Electromyography: Understanding Muscle Function and Innovations
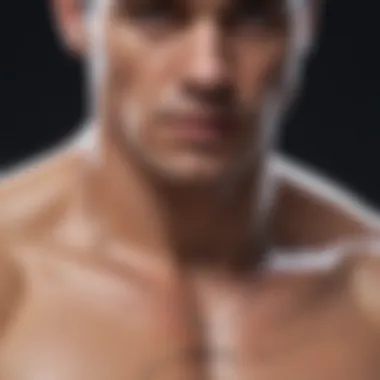
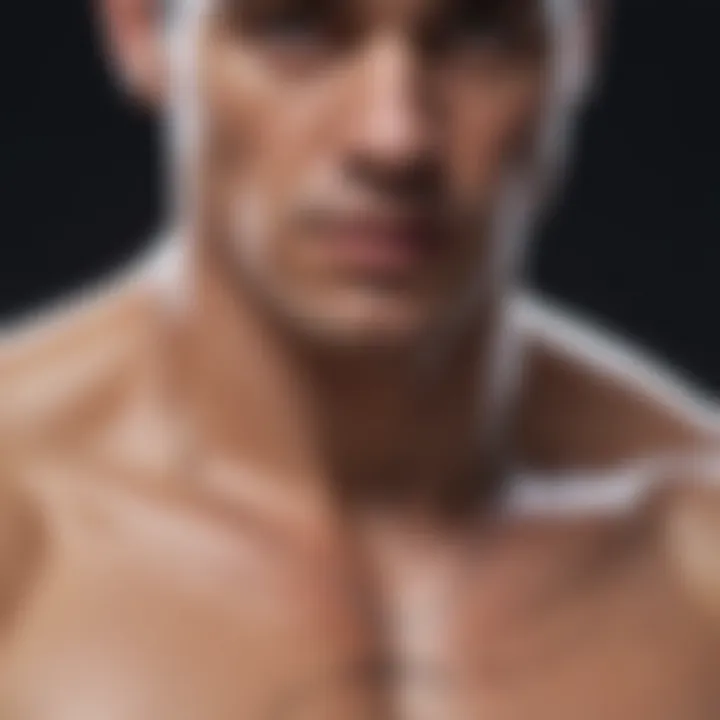
Intro
Electromyography (EMG) has taken center stage in the realm of muscle function analysis and neuromuscular disorder diagnostics. This technique offers a window into the electrical activity of muscles, revealing insights that were considered elusive not so long ago. The journey we embark on in this exploration aims to illuminate the principles underpinning EMG, while also examining the myriad applications of this significant technology.
In an age where advancements in science and technology continue to push boundaries, understanding EMG's principles and applications is crucial. Whether you're a student peering into the depths of muscle biomechanics, a researcher seeking innovative methodologies, or a healthcare professional striving for accurate diagnoses, this guide serves your curiosity.
We will dissect how EMG operates fundamentally, connecting the electrical impulses generated by muscle fibers to the larger picture of human health and athletic performance. From clinical diagnostic settings to cutting-edge applications in sports science and rehabilitation, the breadth of EMG's significance is almost staggering. As we delve deeper, keep an eye out for the innovations that promise to reshape how we perceive and utilize electromyography.
"Understanding muscle signals through EMG remains pivotal in making strides towards enhancing health and athletic performance."
Our exploration is meticulously structured, providing a comprehensive narrative designed to deliver clarity and depth. This begins with a research overview that highlights key findings and objectives pertinent to electromyography, laying the groundwork for further investigation into its methodologies and applications. Let's proceed, step by step, in unraveling this fascinating subject.
Prelude to Electromyography
Electromyography, or EMG as it is commonly known, serves as a cornerstone in both clinical and research settings for muscle function assessment. This technique provides insights not only into how muscles operate but also into how they fail. Understanding EMG is crucial for medical professionals and researchers alike, as it opens a window into the vast and intricate world of neuromuscular disorders, rehabilitation practices, and even sports science.
To kick things off, let’s delve into the historical backdrop of this fascinating technique. How did it all begin? What role has it played in medical advancements? These questions set the stage for a rich discussion around EMG.
Definition and Historical Context
Electromyography is defined as a diagnostic procedure that assesses the health of muscles and the nerve cells that control them. It detects electrical activity in muscle tissues when they are activated. This activity provides a treasure trove of information, revealing how nerves communicate with muscles, and how muscles respond to that communication.
The roots of EMG trace back to the late 19th century. In 1849, Guillaume Duchenne, a pioneering French neurologist, laid down the first principles of the technique. He was among the first to demonstrate that muscles could be stimulated electrically, which was revolutionary at the time. Fast forward to the 20th century, and advancements in technology allowed for more sophisticated methods of capturing and interpreting electomyographic signals. Today, surface electrodes and intramuscular electrodes provide a wide range of data on muscle activity, significantly aiding in diagnostic efforts.
Just a century ago, this kind of analysis was largely the stuff of dreams. The electrical nature of our bodies was little understood, and medical professionals often had to rely on physical observations alone. Presently, we have devices that can record EMG signals in real time, yielding immediate insights into muscle health and function. This evolution shows not just technological advancement, but a profound appreciation of the human body's complexities.
Significance in Medical Science
The significance of electromyography in the medical realm cannot be overstated. For starters, it plays a pivotal role in the diagnosis of a variety of neuromuscular diseases, such as amyotrophic lateral sclerosis (ALS), myasthenia gravis, and peripheral neuropathies. EMG allows physicians to determine whether the muscle weakness is stemming from a problem with the muscle itself or with the nerve that supplies it.
Moreover, EMG findings can guide treatment decisions. Clinicians use the data to tailor rehabilitation programs for patients recovering from injuries, surgeries, or chronic conditions. For instance, physical therapists often rely on EMG readings to monitor muscle activation patterns, ensuring that their therapeutic interventions are effective. In addition to diagnosis and treatment, EMG serves as a critical tool for research. Investigating how muscles behave under various conditions—like fatigue, injury, or stimulatory therapies—fuels advancements in restoring muscle function.
To highlight the importance of this technology, consider the following points:
- Diagnosis: It aids in pinpointing neuromuscular disorders that might otherwise go undetected.
- Therapeutic Guidance: Customizing rehabilitation strategies based on actual muscle function data.
- Research Applications: Facilitating groundbreaking studies that enhance understanding of muscle mechanics.
"The electrical signals produced by muscles are not just data points; they narrate a story about our physical state, both in health and disease."
In summary, the introduction of electromyography marks a significant milestone in our quest to understand neuromuscular function, impacting diagnosis, treatment, and research in profound ways. The evolution of EMG from a nascent idea to a sophisticated tool is not only a testament to human ingenuity but also highlights its essential role in the future of medical science.
Principles of Electromyography
Understanding the principles of electromyography (EMG) is cornerstone to grasping its applications in both clinical and research settings. EMG serves as a bridge between muscle activity and electrical signal interpretation. This section delves into the neurophysiology that underlies muscle contractions, as well as the various methods used to acquire these vital signals.
Neurophysiology of Muscle Contraction
At the heart of electromyography lies the neurophysiology of muscle contraction. When a motor neuron fires, it sends an action potential along its axon to the neuromuscular junction where it triggers the release of acetylcholine. This neurotransmitter binds to receptors on the muscle fiber membrane, leading to muscle contraction. This fundamental process involves an intricate interplay between the nervous system and muscular mechanics, which EMG aims to capture.
Key Points about Muscle Contraction and EMG:
- Action Potential: The initial triggering element that leads to muscle fiber contraction.
- Neuromuscular Junction: The site where muscle and nerve meet, essential for effective contraction.
By understanding these basic principles, researchers and clinicians can accurately interpret EMG signals and assess muscle function.
Signal Acquisition Techniques
Signal acquisition is critical in electromyography, as inaccuracies can lead to flawed interpretations of muscle activity. The methodologies can mostly be categorized based on the positioning of electrodes and the type of signals they record. The three main techniques include surface electromyography, intramuscular electromyography, and needle electromyography.
Surface Electromyography
Surface electromyography (sEMG) involves placing electrodes on the skin above the muscle of interest. One of its leading advantages is non-invasiveness, making it a popular choice in both clinical and research phases. Its ease of application allows for wider testing in diverse settings, from sports science labs to rehabilitation clinics.
Key Characteristics of Surface Electromyography:
- Non-Invasive: No needles required, making it suitable for almost any patient demographic.
- Accessibility: Simple setup enables multiple readings, fostering larger dataset collection.
However, it does have drawbacks. The positioning of the electrodes can influence results, and crosstalk from nearby muscles can lead to interpretation challenges, particularly in intricate movements.
Intramuscular Electromyography
Intramuscular electromyography (iEMG) delves deeper by inserting a fine wire electrode directly into the muscle. This technique provides high-fidelity signals, cutting down on noise from adjacent muscles. The key characteristic here is precision, making it immensely beneficial when analyzing specific muscle units.
Key Characteristics of Intramuscular Electromyography:
- High Fidelity: Captures precise muscle activity with less interference from neighboring muscles.
- Targeted Analysis: Useful for detailed studies such as those focusing on small muscle groups.
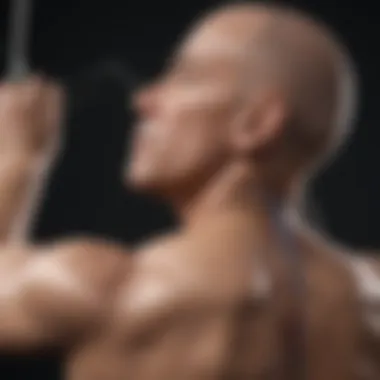
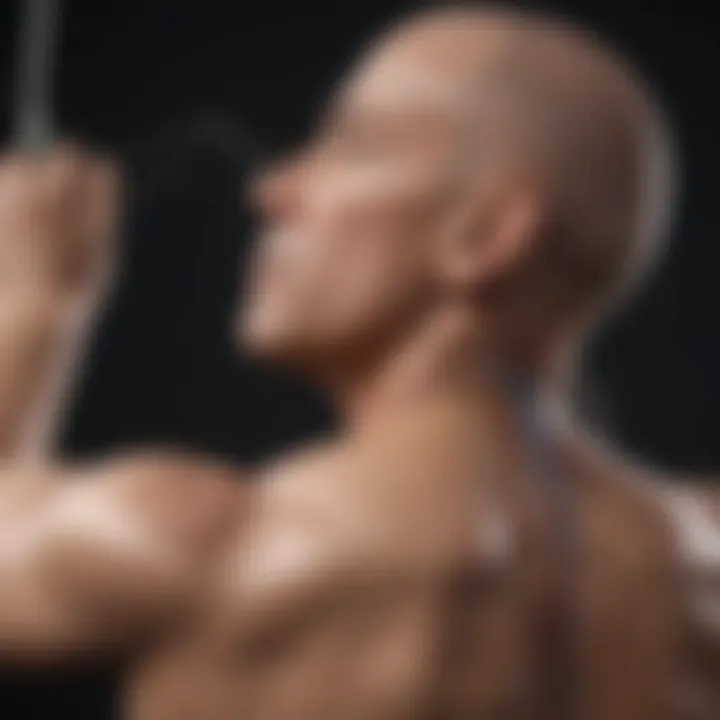
Nevertheless, this technique comes with challenges such as invasiveness, which could cause discomfort or even reluctance in some patients, especially in pediatric populations or those with phobias related to needles.
Needle Electromyography
Needle electromyography is closely related to intramuscular methods. It employs the use of a thin needle electrode inserted directly into the muscle, which captures electrical signals more effectively than surface methods. One of its distinctive features is its ability to provide information about single muscle fibers, offering insight that broader techniques cannot.
Key Characteristics of Needle Electromyography:
- Detailed Measurements: Can assess small, localized areas of muscle activity, yielding valuable diagnostic information.
- Diagnostic Utility: Particularly useful in distinguishing between different neuromuscular disorders.
However, like iEMG, it’s invasive, requiring careful handling to minimize risk of infection or discomfort.
"Understanding the principles of EMG and the nuances of its techniques is fundamental in providing accurate diagnoses, and revealing the intricacies of muscle function."
By considering these techniques, one can grasp the breadth of electromyography’s capabilities and limitations. Each method holds its own merits and pitfalls; thus, selecting the proper strategy is paramount depending on the specific application or research focus. The next section will elaborate on the data methodologies used in processing the signals gathered from these techniques.
Methodologies in Electromyography
Electromyography (EMG) stands at the crossroads of neurophysiology and practical applications, fundamentally influencing how we understand muscle function and dysfunction. The methodologies employed in EMG are crucial, determining the precision of data collected and ultimately shaping clinical outcomes. This section delves into the heart of EMG methodologies, highlighting the importance of rigorous data collection, sophisticated signal processing, and meticulous result analysis.
Data Collection Procedures
Data collection in electromyography is foundational, providing raw signals that reflect muscle electrical activity. The methods adopted can significantly impact the reliability of the results. When collecting data, the objective is to ensure that the recorded signals are as clean and representative of the underlying muscle activity as possible. This often involves selecting appropriate electrode types, placement, and preparation protocols.
Successful data collection rests on a few key considerations:
- Electrode Selection: Surface electrodes are typically employed for non-invasive studies, while fine-wire or needle electrodes may be used for more invasive applications, particularly in diagnosing specific neuromuscular disorders.
- Skin Preparation: Proper preparation is essential to optimize conductivity. This can include cleaning the skin with alcohol or abrading it slightly to reduce impedance.
- Interference Management: During data collection, care must be taken to minimize external interference from electrical devices or movement artifacts.
Signal Processing Techniques
Signal processing techniques are essential for enhancing the quality of the collected data, allowing for a more accurate interpretation of muscle activity. The following are crucial components of this stage:
Filtering
Filtering is pivotal in electromyography, acting as a gatekeeper that allows only relevant frequency ranges to pass through while blocking unwanted noise. This process helps in isolating meaningful muscle signals from electrical interference, ensuring cleaner data for analysis.
- Key Characteristic: The ability to remove high-frequency noise while maintaining the integrity of low-frequency muscle signals makes filtering a no-brainer in any EMG study.
- Unique Feature: One popular filtering method is the bandpass filter, which only permits signals within a specific frequency range to be analyzed, thus preventing unwanted artifacts from corrupting the data.
- Advantages/Disadvantages: The main advantage of filtering is improved data clarity, which is crucial for accurate clinical diagnoses. However, overly aggressive filtering can sometimes lead to the loss of critical data.
Amplification
Amplification is necessary to elevate the weak muscle signals captured by electrodes into a range that can be accurately measured and analyzed. In electromyography, low-amplitude signals need enhancement due to their subtlety.
- Key Characteristic: High gain settings allow for the detection of even slight muscular contractions, which is particularly important in conditions where muscle activity is minimal.
- Unique Feature: Adaptive amplifiers can automatically adjust to varying signal levels, ensuring consistent performance across different situations.
- Advantages/Disadvantages: Amplification increases data accuracy but too much gain can introduce additional noise and distort the signal.
Noise Reduction
Noise reduction techniques play a critical role in EMG interpretation. Muscle signals, even when processed correctly, can still be sullied by various sources of noise—be it from the environment, equipment, or even physiological processes.
- Key Characteristic: The use of techniques such as common-mode rejection allows EMG systems to differentiate between actual muscle signals and extraneous noises.
- Unique Feature: Noise cancellation algorithms can enhance the quality of data without compromising signal integrity, thus representing muscle activity more accurately.
- Advantages/Disadvantages: While noise reduction is instrumental in improving the clarity of results, there is always a potential risk of mistakenly filtering out significant signal components if not handled carefully.
Analysis and Interpretation of Results
Once data is gathered and processed, the next critical step is analysis and interpretation. This step helps translate raw data into useful clinical insights.
Quantitative Measurement
Quantitative measurement in EMG provides a numerical basis for understanding muscle activity. Measurements such as the amplitude and frequency of muscle contractions allow clinicians to objectively evaluate muscle function.
- Key Characteristic: This approach lends itself to statistical analysis, vital for identifying abnormalities and assessing treatment responses.
- Unique Feature: Standardized metrics such as Root Mean Square (RMS) values can be useful in comparing across different patient populations or assessing longitudinal changes in muscle function.
- Advantages/Disadvantages: While quantitative measurements offer compelling insights, they sometimes obscure complex patterns of muscle activity that might be better understood through qualitative means.
Qualitative Assessment
On the other hand, qualitative assessment provides a descriptive interpretation of muscle function, focusing on the patterns and variations in muscle activity. This approach can often reveal subtleties that numeric data might miss.
- Key Characteristic: Qualitative analysis can provide deeper insights into muscle coordination, fatigue patterns, and recruitment strategies, crucial for rehabilitation strategies.
- Unique Feature: Visual inspection of EMG waveforms can help clinicians grasp the functional status of a muscle more holistically.
- Advantages/Disadvantages: Qualitative assessments help in understanding individual patient scenarios but can be subjective and inconsistent without a rigorous framework.
In summary, methodologies in EMG encompass a wide range of techniques and procedures that form the backbone of muscle signal analysis. Accurate data collection, effective signal processing, and thorough analysis frame a comprehensive approach to understanding muscle function, paving the way for improved diagnoses and treatment options.
Collectively, these aspects create a robust framework that supports ongoing innovation and application in this critical area of health science.
Types of Electromyography
Electromyography encompasses various techniques that serve distinct purposes in both research and clinical settings. Understanding the types of electromyography is crucial, as it allows for tailored applications based on specific needs. The choice between these methods can significantly influence the quality of data collected and the insights derived from muscle activity. Here, we will discuss static and dynamic electromyography as well as multi-channel electromyography, highlighting their respective importance and unique benefits.
Static and Dynamic Electromyography
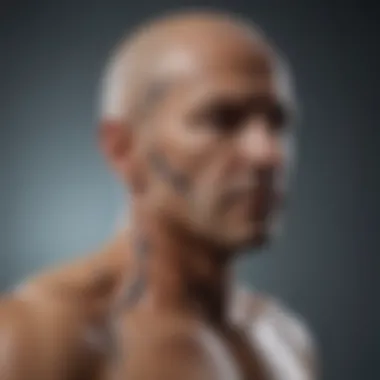
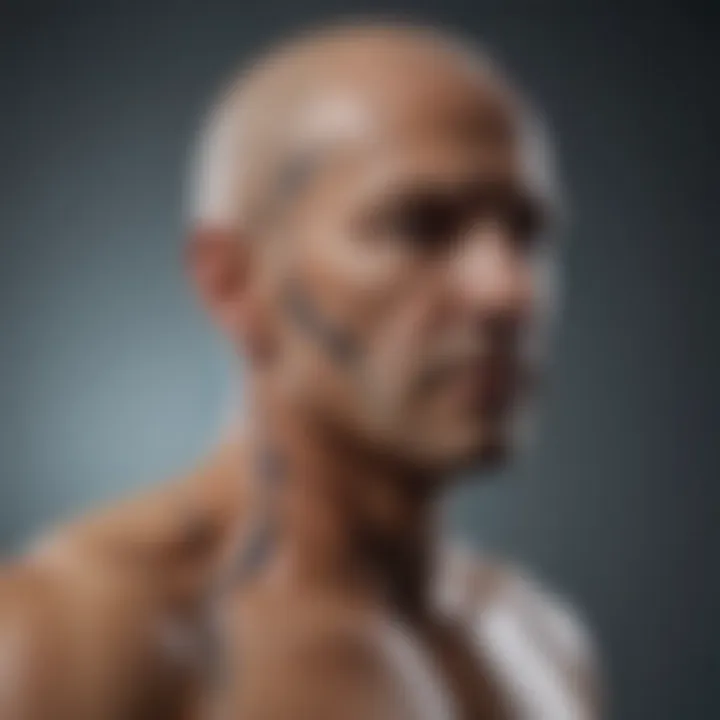
Static electromyography (sEMG) captures muscle electrical activity during isometric contractions—think of holding a weight without moving. It’s particularly useful in controlled clinical examinations. This method provides a wealth of information about the muscle’s ability to maintain force and stability. It's employed frequently in diagnosing conditions like myopathy, where muscle performance needs to be rigorously assessed.
On the flip side, dynamic electromyography (dEMG) shines a light on muscle activity during movement—imagine your muscles firing while you perform an exercise or run. This technique captures the changes in muscle activity in real-time, providing invaluable insights, especially in rehabilitation scenarios where understanding how muscles interact during functional activities can influence recovery strategies.
- Key Elements:
- sEMG is better for assessing muscle strength and endurance, while dEMG is ideal for analyzing movement patterns.
- Statics help identify neuromuscular deficiencies, while dynamics offer perspective on muscle coordination.
Multi-channel Electromyography
Multi-channel electromyography takes the capabilities of traditional methods a step further. By utilizing multiple electrodes placed at various sites on the skin or within the muscle, this technique allows for a more comprehensive assessment of muscle function. It captures simultaneous signals from different muscle regions, which is particularly useful in evaluating complex movements.
- Benefits of Multi-channel EMG:
- Enables spatial mapping of muscle recruitment and contraction.
- Offers a detailed view of muscle interplay during activities, valuable in sports science and rehabilitative practices.
- Assists in pinpointing dysfunctions and motor control issues that may not be visible in single-channel setups.
"The true potential of electromyography lies in its ability to adapt to various needs, yielding insights ranging from basic muscle function to complex movement coordination."
In summary, distinguishing between static, dynamic, and multi-channel electromyography adds layers to our understanding of muscular behavior. These techniques not only enhance diagnostic capabilities but also pave the way for advanced rehabilitation strategies and optimized athletic performance.
Applications of Electromyography
Electromyography (EMG) holds a significant place within the realm of health and performance. It bridges the gap between the scientific understanding of muscle function and real-world applications. The applications of EMG extend beyond just diagnostics, playing vital roles in clinical settings, rehabilitation, and even sports. Each application employs the key elements in EMG for various benefits, helping to enhance understanding and advancements in muscular health.
Clinical Applications
Diagnosis of Neuromuscular Disorders
The diagnosis of neuromuscular disorders is a pivotal use of electromyography. When we think about diagnosing conditions like amyotrophic lateral sclerosis (ALS) or muscular dystrophy, EMG stands out because of its ability to provide detailed insights into nerve and muscle health. By examining muscle electrical activity, clinicians can determine whether muscle weakness is due to a problem in the muscle or somewhere in the nervous system.
One key characteristic of using EMG in this context is its effectiveness in detecting abnormal electrical activities, which can be crucial for accurate diagnosis. This is a popular choice in medical practice because it offers clearer diagnostic insights compared to some other methods.
The unique feature of EMG's ability to provide real-time analysis allows for quick decision-making in clinical environments. While there are advantages like high specificity, some disadvantages do exist, such as discomfort during the procedure or the need for skilled personnel to conduct and interpret it well.
Surgical Planning and Monitoring
Surgical planning and monitoring rely heavily on electromyography. Surgeons often use EMG to visualize muscle and nerve functions when planning operations. Take the case of nerve repairs; EMG can determine which nerves are functioning well before the surgery takes place, allowing for better planning.
A fundamental aspect here is the insight EMG provides into the functional status of muscles prior to surgical intervention. This focus on functional assessment makes EMG invaluable in pre-operative evaluations. It’s a beneficial choice as it not only aids in planning but also helps in minimizing risks associated with surgical procedures.
The distinct capability of EMG to monitor muscular activity during surgery is another factor that sets it apart. However, the inherent limitations include the potential for false readings due to patient movement or interference from other electrical sources, making efficiency and precision paramount.
Rehabilitation Medicine
Muscle Rehabilitation Strategies
Within the field of rehabilitation medicine, EMG plays a crucial role in devising effective muscle rehabilitation strategies. Therapists utilize real-time EMG feedback to create personalized rehabilitation programs tailored to individual patient needs. This application is especially important for patients recovering from injuries or surgeries, as it directly influences recovery outcomes.
The emphasis in this context is on coordinated rehabilitation efforts, which is why EMG is a popular choice among practitioners. The ability to assess muscle activation patterns allows clinicians to modify rehab strategies dynamically based on the patient's progress. This personalized approach is a unique feature that stands out, promoting efficiency in recovery while also enhancing motivation for patients.
However, as beneficial as it is, one must consider potential limitations, such as the fact that some patients may find the technology intimidating or intrusive, potentially leading to reduced compliance with treatment plans.
Biofeedback Mechanisms
The concept of biofeedback mechanisms is yet another prominent application of EMG in rehabilitation. By providing real-time muscle activity feedback, EMG assists patients in understanding how they control their muscles during exercises. This understanding can be transformative, motivating patients to engage more thoroughly in their rehabilitation.
A significant characteristic of biofeedback is that it empowers patients by making them aware of their own muscle function. This effectiveness is what makes it a popular choice in modern therapy settings. In contrast to traditional methods, where the feedback may be less immediate, EMG-driven biofeedback offers an interactive experience that informs patients continuously about their performance.
Nevertheless, certain drawbacks exist, such as the need for the technology to be adequately integrated into therapy sessions, requiring training and resources that may not be universally available.
Sports Science and Performance Analysis
Muscle Fatigue Assessment
In the domain of sports science, EMG serves a crucial purpose in muscle fatigue assessment. Athletes rely on data gathered from EMG to understand how fatigue affects muscle performance over time. When measuring electrical activity in muscles during exertion, coaches can glean important insights into an athlete's endurance levels, potentially informing training regimens.
The focus here is on identifying fatigue patterns, which can be key for performance optimization. This makes EMG a valuable tool for coaches and athletes alike. One unique feature of EMG in this context is its ability to provide quantitative data that can track muscle fatigue not observable through traditional training techniques, allowing for a more scientific approach to training.
While effective, some might argue that EMG requires a thorough understanding of how to interpret the data correctly, presenting a challenge for those without advanced training in sports science.
Technique Optimization
Finally, we arrive at technique optimization in sports, where EMG shines by analyzing muscle activation during various athletic techniques. By utilizing EMG data, athletes can refine their movements, ensuring that they are not just training harder, but also training smarter.
The process of technique optimization relies heavily on data-driven insights, meaning that performance improvement can be quantitatively measured. This characteristic makes it a popular choice among trainers looking to enhance their athletes’ capabilities. The dynamic assessment capabilities of EMG during training sessions provide unique insights that directly influence performance.
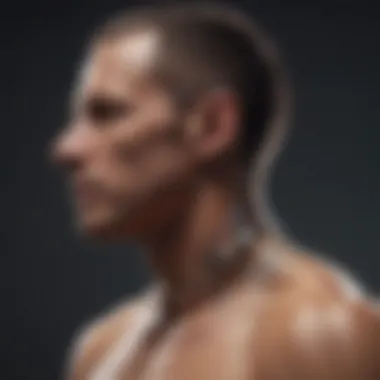
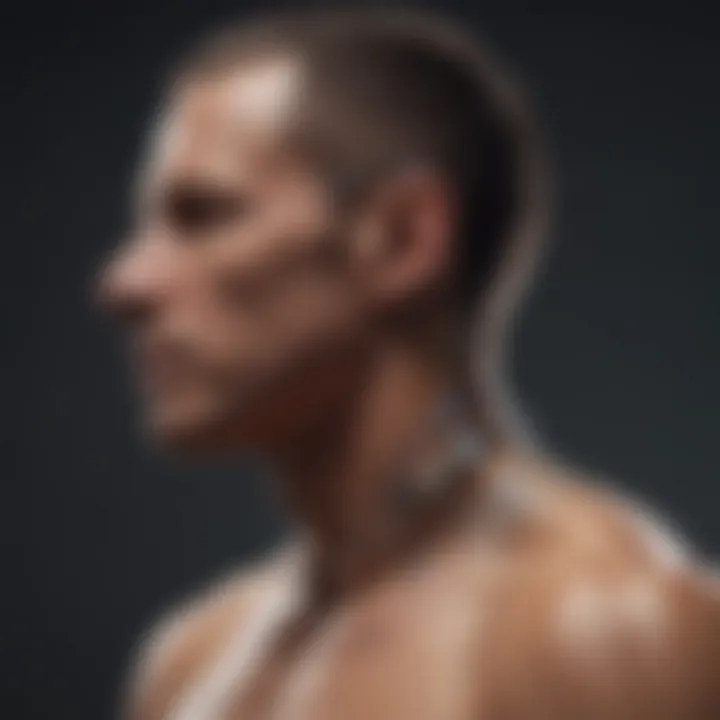
However, one should also consider challenges such as equipment cost and the sporadic need for trained technicians, in order to implement EMG successfully across various training environments.
"The applications of electromyography extend beyond just diagnostics— it influences rehabilitation, sports performance, and surgical interventions. Each application zone provides unique insights that help refine methodologies in muscle health management."
In summary, the applications of electromyography within clinical, rehabilitative, and sports contexts illustrate its versatility and profound impact on muscle health. Each area utilizes the intricate data EMG provides to foster improvements and knowledge development, helping patients and athletes optimize their functioning effectively.
Advancements in Electromyography Technology
The evolution of electromyography technology has dramatically enhanced the ability to monitor and interpret muscle activity. This section explores pivotal advancements made in the field, focusing on specific elements like wearable technologies and the integration of artificial intelligence, each playing a crucial role in refining how electromyography is applied in various settings. As technology progresses, these advancements not only improve data accuracy but also open doors to innovative applications and transformative healthcare solutions.
Wearable Technologies in Electromyography
Wearable technologies represent a revolutionary stride in electromyography, shifting the paradigm from traditional lab-based equipment to convenient, user-friendly devices. This advancement allows for continuous monitoring of muscle activity in naturalistic settings, rather than restricted environments, which adds significance to the data collected.
- Features of Wearable Devices: These devices often include lightweight sensors that can be attached directly to the skin, using surface electromyography to capture electrical signals from muscles effectively. This permits real-time feedback on muscle performance, especially valuable for athletes and rehabilitation patients.
- Benefits: The immediate benefits of wearables include improved accessibility for users and enhanced engagement in personal health monitoring. They empower users to participate actively in their recovery or performance enhancement journey, often leading to better adherence to therapeutic protocols.
While these technologies come with a price tag, the long-term benefits in terms of personalized data-driven insights can outweigh initial costs, making them a popular choice among clinicians and researchers.
Integration with Artificial Intelligence
The integration of artificial intelligence (AI) has introduced a layer of sophistication in electromyography that was previously unimaginable. It elevates the process of data analysis, allowing for faster and more accurate interpretation of muscle signals, which is essential for both diagnostics and research.
Machine Learning Applications
Machine learning applications are at the forefront of this integration, offering significant contributions to the overall understanding of muscle dynamics. By leveraging vast amounts of data, machine learning algorithms can identify patterns that may not be apparent to the human eye.
- Key Characteristic: One of the standout features of machine learning in this context is its ability to adapt and improve over time. The more data the system collects, the more accurate its predictions and assessments become.
- Benefits: This adaptability allows for more personalized treatment plans, tailored specifically to individual muscle function.
- Unique Feature: Another advantage is the capability for automated interpretation of results, reducing the workload for clinicians and minimizing human error. However, it’s important to note that reliance on AI can raise concerns about data privacy and the need for ethical oversight in its application.
Predictive Analytics
Predictive analytics, a specific facet of AI integration, has gained traction in helping clinicians foresee potential neuromuscular issues before they escalate. By analyzing historical data and identifying trends, predictive analytics offers a proactive approach to patient care.
- Key Characteristic: The predictive capabilities provide forecasts on muscle performance based on previous activity, which can guide future training or rehabilitation strategies.
- Benefits: This foresight aids both athletes and patients in modifying regimes to prevent injuries or exacerbation of conditions.
- Unique Feature: However, the limitations do exist, especially when dealing with variability in individual responses to training or treatment. The reliance on historical data can sometimes misrepresent future outcomes if proper context isn't considered.
"The marriage of wearable tech and artificial intelligence paves a new way for patient engagement, turning the user into an active participant in their health journey."
Future Directions and Challenges
The realm of electromyography (EMG) is continually evolving, influenced by technological innovations and expanding research domains. As we stand on the cusp of novel methodologies and applications in EMG, understanding the future directions and challenges becomes crucial. This exploration is not merely an academic exercise but a necessary dialogue among students, researchers, educators, and professionals to foster advancements in muscle function analysis and neuromuscular disorder diagnosis.
Emerging technologies, particularly in the wearable sector, lead the charge in reshaping how EMG data is collected and interpreted. For instance, the advent of skin-embedded sensors that use nanotechnology has the potential to monitor muscle activity in real time. With devices becoming more compact and accurate, the accuracy of muscle signal analysis improves, which can lead to better patient outcomes.
However, with these advancements come several challenges that demand attention. One significant hurdle is integrating such technologies into traditional healthcare practices. Healthcare professionals often face resistance to new tools, primarily due to a lack of training or understanding. Thus, fostering educational programs becomes critical to bridging this gap.
Emerging Research Areas
As research in EMG expands, specific areas have begun to garner emphasis:
- Neurotechnological Interfaces: Innovations here promise not only controlled muscle function but a deeper understanding of neuro-motor functions. The integration of neuroengineering and EMG can lead to groundbreaking rehabilitation protocols, especially for patients suffering from paralysis.
- Machine Learning Algorithms: The use of artificial intelligence in interpreting EMG signals is burgeoning. Machine learning can help identify patterns in muscle activation, which may assist in early diagnosis of neuromuscular disorders. The more the data, the clearer the diagnosis.
- Virtual Reality Integration: As virtual environments become more sophisticated, combining them with EMG analysis offers new avenues for rehabilitation. Just imagine a patient undergoing muscle training through simulations that react in real-time to their muscle activity.
Potentially, these research areas can redefine how we view muscle rehabilitation and monitoring, presenting a mix of opportunities for improved patient care and understanding of muscular mechanics.
Ethical Considerations in Electromyography Research
Diving into the ethical landscape of EMG research, it’s essential we consider several aspects, which directly impact research integrity and public trust:
- Data Privacy: With the increasing data collection from wearables, maintaining user privacy is paramount. Researchers must establish robust protocols to protect sensitive information garnered from individuals.
- Informed Consent: Participants in EMG studies should be made fully aware of how their data will be used, including potential benefits and risks. Clear communication fosters trust and ensures ethical standards are upheld.
- Access and Equity: As EMG technologies advance, we risk widening the gap between those who have access to high-quality medical devices and those who don’t. This disparity necessitates proactive measures to ensure equitable access to cutting-edge therapies.
As the EMG landscape matures, it presents not only technological hurdles but also profound ethical dilemmas. Addressing these challenges head-on is essential for the sustainable growth of electromyography in research and clinical practice.
"The future of EMG is as much about technology as it is about ethical stewardship and equity in healthcare."
Culmination
In wrapping up this exploration of electromyography, it’s crucial to underline the essence of this technique in both clinical practice and research domains. Electromyography doesn't just allow us to peek into the workings of muscle activity; it acts as a compass guiding medical professionals in diagnosing various neuromuscular disorders. By accurately capturing electrical signals generated by muscles, electromyography serves as a pivotal tool in understanding muscular dysfunction. It's like being handed the keys to a hidden world where signals translate to tangible movements and coordinated activities—without these insights, clinicians might often be left in the dark when unraveling complex cases.
Summation of Key Insights
Throughout this article, we’ve navigated various facets of electromyography. The fundamental principles lay the groundwork for grasping how these electrical signals convey vital information about muscle health. We explored various methodologies used to collect and analyze data, where we noted that techniques like surface and needle electromyography cater to different clinical needs. The advancements in technology, especially in wearable devices and AI, promise a future where monitoring and analysis become less cumbersome and more integrated into daily care.
A few key insights include:
- Diversity of Applications: Electromyography finds applications in clinical settings to diagnose conditions, in rehabilitation strategies, and even within the realm of sports science for enhancing performance.
- Significance of Data: The ability to quantify and interpret muscle activities yields important information not just for diagnosing conditions but also for assessing treatment effectiveness over time.
- Technological Integration: The fusion of electromyography with cutting-edge technologies such as machine learning expands its capabilities, opening doors for predictive analytics in patient care.
In essence, electromyography is not just a standalone technique. It’s a bridge connecting our understanding of neuromuscular health with practical applications in therapy and rehabilitation.
Call for Continued Research and Innovation
The scope of electromyography continues to evolve, yet there are still galaxies of knowledge left to explore. Calls for continued research and innovation are imperative as we venture deeper into this interdisciplinary domain. Understanding the nuances of muscle signaling even further could lead to breakthroughs in treatment approaches for chronic spinal cord injuries or degenerative nerve conditions.
- Promoting Interdisciplinary Collaboration: Encouraging partnerships between neuroscientists, engineers, and clinicians could foster a fresh wave of research, leading to novel applications of electromyography.
- Addressing Ethical Implications: With the rise of wearable technologies and AI integration in electromyography, it’s crucial to navigate ethical considerations around data privacy and the ramifications of automation in diagnostics.
- Funding and Support for Innovation: More financial backing for research initiatives will facilitate projects aimed at pushing the boundaries of how we use electromyography in various fields.
As we stand on the precipice of these innovations, the future of electromyography looks promising. It beckons researchers and practitioners alike to delve deeper, ensuring that we unlock the full potential of this indispensable tool in our pursuit of enhanced human health and performance.