temperatures.
Neglecting thermal management can lead to catastrophic failure of the system. Hence, understanding how to effectively manage heat is vital to maintaining converter reliability and longevity.
EMI and EMC Considerations
Electromagnetic interference (EMI) and electromagnetic compatibility (EMC) issues present important challenges in the design of power electronic converters. The rapid switching of voltages and currents in these devices can generate unwanted electromagnetic fields that interfere with surrounding electronic components.
Key measures for managing EMI and ensuring EMC include:
- Filtering: Using filters can minimize high-frequency noise from escaping or entering a converter.
- Shielding: Enclosing sensitive components in conductive materials can reduce interference.
- Layout design: Thoughtfully routing traces on a printed circuit board can help reduce the effects of parasitic capacitance and inductance.
Designers must prioritize EMI and EMC regulations to meet industry standards. Addressing these factors ensures functional reliability and robust performance in power electronic converters.
Future Trends in Power Electronics
The realm of power electronics is undergoing rapid evolution, driven by demands for increased efficiency and enhanced integration in electrical systems. Future trends are pivotal in shaping the landscape of power converters, influencing both their design and application.
Integration with Smart Grids
Smart grids represent a fundamental shift in how electricity networks operate. The integration of power electronic converters with smart grids enables real-time management of energy flow and optimal utilization of renewable sources.
- Dynamic Load Management: With the right converters, grid operators can manage loads dynamically, adjusting to fluctuations in supply and demand.
- Increased Resilience: Smart grids equipped with power converters can withstand disruptions more effectively, ensuring reliable power supply even in adverse situations.
- Distributed Generation: Converters facilitate the integration of distributed energy resources. This means more solar panels and wind turbines can be connected to the grid, promoting sustainability.
Overall, the integration enhances grid efficiency and supports the broader goal of achieving energy independence.
Advancements in Semiconductor Materials
The ongoing research and development of semiconductor materials play a vital role in the progression of power electronic converters. Advanced materials such as silicon carbide (SiC) and gallium nitride (GaN) are gaining prominence. These materials are central to enhancing performance metrics for converters.
- Higher Efficiency: SiC and GaN devices exhibit lower switching losses, contributing to overall better energy efficiency.
- Temperature Resilience: These materials manage heat more effectively, resulting in improved thermal performance and reduced cooling requirements.
- Compact Design: The capacity to operate at higher voltages and frequencies allows for more compact converter designs, saving space and reducing costs.
Such advancements not only lower operational costs but also lead to a reduced environmental impact by enabling more sustainable practices in power electronics.
Role of AI in Power Electronics
Artificial intelligence is emerging as a transformative force within power electronics, especially in the operation and management of converters. The use of AI can enhance the performance of power electronics in several ways:
- Predictive Maintenance: AI algorithms can analyze performance data to foresee potential failures before they occur. This proactive approach minimizes downtime and maintenance costs.
- Optimized Control Strategies: AI can fine-tune control strategies for converters dynamically, assisting in enhancing efficiency further.
- Data Analytics: The ability to process vast amounts of operating data leads to improved decision-making, optimizing operational parameters based on real-time conditions.
The convergence of AI with power electronics opens pathways for innovations that were unimaginable a few years ago. This melds greatly with trends toward increased automation and smart system integration.
"The future of power electronics is synergized with advancements in technology and systemic integration, ensuring sustainable growth in the energy sector."
In summary, the future of power electronics lies in smarter, more efficient devices that align with global energy goals. These trends shape power converters into pivotal components of modern electrical networks, catering to an ever-evolving landscape of energy needs.
Epilogue
The conclusion of this article plays a vital role in encapsulating the vast information presented about power electronic converters. Throughout the sections, we have explored different types of converters, their operational principles, applications, and the challenges they face. Highlighting this information allows readers to consolidate their understanding and assess the relevance of power electronic converters in contemporary technology.
One key benefit of having a well-structured conclusion is that it serves to reinforce the knowledge gained. Readers can revisit the essential points and see how the different types discussed contribute to emerging technologies like electric vehicles and renewable energy systems. Additionally, a thoughtful synthesis can prompt further exploration of topics, encouraging students and professionals to delve deeper into the innovative aspects of power electronics.
In considering the importance of the conclusion, it is crucial to recognize that this section aids in cementing the article's contribution to the readerβs knowledge. The accumulation of information can lead to practical applications and inspire new research avenues. As we look toward the future, understanding the foundational elements and trends in power electronic converters becomes essential in harnessing their capabilities in various industries.
"The future of power electronics is a reflection of the innovations today, where understanding converter technologies lays the groundwork for advancements tomorrow."
Summary of Key Points
In this article, we have covered several key points that are essential to understanding power electronic converters:
- Types of Converters: Explored the various classifications, including AC to DC, DC to AC, DC to DC, and AC to AC converters. Each type performs specific functions based on the nature of input and output voltages.
- Operational Principles: Detailed the working principles of converters, emphasizing their electronic components and control methods. Awareness of these principles is critical for selecting the appropriate converter for a given application.
- Applications: Highlighted real-world implementations such as renewable energy systems, electric vehicles, and industrial motor drives.
- Challenges: Discussed the limitations in terms of efficiency, thermal management, and electromagnetic interference. Awareness of these challenges is crucial for ongoing development in the field.
- Future Trends: Considered the evolution of power electronics driven by developments in materials and integration with smart grids, as well as the potential impact of artificial intelligence.
Looking Ahead
Looking ahead, the domain of power electronics is poised for significant advancements. The intricate interconnections between power electronics and other emerging technologies, particularly in sustainable energy and smart infrastructure, will drive future developments.
- Technological Innovations: Continuous advancements in semiconductor materials and control technologies are expected to enhance converter efficiency and reduce costs.
- Integration: Greater integration with smart grid technologies will enable more efficient energy distribution and management, highlighting the role of power electronic converters in the modern electrical ecosystem.
- Role of AI: Artificial intelligence may play a transformative role in optimizing the performance of power electronic devices, allowing for smarter, adaptable systems that respond to real-time data.
In summary, understanding the various aspects of power electronic converters equips professionals and researchers with the necessary knowledge to navigate future challenges and innovations in the field. As technology continues to evolve, staying informed about these developments will be crucial for leveraging power electronics effectively.