Exploring Cell Viability Measurement Techniques
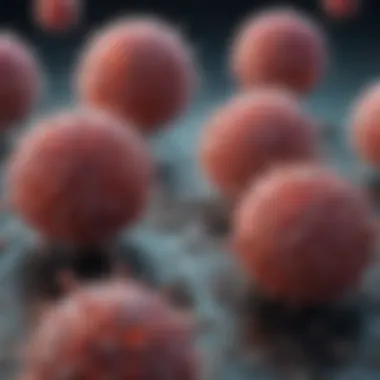
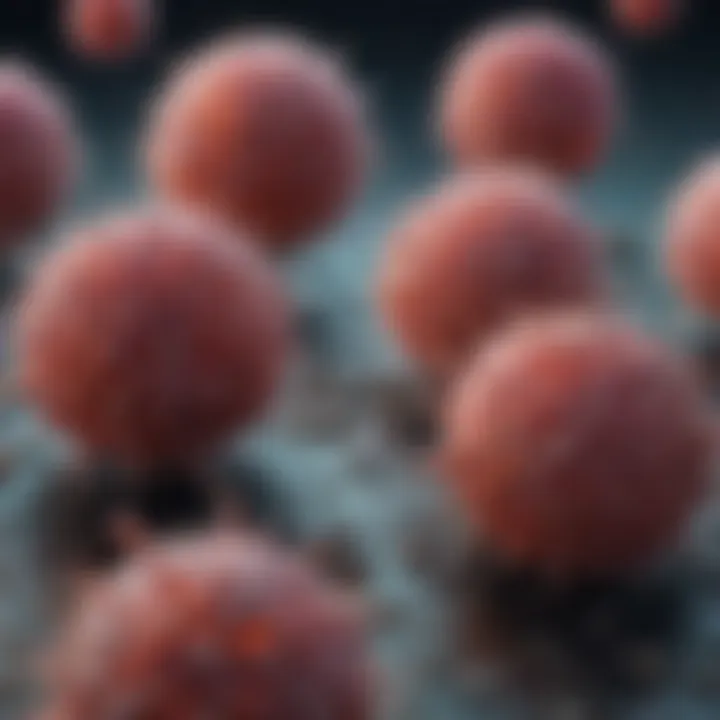
Intro
Cell viability is a critical parameter in biological research and medical applications. This concept serves as an indicator of cellular health, providing insight into the state of cells in various conditions. Accurate measurements of cell viability are essential for understanding drug efficacy, toxicological impacts, and fundamental biological processes. Researchers increasingly rely on different methodologies for assessing cell viability, each with distinct characteristics that can influence the outcomes of their studies.
Addressing the nuances in cell viability assessment is of utmost importance, especially within the context of drug development and toxicology. As scientific inquiry progresses, the demand for reliable, precise methods becomes more pronounced. This article will elucidate the methodologies employed in measuring cell viability, encompassing both traditional techniques and innovative approaches.
Research Overview
Research in the field of cell viability is expansive and multifaceted. Various studies contribute to a more profound understanding of how different conditions affect cellular health.
Summary of Key Findings
Recent findings in cell viability research underscore the need for a thorough evaluation of measurement techniques. Key insights include:
- Diversity of Methods: Different assays, such as the MTT assay, Trypan Blue exclusion, and flow cytometry, each offer unique advantages and drawbacks.
- Influence of Environmental Factors: Factors like pH, temperature, and incubation time can significantly impact assay outcomes, making standardization critical.
- Reproducibility Concerns: Variability in methodology can lead to inconsistent results, emphasizing the need for rigorous protocol development.
Research Objectives and Hypotheses
The objectives of this exploration include:
- To examine the range of methodologies used for measuring cell viability.
- To compare the effectiveness and reliability of each method.
- To highlight the implications of cell viability measurements in drug discovery and toxicology.
Hypotheses underpinning this research suggest that methodologies exist with varying levels of sensitivity, specificity, and applicability, which can lead to differing constraints and applications in real-world scenarios.
Methodology
In order to present a comprehensive discourse on measuring cell viability, it is vital to outline the research approach and data collection techniques utilized in this field.
Study Design and Approach
The study design is primarily observational, relying on comparative analyses of existing methodologies. Literature reviews of published research, alongside key laboratory protocols, provide an evidence base for critical evaluation.
Data Collection Techniques
Data is gathered through reviewing experimental protocols, published assay results, and available datasets. Metrics such as cell survival rates, cytotoxicity levels, and threshold values for assay sensitivity are analyzed to provide a clear picture of the methodologies in question. By organizing this information systematically, the article intends to guide readers through the complexities of measuring cell viability effectively.
"Understanding the methodologies behind measuring cell viability ensures that researchers can choose the right tools for their specific needs."
Intro to Cell Viability
Cell viability is a critical parameter in biological and biomedical research. It pertains to the health status of cells in various conditions and environments, and understanding this concept is essential for several reasons. This article aims to provide a comprehensive overview of cell viability, emphasizing the techniques available for measurement, the relevance of these measurements, and their implications across different fields of study.
The significance of measuring cell viability lies in its broad application in research. Viability assays are pivotal in assessing the effectiveness of drugs, understanding cell responses to stimuli, and studying disease mechanisms. Researchers frequently evaluate cell viability to determine a drug's potential toxicity or efficacy before progressing to more complex in vivo studies. By accurately gauging which cells are alive or dead, researchers can draw valid conclusions about cellular functions, paving the way for advancements in therapeutic approaches.
Moreover, cell viability measurements play a crucial role in stem cell research, where understanding the differentiation and survival of cells is paramount. It also is vital in toxicology studies, where assessing how substances affect the health of cells helps in evaluating risks associated with exposure to chemicals or pollutants.
Several factors must be considered when interpreting cell viability assays, including the specific context of the study and the nature of the cell type involved. Different cell types can exhibit varied responses to stress, requiring tailored approaches for evaluation. The development and selection of appropriate assays, therefore, become imperative in yielding reliable results.
In summary, the introduction to cell viability sets the stage for a deeper understanding of its importance within scientific research. The correct interpretation and application of viability measurements can significantly influence not only experimental outcomes but also the future direction of research in various disciplines.
Understanding cell viability is foundational, guiding decisions that impact research integrity and innovation, particularly in fields like drug development and toxicology.
The Importance of Cell Viability Measurements
Cell viability measurements are crucial in various scientific disciplines. They provide essential insights into the health and functionality of cells under different conditions. Assessing cell viability informs researchers about how cells respond to drugs, environmental stressors, or genetic modifications. Thus, accurate measurements are not just beneficial; they are necessary for the integrity of many scientific conclusions and advancements.
Applications in Biomedical Research
In biomedical research, understanding cell viability is fundamental. Studies involving cancer research, regenerative medicine, and immunology heavily rely on these measurements to draw accurate conclusions. For example, researchers commonly analyze the effects of novel compounds on cancer cell lines. By determining whether the tested drugs induce cell death or inhibit proliferation, scientists can identify potential therapies. In this context, cell viability data directly influences the subsequent steps of clinical trials, marking its importance in transitioning from bench to bedside.
Role in Drug Development
The role of cell viability measurements in drug development cannot be overstated. Companies in the pharmaceutical industry routinely utilize viability assays to screen new drugs. Here, the primary objective is to measure how effective a compound is in eliminating unhealthy cells, like cancer cells, while sparing healthy ones. These evaluations are a part of preclinical testing to optimize drug efficacy. Moreover, cell viability assessments can also help identify the toxicity levels of new substances, leading to safer therapeutic options. To maintain regulatory compliance, thorough documentation of these measurements is often required.
Impact on Toxicology Studies
Toxicology studies investigate how substances may harm living organisms. Within this framework, measuring cell viability is crucial. It determines the cytotoxic effects of various chemicals, including industrial pollutants or consumer products. Understanding cell death mechanisms enables scientists to establish safety protocols and regulatory guidelines. Furthermore, assessments of cell viability guide researchers in categorizing substances by their harmful potential. This has implications for public health, leading to informative risk assessments and safety regulations.
Relevance in Stem Cell Research
Stem cell research presents unique challenges and opportunities, where measuring cell viability serves a different purpose. Ensuring viability is critical for the success of stem cell therapies. Scientists must monitor how stem cells differentiate and how well they survive post-transplantation. Viability measurements can reveal how well stem cells are integrated into host tissues, affecting the outcome of regenerative approaches. This understanding is essential for advancing treatments in areas like organ replacement or tissue engineering.
"Cell viability measurements guide discovery and innovation across various scientific fields. Understanding these metrics aids in significant applications that can shape future therapies and public health policies."
Conceptual Framework of Cell Viability
Understanding the conceptual framework of cell viability is vital for a thorough grasp of the methodologies discussed in this article. Cell viability refers to the ability of cells to maintain homeostasis and function properly under specific conditions. This framework is not only foundational for life sciences but also critical in fields such as drug development and toxicology. Each methodology for measuring cell viability serves distinct purposes, often dictated by the specific context of the study.
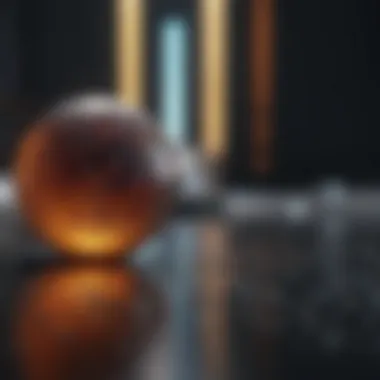
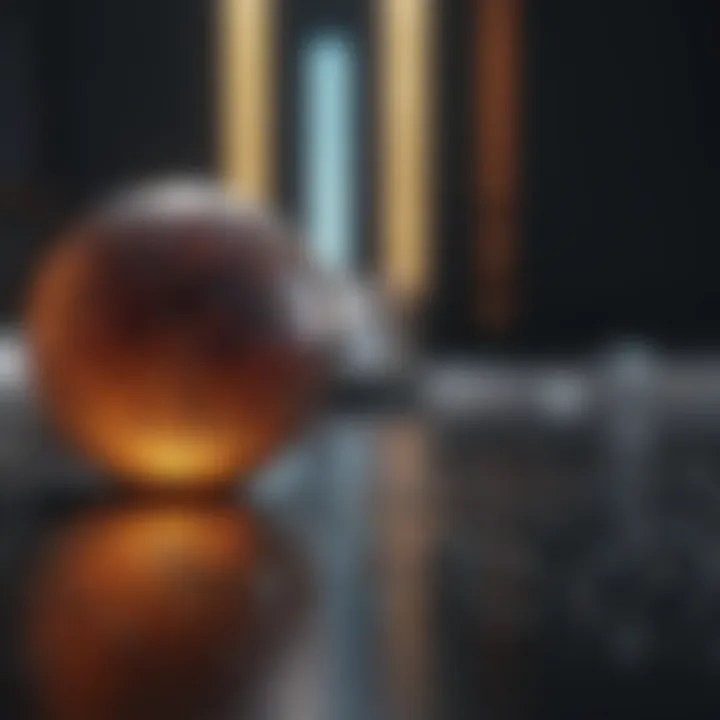
Defining Cell Viability
Cell viability encompasses the ability of cells to survive and function within a given environment. This definition extends to both physiological and pathological conditions. To determine cell viability, one typically assesses the cellβs metabolic activity, membrane integrity, and ability to proliferate. Researchers often refer to viable cells as those that can perform essential functions, including growth and replication. It is crucial to recognize that the parameters for defining cell viability may differ depending on the experimental context. For instance, in some research scenarios, specifically in apoptosis studies, even cells that have completed programmed cell death may be counted as viable if they exhibit certain metabolic activities in initial assays.
Difference Between Viability and Proliferation
While cell viability indicates whether cells are alive, proliferation refers to the complex process of cell division and growth. It is important to distinguish the two, as a cell can be viable but not proliferative. This distinction has practical implications in research.
- Viability assesses the health of cells, focusing on their functional capacity.
- Proliferation, on the other hand, evaluates how a population of cells increases over time.
In many cases, drugs targeting specific pathways might affect cell viability without influencing proliferation rates, necessitating dual assessments. Understanding this difference enables researchers to interpret results accurately, allowing for more informed decisions in experimental design and therapeutic applications.
In summary, the conceptual framework solidifies the understanding of cell viability and proliferation as crucial markers in biological research. This comprehension lays the groundwork for utilizing various assays and techniques effectively.
Common Techniques for Measuring Cell Viability
Understanding the techniques for measuring cell viability is vital in various fields, especially in biological research. Each method offers unique insights into cell health, function, and overall biological activity. Selecting the right technique is crucial, as it can directly impact experimental outcomes and interpretations.
In this section, we will discuss several common techniques, focusing on their mechanisms, advantages, and limitations. The choice of assay often depends on specific research needs, the type of cells being studied, and the resources available.
MTT Assay
Mechanism
The MTT assay measures cell viability based on metabolic activity. Viable cells convert the yellow MTT reagent into purple formazan crystals. This process is seen as an indicator of cellular health. A significant characteristic of this technique is its simplicity and ability to provide quantitative results rapidly. In many laboratories, MTT is favored for its reliability in detecting viable cells.
Advantages
One key advantage of the MTT assay is its quantitative nature. It allows researchers to discern the degree of cell viability efficiently. Additionally, the method is cost-effective and easy to perform. The fast results facilitate timely decision-making processes in experimental procedures, making it a popular choice in many studies.
Limitations
Despite its strengths, the MTT assay has limitations. It does not distinguish between live and dead cells effectively in certain conditions. Furthermore, this method requires additional steps to dissolve the formazan crystals, which can introduce variability in results. As such, relying solely on MTT may not always provide the complete picture needed for precise analyses.
Trypan Blue Exclusion
Mechanism
The Trypan Blue exclusion method utilizes the principle that viable cells exclude the dye, whereas non-viable cells absorb it. This straightforward method allows researchers to count both living and dead cells under a microscope. The practicality of this technique makes it a commonly used choice in many laboratories.
Advantages
Its principal advantage is direct observation of cell viability, allowing researchers to assess cell condition visually. The method is also simple and quick, providing results that can be obtained in real-time. This aspect is particularly beneficial when immediate assessments of cell health are necessary.
Limitations
However, the Trypan Blue exclusion method is not without drawbacks. It requires manual counting, which can be prone to human error and may lead to inconsistencies. Additionally, it does not quantify the degree of cell viability; it only records the number of living versus dead cells, potentially oversimplifying the situation.
Calcein AM Assay
Mechanism
The Calcein AM assay relies on the ability of live cells to retain the non-fluorescent Calcein AM dye. Once inside the cell, the dye is converted into fluorescent calcein by intracellular esterases. This change is exclusively observable in live cells, thus indicating viability. This method is popular for its simplicity and clear indication of cell health.
Advantages
One significant advantage is that it provides quantitative data with the ability to visualize using fluorescence microscopy or flow cytometry. Also, Calcein AM is versatile, applicable in various cell types and conditions. This flexibility makes it a favored tool in many experimental settings.
Limitations
Nonetheless, the Calcein AM assay is not free from limitations. The presence of high concentrations of cell debris can interfere with fluorescence readings, leading to skewed results. Moreover, the method does not differentiate between apoptotic and necrotic cells, which may be relevant in specific research areas.
Annexin /PI Staining
Mechanism
The Annexin V/PI staining method utilizes two dyes to assess different phases of cell death. Annexin V binds to phosphatidylserine, which is exposed on the outer membrane of early apoptotic cells. Propidium iodide (PI), on the other hand, penetrates only necrotic cells. This allows for the differentiation between viable, apoptotic, and necrotic cells, providing a more nuanced analysis.
Advantages
A key strength of this method is its ability to categorize cells effectively. This is particularly useful in studies investigating apoptotic processes. The technique also allows for quantitative assessments when combined with flow cytometry, increasing reliability in data interpretation.
Limitations
Yet, the Annexin V/PI method has some restrictions. The requirement for specific equipment such as flow cytometers can limit access in some laboratories. Furthermore, the staining procedure is time-sensitive; delays can lead to false-positive results if cells deteriorate before analysis.
Lactate Dehydrogenase (LDH) Release Assay
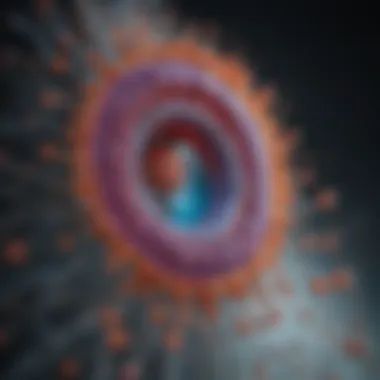
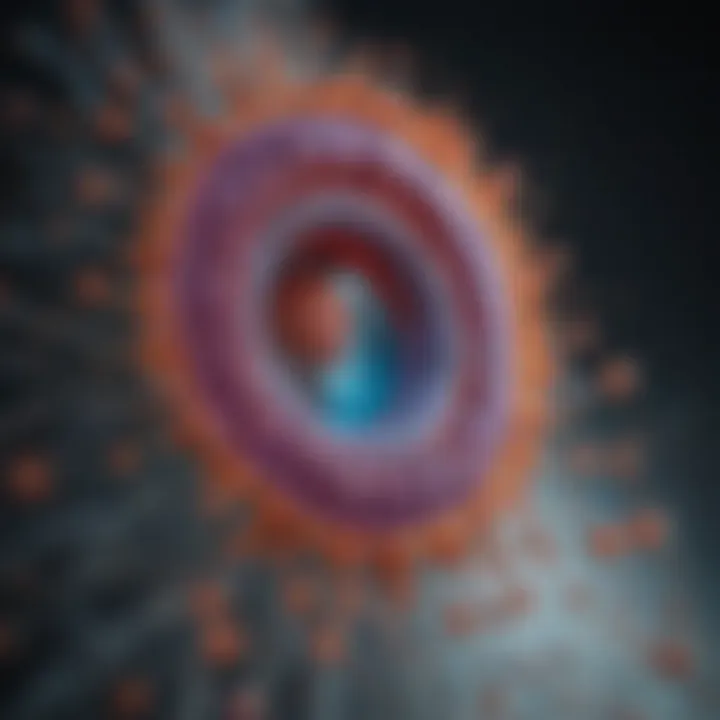
Mechanism
The LDH release assay measures cell damage based on the enzymatic release of lactate dehydrogenase from damaged cells into the surrounding medium. This offers a straightforward way to quantify cellular damage using a colorimetric or fluorometric approach. The ability to measure LDH levels provides immediate insights into cell viability.
Advantages
This method's main advantage is its ability to assess cell membrane integrity and damage effectively. The LDH assay can quantify cell death without requiring direct counting or observations. This aspect enhances its usability in high-throughput settings where large sample sizes are common.
Limitations
However, the LDH release assay is not entirely without issues. It does not specify the cause of cell death, limiting insights into the underlying mechanisms at play. Additionally, some cellular processes can release LDH independently from cell death, complicating interpretation without correlation to viability assessments.
"Choosing the right cell viability assay requires an understanding of the specific biological questions being investigated and the context of the study."
Each of the techniques discussed plays a crucial role in cell viability assessment, yet their application must be carefully considered in light of their respective strengths and weaknesses to ensure accurate interpretation of research results.
Emerging Techniques in Cell Viability Measurement
The evolving landscape of cell viability measurement has introduced new methodologies that enhance precision and offer unique insights. Emerging techniques are crucial because they provide alternative approaches to traditional assays, addressing limitations in sensitivity, accuracy, and throughput. Understanding these innovative methods is fundamental for researchers aiming to optimize their experiments and acquire reliable data.
Flow Cytometry
Overview
Flow cytometry is a powerful analytical technique that allows for the simultaneous measurement of multiple physical characteristics of cells. It uses lasers to illuminate cells as they flow in a stream through a focused beam of light. This method is popular because it provides rapid quantification of cell populations and can analyze thousands of cells per second. An advantage is its ability to assess several markers and parameters at once, making it an efficient tool for cell viability assessments. However, flow cytometry requires specialized equipment and expertise, which could be a barrier for some laboratories.
Applications
In the context of cell viability measurement, flow cytometry offers numerous applications. It is particularly beneficial in analyzing apoptosis, necrosis, and overall cell health in various experimental conditions. This technique can distinguish between live, dead, and apoptotic cells, which is essential for understanding cellular responses to drugs or environmental stressors. Its unique capacity for high-throughput analysis allows researchers to process large sample sizes quickly, thus facilitating extensive statistical analysis. However, the cost of flow cytometry equipment and maintenance can be substantial.
Imaging Techniques
Overview
Imaging techniques, such as live-cell imaging and high-content screening, have become significant in assessing cell viability. These methods utilize microscopy to observe cells over time, providing visual data that enhance understanding of cellular behavior. The key characteristic of imaging techniques is that they allow for direct visualization of live cells, offering real-time insights. This attribute makes them beneficial for studying dynamic biological processes. Yet, challenges include potential phototoxicity and the complexity of image analysis, which may require advanced software and expertise.
Applications
In research, imaging techniques can evaluate the impact of drug treatments or environmental factors on cell viability. They facilitate the observation of morphological changes and cellular interactions, which are not always quantifiable by traditional assays. This methodology's unique feature lies in its ability to provide spatial and temporal information about cells. However, the resolution and clarity of images can be affected by factors such as fluorescent dye concentration and the imaging environment, which needs careful optimization.
Microfluidics
Overview
Microfluidics refers to the manipulation of tiny volumes of fluids, usually in the microliter range, to study biological processes and analyze cells. This approach allows for precise control over experimental conditions and enables the integration of multiple assays on a single platform. The key benefit of microfluidics is its ability to perform high-throughput analyses with minimal sample volumes, making it a cost-effective choice in cell viability studies. Nevertheless, the technology can be complex to develop and may require substantial initial investment.
Applications
Microfluidic devices find applications in drug discovery, toxicity assessment, and analysis of single-cell behaviors. These devices can conduct real-time viability assays, rapidly screening multiple drug candidates under controlled conditions. A unique feature of microfluidics is that it allows for the combination of assays, enabling simultaneous analysis of cell viability, proliferation, and response to stimuli. However, the integration of microfluidics into existing laboratory workflows can pose challenges, particularly for researchers unfamiliar with this technology.
Comparison of Cell Viability Assays
The comparison of cell viability assays provides critical insight into how different methodologies can impact research outcomes. By scrutinizing these assays, one can determine which method is most suitable for specific experiments and how varying techniques can influence the interpretation of results. This section highlights essential elements in assessing the strengths and weaknesses of prevalent techniques used in measuring cell viability, thereby guiding researchers in choosing the right approach.
Scenarios for Use
Different research scenarios demand specific cell viability assays. It is important to select the best fitting method based on the context of the study.
- Cell Type: Some assays work better with certain cell types. For instance, adherent cells may require different consideration than suspended cells.
- Experimental Design: The complexity and design of an experiment can dictate the choice of assay. For high-throughput screening, methods like the MTT assay may be preferred for their speed and cost-effectiveness.
- Sensitivity Requirements: In studies where subtle distinctions in viability need to be measured, more sensitive methods, such as flow cytometry, may be required.
Selecting the right assay helps in accurately reflecting the biological state in various conditions and treatments.
Cost vs. Accuracy
Cost considerations often play a vital role in experiment planning. However, researchers must balance cost efficiency with accuracy and reliability of the results. Some key points to consider include:
- Budget Constraints: Lower-cost assays like Trypan Blue exclusion provide quick results but may not offer the accuracy needed for more complex applications.
- Investment in Technology: Advanced techniques, such as flow cytometry, may entail higher upfront costs but provide precise data that can justify their expense during long-term studies.
- Quality of Data: Investing in accurate assays ensures that the conclusions drawn are valid. This is critical in fields necessitating high fidelity, like drug development and toxicology.
Ultimately, a comprehensive understanding of how costs correlate with accuracy can inform more strategic experimental decisions.
Throughput Considerations
Throughput needs can greatly influence the choice of viability assays. In a research context where multiple samples are processed simultaneously:
- High-Throughput Screening: Techniques that allow for simultaneous analysis of many samples, such as the Calcein AM assay, are essential for large-scale studies. These assays streamline data collection without compromising on quality.
- Sample Size Implications: In experiments requiring large sample sizes, methods that are straightforward and quick to execute are preferred. The efficiency of assays can lead to better resource management and time savings.
- Automation Opportunities: Some assays are readily adaptable to automation, which can further enhance throughput and consistency of results.
By aligning the choice of assay with throughput demands, researchers can optimize both data collection and analysis.
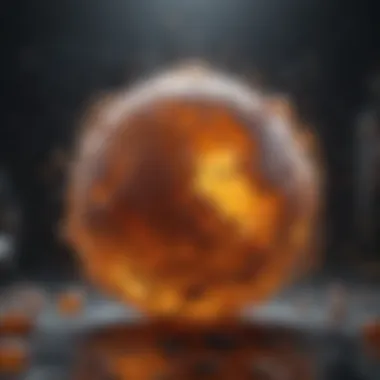
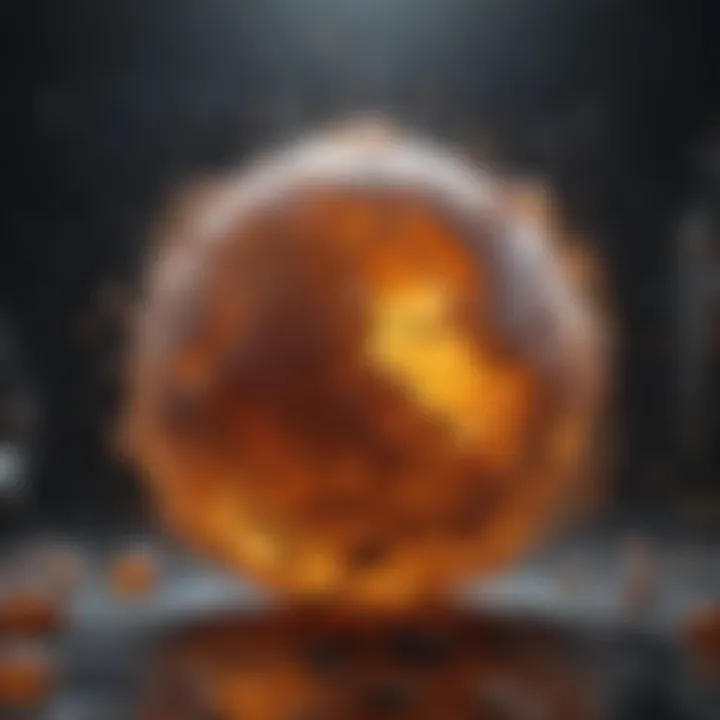
"Choosing the right cell viability assay is pivotal to ensure that the results of biological experiments are valid and reflective of physiological conditions."
Interpreting Results: Relevance and Limitations
Understanding the interpretation of cell viability results is vital in crafting accurate conclusions about experimental findings. The data obtained from various assays should guide future research and clinical applications. Without a proper understanding of the relevance and limitations of these results, misinterpretations can lead to flawed hypotheses and potentially detrimental outcomes in biomedical applications. Each measurement technique has its own set of advantages and drawbacks that must be considered when evaluating data.
Understanding Data Variability
Data variability is an inherent part of scientific measurements. Cell viability assays are no exception. Differences in sample handling, assay conditions, and even the biological characteristics of the cells can lead to variations in results. High variability in data can obscure meaningful trends and result in misunderstandings of cell behavior. To tackle this, researchers should always consider factors that might introduce variability.
- Repetitive Testing: Performing assays in triplicate or more can provide more reliable data. This approach reduces the impact of outlier results and helps in establishing a more consistent dataset.
- Standard Operating Procedures: Implementing thorough guidelines for conducting assays can minimize variability. For example, using the same cell line, culture conditions, and reagent lots will ensure more reliable comparisons across experiments.
- Statistical Analysis: Employing proper statistical analyses can help quantify variability and assess the significance of results. Understanding the standard deviation and confidence intervals offers a clearer picture of data reliability.
Biological Context Matters
When analyzing data from cell viability assays, it is important to contextualize the results within the biological framework of the experiment. Cell viability is just one aspect of cellular behavior. Understanding how specific drugs, treatments, or environments affect cellular health is crucial. A few key points to consider include:
- Cell Type Specificity: Different cell types may respond differently to certain treatments. Factors such as maturation stage, genetic background, and environmental adaptations can influence viability outcomes. Hence, results from one cell type should not be blindly extrapolated to another.
- Microenvironment Influence: The surrounding environment of the cells plays a role in their viability. Conditions such as nutrient availability, cell density, and the presence of growth factors can significantly alter how cells respond to treatments.
- Long-term vs. Short-term Effects: It is essential to differentiate between instant cell death and more delayed responses. While immediate results may seem promising, the impact of treatments may manifest over time in a different way.
"The interpretation of data should always be seen within the larger biological narrative, not in isolation. This approach fosters better understanding and innovative research outcomes."
Regulatory Perspectives on Cell Viability Testing
In the field of biological research and pharmaceutical development, understanding regulatory perspectives on cell viability testing is of paramount importance. Regulatory agencies, such as the Food and Drug Administration (FDA) and the European Medicines Agency (EMA), play a crucial role in ensuring that cell viability tests are reliable, reproducible, and relevant to human health.
Importance of Regulatory Guidelines
Regulatory guidelines provide a framework to ensure that scientific research adheres to high standards of integrity and ethical considerations. They establish the norm for methodologies that are accepted within the scientific community. These guidelines emphasize the significance of:
- Consistency: Standard procedures for cell viability assays enable comparison of data across different studies and laboratories.
- Reproducibility: Ensuring that experiments can be repeated with the same outcomes is essential for validation.
- Safety: Cell viability assays help in determining the impact of drugs and chemicals, ensuring safety for potential human use and minimizing harmful side effects.
Specific Elements of Regulatory Considerations
Within the context of cell viability testing, several specific elements warrant attention. These include:
- Method Selection: Regulatory bodies provide recommendations on which assays are most suitable for specific applications, such as the MTT assay or Annexin V/PI staining. Choosing the right method can have implications for data interpretation and subsequent research steps.
- Data Quality and Reporting: It is imperative for researchers to adhere to stringent data collection and analysis standards. Agencies require detailed documentation of methodologies, controls, and results to ensure transparency and accuracy.
- Clinical Relevance: Procedures must reflect conditions that accurately mimic the physiological environment. This relevance is crucial for translating findings into real-world applications.
Benefits of Adhering to Regulatory Standards
Complying with regulatory perspectives brings numerous benefits, not only to researchers but also to the broader scientific community. These benefits include:
- Improved Trust in Research: By following established guidelines, researchers enhance the credibility of their findings.
- Facilitation of Approval Processes: For those in the pharmaceutical industry, adherence can lead to smoother regulatory approvals and quicker time to market for new drugs.
- Encouragement of Innovation: Strict regulatory standards have the potential to drive innovation, as they may push researchers to develop more effective and advanced methodologies.
"Regulatory compliance is not just a box to tick; it is a commitment to the integrity of science and the safety of patients."
Considerations for Future Research
As the landscape of biomedical research evolves, regulatory perspectives will continue to adapt. Future considerations may include:
- The incorporation of new technologies, like machine learning and microfluidic platforms, necessitating updates to existing regulations.
- Greater emphasis on the integration of in vitro and in vivo data, refining the approach to evaluating cell viability in the context of whole organisms.
- Expanding global harmonization of regulatory standards, ensuring that researchers worldwide have access to similar guidelines and expectations.
Future Directions in Cell Viability Research
Cell viability research has undergone significant advancements in recent years, yet many opportunities for further innovation remain. The future of this field can heavily influence a range of scientific inquiries, from cancer research to regenerative medicine. Understanding these future directions is essential for researchers and educators passionate about enhancing methodologies and outcomes.
Technological Innovations
Technological innovations play a crucial role in advancing the efficacy and accuracy of cell viability measurement. Some current trends include:
- High-throughput screening: This method allows for simultaneous testing of numerous samples, enabling researchers to gather large datasets quickly. The ability to process multiple conditions in one run can dramatically increase productivity.
- Lab-on-a-chip technology: This innovation integrates various laboratory processes on a single chip, yielding faster results with reduced sample size. Developing microfluidic systems can provide precise control over experimental conditions, leading to improved data quality.
- Nanotechnology: The integration of nanomaterials into cell viability assays can enhance sensitivity. Nanoparticles may facilitate the delivery of reagents or improve imaging techniques by highlighting cellular activities more clearly.
These innovations promise to streamline workflows and improve the detectability of subtle changes in viability levels, which are pivotal in many applications.
Integrating Machine Learning
The incorporation of machine learning algorithms into cell viability research presents a fresh frontier for data analysis and interpretation. Machine learning techniques can be used to:
- Predict cell responses based on historical data, allowing researchers to identify trends that may not be immediately apparent.
- Optimize assay conditions by analyzing the response patterns of different cell types to various substances, improving the design of experiments.
- Enhance image analysis: Automated systems can accurately quantify cell viability from images obtained through microscopy, minimizing human error and expediting result interpretations.
The integration of machine learning into biological research frameworks has the potential to redefine how we analyze and interpret biological data, leading to more robust findings.
As these technologies and techniques continue to evolve, researchers must adapt their methodologies accordingly. Embracing these advancements is crucial for pushing the boundaries of what is currently possible in cell viability assessment.
Epilogue
In summary, this article emphasizes the critical importance of measuring cell viability within various realms of scientific research. The topic is approached from multiple angles, revealing insights that are essential for students, researchers, educators, and professionals alike.
The measurement of cell viability has far-reaching implications, particularly in biomedical research, drug development, toxicological assessments, and stem cell studies. Understanding the differences between established and emerging methods allows researchers to make informed choices when designing experiments. This ensures that they achieve reliable and reproducible results, which is paramount in scientific investigation.
One significant benefit of employing accurate viability measurements is the ability to assess cellular health in response to treatments or environmental changes. These assessments guide the optimization of therapeutic strategies and mitigate risks associated with toxic compounds, enhancing the safety profile of potential drugs.
Considerations regarding the choice of assay method cannot be overstated. Factors such as sensitivity, specificity, cost, and throughput must align with the specific objectives of the research. For instance, while MTT assay provides quick results for high-throughput settings, techniques like flow cytometry can yield depth in analyzing cell populations but might involve higher costs and complexity.
As scientific methodologies evolve, keeping abreast of technological innovationsβsuch as machine learningβis vital. These advances promise to refine accuracy and efficiency in measuring cell viability, providing deeper insights into cellular behaviors across diverse contexts.
Ultimately, cell viability measurements serve not just as a technical requirement but also as a foundational pillar that supports broader scientific inquiries. By integrating the knowledge shared in this article, researchers can enhance their investigative processes and contribute meaningfully to advancements in biological science.