Esterase: Functions, Classes, and Applications Explored
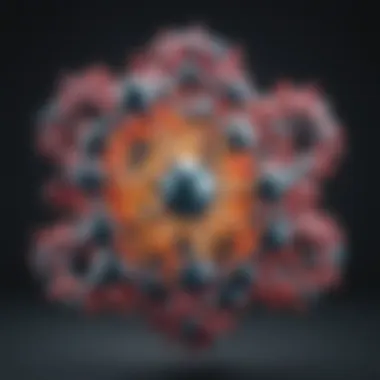
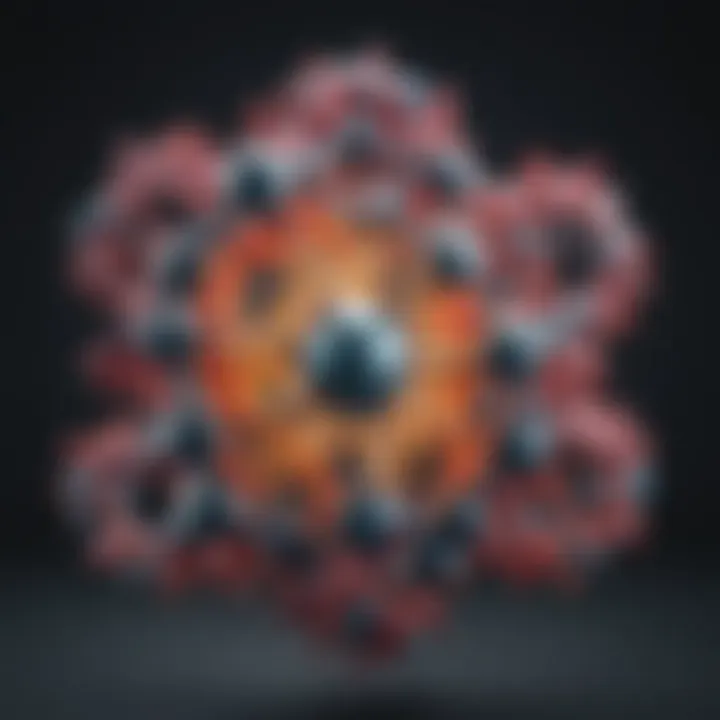
Intro
Esterases are more than just biochemical players; theyβre crucial for many biological systems. These enzymes catalyze the breaking of ester bonds, a reaction that is fundamental in various metabolic processes. In this exploration, weβll dissect the world of esterases, both from a functional standpoint and considering their diverse applications.
Understanding esterase enzymes not only helps to grasp the dynamics of biological systems but also their industrial significance. From agriculture to pharmaceuticals, esterases are pivotal in crafting solutions that benefit multiple sectors. Let's delve deeper into this essential enzyme family.
Prolusion to Esterases
Esterases play crucial roles in a variety of biological processes, acting as essential enzymes that hydrolyze ester bonds. This action is vital for functioning biological systems, influencing everything from metabolism to neurotransmission. Understanding esterases enables researchers to apply this knowledge in practical ways, extending beyond pure science into diverse industries.
Including a comprehensive discussion on esterases in this article serves several key purposes. Firstly, it expands the awareness of their importance, not just within biochemistry but also in industrial applications. Secondly, understanding the classification and mechanisms behind esterase action gives insights into how they can be manipulated for specific needs, benefiting fields like medicine and environmental science.
This article aims to provide a framework for recognizing the multifaceted roles of esterases. By systematically exploring their definition, historical context, classifications, and practical applications, readersβbe they students or professionalsβwill gain a thorough grounding in this significant area of study.
Definition and Overview
Esterases are enzymes that catalyze the hydrolysis of ester bonds, breaking them down into their constituent acids and alcohols. In simpler terms, if one thinks of esters as locks, esterases are the keys that open them, releasing smaller molecules that can be utilized by the body.
These enzymes are a part of several physiological processes, playing roles in lipid metabolism and neurotransmitter regulation, which are fundamental for maintaining homeostasis. There are two major classes of esterases: acyl-esterases and choline-esterases, each with its specific function and significance.
Additionally, esterases are widely present in living organisms β from humans to plants to bacteria. This ubiquity underscores the importance of esterase study in multiple biological and environmental contexts.
Historical Context
The study of esterases dates back to the mid-20th century when significant discoveries started linking these enzymes to various physiological functions. Initially, scientists recognized their action in the degradation of esters in the digestive system, but as research progressed, their roles expanded into neurobiology and cellular metabolism.
For instance, the identification of acetylcholinesterase in the late 1940s marked a turning point, highlighting how esterase activity is crucial for terminating neurotransmitter signals. This knowledge paved the way for developments in pharmacology, leading to the creation of enzyme inhibitors for treating neurological disorders.
Moreover, increasing awareness of esterase involvement in bioremediation processes emphasizes their adaptability. Research focused on environmental esterases has gained momentum, showcasing how these enzymes can break down pollutants, thus contributing positively to ecological conservation efforts.
"Esterases have evolved from being mere biocatalysts in digestion to pivotal players in pharmaceutical discoveries and environmental restoration."
Understanding the historical context enriches the narrative around esterases, illustrating a journey of discovery that continues to evolve with emerging technologies.
Classification of Esterases
Understanding the classification of esterases is pivotal for grasping their roles in various biological processes and industrial applications. This section delves into the ways in which esterases are categorized, emphasizing both functionality and structural characteristics. Each classification not only aids in organizing knowledge about these enzymes but also enhances the ability to study their diverse roles within different contexts.
By Functionality
Acyl-esterases
Acyl-esterases are crucial enzymes that primarily catalyze the hydrolysis of acylated compounds. Their role cannot be overstated, particularly in metabolism, where they help break down complex molecules into simpler forms. One defining characteristic of acyl-esterases is their broad substrate range, which allows them to act on various acyl groups and contribute significantly to lipid metabolism.
In the context of this article, the versatility of acyl-esterases makes them a popular choice for various applications, including biofuel production. Their ability to break down fatty acids into usable energy showcases their beneficial nature. However, one potential drawback is that the efficiency of acyl-esterases can vary depending on environmental factors, such as temperature and pH, which may be limiting in some industrial settings.
Choline-esterases
Choline-esterases, on the other hand, are best known for their involvement in neurotransmission. These enzymes break down acetylcholine, an essential neurotransmitter in nervous system signaling. The key characteristic that sets choline-esterases apart is their high specificity for choline-based substrates, making them indispensable for maintaining synaptic function.
The significance of choline-esterases in pharmacology is notable as well. Their ability to modulate neurotransmitter levels has implications for treating conditions like Alzheimer's disease. Yet, while they are extremely valuable, choline-esterases can have unwanted effects, such as when inhibiting their activity leads to excessive neurotransmitter accumulation, resulting in issues like muscle spasms.
By Structure
Serine Esterases
Serine esterases hold a distinctive position in the landscape of synthetic biology due to the presence of a serine residue in their active site. This characteristic is essential for their enzymatic function, allowing for the formation of a covalent enzyme-substrate complex that is key to their catalytic activity.
In this article, serine esterases are explored for their utility in industrial processes, significantly contributing to the chemical industry by facilitating the synthesis of various esters. Their robustness makes them favorable in diverse conditions. However, they can be limited by substrate specificity, occasionally leading to slower reactions when exposed to less suitable substrates.
Cysteine Esterases
Cysteine esterases stand apart due to the cysteine residue present at their active site. This specific structure grants them unique catalytic properties that are advantageous in certain biochemical pathways. They are particularly useful in studies involving proteolysis and lipid metabolism.
The distinct feature of cysteine esterases is their ability to perform reactions that are often impeded by traditional serine-based methods. This allows researchers to explore innovative approaches in biotechnology and therapeutic development. Nevertheless, their sensitivity to oxidation can be a significant disadvantage, potentially hindering their application in industrial processes.
In summary, the classification of esterases, whether by functionality or structure, provides crucial insights into their respective roles and applications. Understanding these categories furthers our knowledge of how esterases impact both biological processes and industrial innovations.
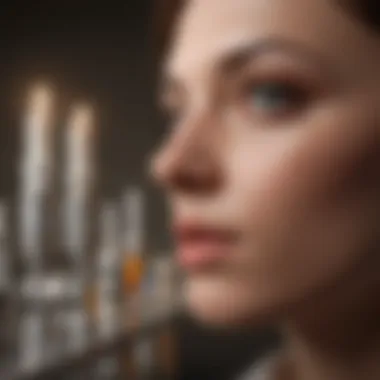
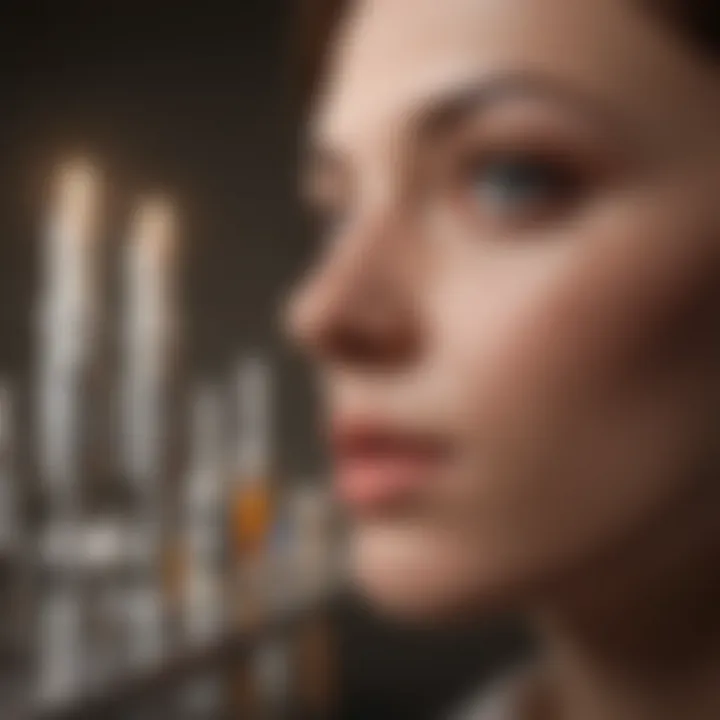
With this foundational understanding, we can now transition to the mechanisms by which these enzymes exert their influence in various biological and industrial contexts.
Mechanisms of Esterase Action
Understanding how esterases work is key to grasping their significance in both biological systems and industrial applications. These enzymes catalyze the hydrolysis of ester bonds, and the specifics of their mechanisms can vary widely. This diversity allows them to perform distinct roles in various biochemical processes, highlighting their adaptability.
Enzymatic Catalysis
At the core of esterase action is enzymatic catalysis, a highly efficient process that accelerates chemical reactions. In the case of esterases, the hydrolysis of ester bonds occurs through a multi-step mechanism. This typically involves the formation of an enzyme-substrate complex where the ester bond is positioned in a way that optimally facilitates reaction.
Through catalytic triads β usually made up of serine, histidine, and aspartate β esterases lower the activation energy required for the reaction. This catalytic action can be depicted as follows:
- Substrate Binding: The ester substrate binds to the active site of the esterase enzyme.
- Formation of Tetrahedral Intermediate: The catalytic serine attacks the carbonyl carbon of the ester, creating a tetrahedral intermediate.
- Collapse of the Intermediate: This leads to the cleavage of the ester bond, releasing the alcohol and producing an acyl-enzyme complex.
- Release of Products: Finally, water attacks the acyl-enzyme, regenerating the serine and releasing the acid product.
This process showcases not just the effectiveness of esterases but also their specificity to different substrates, emphasizing the nuanced control they have over reactive pathways.
Substrate Specificity
Another pivotal aspect of esterase action is substrate specificity. Not all esterases are created equal when it comes to the types of esters they can hydrolyze. This variability in substrate affinity stems from differences in the enzyme's active site, which is shaped in a way to recognize specific functional groups and chain lengths within substrates.
- Short-chain vs. Long-chain Esters: Some esterases preferentially act on small substrates like acetate esters, while others display a genuine fondness for larger, more complex molecules.
- Functional Group Recognition: The presence of certain functional groups can enhance or diminish binding affinity, further illustrating the enzymes' unique interactions with their substrates.
- Environmental Conditions: Factors like pH and temperature can also influence how specifically an esterase will act on its substrate, affecting its overall efficiency and effectiveness in various environments.
By understanding the mechanisms of esterase action, including how they catalyze reactions and their specificity for substrates, researchers can begin to leverage these enzymes in various fields, from biotechnology to pharmaceuticals. Their potential is vast, with applications that speak to both their efficiency and adaptability.
Physiological Roles of Esterases
Esterases are more than just a set of enzymes that carry out hydrolysis of ester bonds; they are true juggernauts of biological functionality. Their contributions to various physiological processes underline their significance in maintaining metabolic balance and overall health. Understanding the roles of esterases in metabolism and neurotransmission helps reveal their broader implications in medicine and industry. Their intricate functions indicate how they act as mediators in lifeβs biochemical dialogues, making them crucial players at a cellular level.
Metabolism of Lipids and Carbohydrates
One of the most prominent roles of esterases lies in their involvement in the metabolism of lipids and carbohydrates. These enzymes facilitate the breakdown of ester bonds present in triglycerides, phospholipids, and other lipid molecules, which are essential sources of energy and structural components for cell membranes.
- Lipid metabolism: Esterases break down triglycerides into glycerol and free fatty acids, which can be utilized for energy production or stored for future use. For example, when a person exercises, the body demands energy, and esterase activity accelerates the mobilization of lipid stores to meet these needs. Such processes illustrate that esterases are not a mere sideshow in energy metabolismβthey are vital for ensuring that cells have what they need when they need it.
- Carbohydrate metabolism: In certain cases, esterases also participate in carbohydrate metabolism. They help in the hydrolysis of sucrose and other glycosidic compounds, contributing to the overall flow of glucose in metabolic pathways. This adjustment ensures that energy production is harmoniously aligned with the body's demands.
Esterases effectively manage the ebb and flow of these fundamental substances, pointing to their critical roles in metabolic health. One might say they are the quiet custodians of our bio-energetic needs.
Significance in Neurotransmission
Beyond metabolism, esterases play a pivotal role in neurotransmission, which is a key process for communication within the nervous system. Notably, acetylcholinesterase is an enzyme of substantial importance in breaking down the neurotransmitter acetylcholine in the synaptic cleft. This action facilitates the termination of signal transmission, thereby preventing overstimulation of neurons.
- Regulation of neurotransmitter levels: The rapid breakdown of acetylcholine by esterases ensures that neurotransmitter levels are tightly regulated, allowing for precise communication between nerve cells. When this mechanism is disrupted, it can lead to a cascade of neurological issues, such as in the case of certain neurodegenerative diseases.
- Pharmacological implications: The role of acetylcholinesterase has significant implications in pharmacology as well. For instance, certain drugs used in treating Alzheimer's disease are designed to inhibit this enzyme, thereby increasing the levels of acetylcholine in the brain to enhance cognitive functions. This showcases how esterase activity isn't just a biological necessity; it intersects with therapeutic strategies aimed at addressing complex health issues.
"Esterases, like skilled craftsmen, shape and mold the biochemical landscape of our bodies, reflecting their importance in health and disease."
Environmental Influences on Esterase Activity
Understanding the environmental influences on esterase activity is crucial, as these factors can significantly impact enzymatic functions in various biological and industrial processes. Esterases, like other enzymes, do not work in isolation; their activity is profoundly affected by the surrounding environment. Key elements include temperature, pH levels, and the presence of inhibitors, each of which can shift the efficiency and efficacy of esterase-catalyzed reactions.
The importance of recognizing these environmental factors lies in their direct implications for optimization in biotechnological applications. A comprehensive grasp of how these variables affect esterase activity aids in enhancing industrial processes, improving yields, and tailoring specificity for desired products.
Temperature and pH Levels
Both temperature and pH serve as fundamental determinants of esterase activity. Temperatures that stray too high or low can lead to inactivation or denaturation of the enzyme, thus halting its function completely. Generally, esterases exhibit peak activity at specific temperatures, typically between 30Β°C to 60Β°C, depending on the source of the enzyme.
On the other hand, pH levels dictate the charge and structure of the enzymatic active site, affecting the binding interactions with the substrate. Each esterase has an optimal pH that must be maintained for maximum activity. For example, human butyrylcholinesterase operates best around a slightly alkaline pH, while some microbial esterases thrive in more acidic conditions.
Presence of Inhibitors
Inhibitors are substances that can diminish or shut down enzyme activity altogether. The presence of certain chemicals or metabolites in the environment serves as inhibitors that hinder esterase action.
Factors that can lead to inhibition include:
- Heavy metals: Contaminants like lead or cadmium can bind to esterase, altering its conformation and reducing efficiency.
- Affinity binding agents: Certain drugs or ligands can compete with substrates, effectively blocking the active site.
Understanding the mechanisms of inhibition is crucial for designing bioreactors or applications in which esterase activity is valuable. Incorporating strategic approaches to mitigate inhibitor effects can enhance overall process outcomes.
"In the realm of enzyme activity, the environment plays a decisive role; ignoring it would be like trying to catch fish in a desert."
Applications of Esterases in Industry
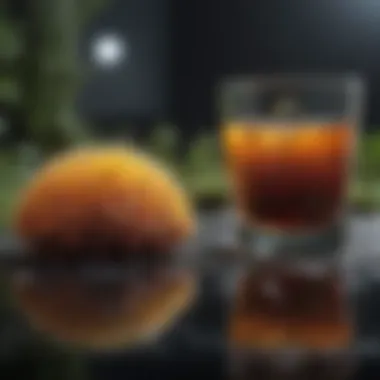
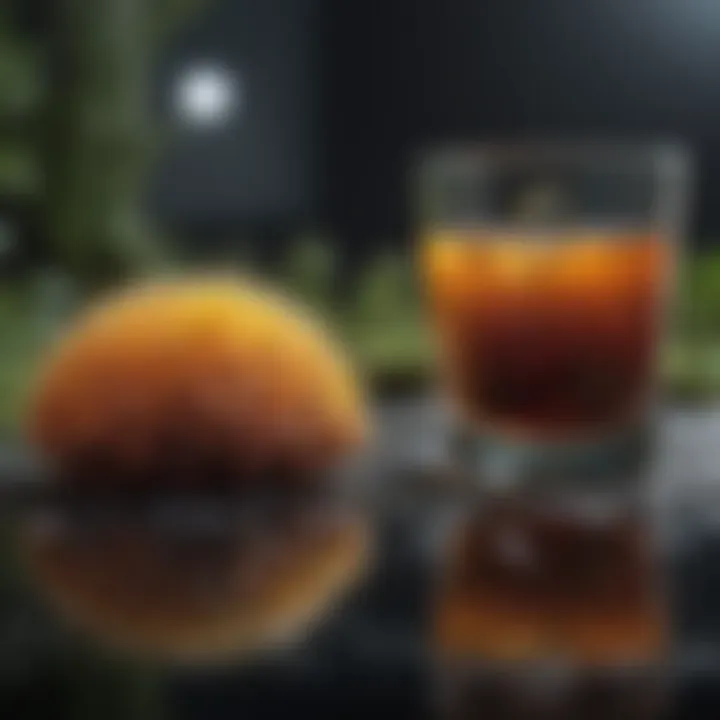
Esterases have carved a niche for themselves in various industrial settings, serving as essential catalysts that offer both efficiency and specificity. The versatility of these enzymes extends to multiple sectors, including pharmaceuticals, agriculture, and food processing. By leveraging the unique properties of esterases, industries manage to enhance their processes while also adhering to principles of sustainability. This section delves into two significant applications of esterases in industry, highlighting the benefits, considerations, and the broader implications of their use.
Biocatalysts in Industrial Processes
Biocatalysis has become a buzzword in the industrial landscape, notably due to its environmentally friendly nature and ability to streamline chemical processes. Esterases, with their ability to catalyze the hydrolysis of ester bonds, play a crucial role here. They are particularly effective in the production of biofuels, flavor compounds, and sustainable polymers.
- Efficiency: Esterases work under mild conditions, requiring less energy compared to traditional chemical catalysts. This can reduce operational costs significantly.
- Selectivity: Their substrate specificity ensures that the desired products are formed with minimal byproducts, enhancing purity and product yields.
- Eco-friendliness: Using biocatalysts like esterases minimizes the environmental impact associated with harmful chemicals employed in synthetic processes.
However, the implementation of esterases is not without challenges.
- Stability: These enzymes can be sensitive to changes in temperature and pH, which may affect their functionality in certain industrial settings.
- Production Costs: While they can reduce expenses, the initial costs associated with enzyme production and optimization can be considerable.
"The ever-growing focus on sustainable practices makes the exploration of esterases a pressing concern for industries aiming for greener solutions."
Pharmaceutical Manufacturing
In the realm of pharmaceuticals, esterases are invaluable. They are implemented in various stages of drug development and formulation, particularly for the synthesis of prodrugs. Prodrugs, which are inactive compounds converted into active drugs upon administration, often rely on esterases for activation.
- Targeted Delivery: By utilizing esterases, pharmaceutical companies can produce drugs that are activated specifically at the site of action, thereby reducing side effects and improving therapeutic efficacy.
- Complexity Management: Esterases can simplify complex synthetic pathways, enabling more straightforward and cost-effective production of intricate drug molecules.
- Metabolic Profitability: As many naturally occurring substances have esterase-sensitive elements, utilizing these enzymes can lead to novel drug discoveries through natural product derivation.
Despite these benefits, there are considerations that must be taken into account:
- Regulatory Compliance: The pharmaceutical industry faces stringent regulations. The use of biocatalysts necessitates thorough documentation and validation to meet these standards.
- Scale-Up Challenges: Transitioning processes from laboratory to industrial scale can lead to unexpected results, raising crucial questions about activity, stability, and overall yield.
These applications illustrate that esterases are not merely adjuncts in industrial practices; they are pivotal in driving efficiency, sustainability, and innovation. As research continues to evolve, the potential for new uses and heightened efficiency presents a promising horizon for the utilization of esterases in various industries.
Esterases in Biotechnology
Esterases represent a class of enzymes that are crucial in various biotechnological applications. Their ability to catalyze the hydrolysis of ester bonds makes them pivotal in numerous processes, ranging from drug development to waste management. The versatility of esterases enhances their utility, allowing applications not only in industrial settings but also in environmental preservation efforts. As such, understanding the role of esterases in biotechnology reveals their significance in addressing pressing global challenges.
Genetic Engineering Applications
In genetic engineering, esterases have emerged as powerful tools for modifying biomolecules. By utilizing these enzymes, scientists can tailor the properties of specific substrates, facilitating the creation of new compounds that might not be achievable through traditional chemical methods. This precision is particularly crucial in the development of biopharmaceuticals, where esterases can help in the formulation of active pharmaceutical ingredients by ensuring that they meet specific criteria for efficacy and stability.
Moreover, esterases play a role in modifying the genetic structures of organisms. For example, researchers can introduce esterase genes into bacteria to enable the production of biodiesel from agricultural waste. This process not only enhances the sustainability of fuel production but also reduces waste, making it a win-win situation.
Esterases have also found their niche in the area of diagnostics. Their ability to interact with a variety of substrates allows for the development of biosensors that can detect specific compounds in biological samples.
Bioremediation Efforts
Bioremediation is the process of using living organisms to remove or neutralize contaminants from the environment, and esterases are vital players in this field. They can break down hazardous substances, such as pesticides or plastics, into less harmful compounds. For instance, certain esterase-producing bacteria have been shown to degrade organophosphate pesticides, making them invaluable for agricultural cleanup processes.
Notably, the biodegradation pathways hinted by esterases have sparked interest in creating bioengineered solutions for environmental cleanup.
Benefits of Esterases in Bioremediation:
- Efficiency: Esterases can work under a variety of environmental conditions, such as different pH levels and temperatures, making them adaptable for real-world applications.
- Specificity: They can be engineered to target specific pollutants, increasing the effectiveness of remediation efforts.
- Cost-Effective: Utilizing microorganisms that produce esterases can reduce the costs associated with traditional remediation methods.
Moreover, the ongoing research simulating esterase pathways can lead to more innovative approaches in restoring contaminated sites, offering a glimpse into a cleaner, safer future.
In essence, esterases are not just enzymes; they are powerful allies in the pursuit of sustainability and environmental health.
Research Trends in Esterase Studies
The study of esterases is constantly evolving, with new research trends emerging that push the boundaries of our understanding. These trends are significant not only for theoretical knowledge but also for practical applications across various fields. In recent years, there has been an increased focus on the engineering of these enzymes to enhance their performance and efficiency. Furthermore, the exploration of therapeutic potentials for esterase inhibitors is gaining traction. Understanding these trends provides insights into both the challenges and opportunities within the realm of esterase research.
Enzyme Engineering and Optimization
One of the prominent research trends in the study of esterases is enzyme engineering and optimization. This methodology is aimed at improving the catalytic efficiency of esterases, which ultimately enhances their applicability in various industrial processes. Researchers have begun to utilize techniques like directed evolution and rational design to tailor esterase properties.
- Directed Evolution: This approach mimics the process of natural selection to develop enzymes with desired traits. Through repeated rounds of mutation and selection, new esterase variants can be produced that may exhibit enhanced substrate specificity or resistance to environmental factors.
- Rational Design: Here, scientists utilize structural information gleaned from X-ray crystallography and molecular modeling to make informed changes to the enzyme's amino acid sequence. This can lead to significant improvements in the enzyme's function.
In addition to enhancing functionality, optimizing esterases for specific conditions can expand their utility in biocatalytic applications. For example, by tweaking temperature resilience, researchers can develop esterases that operate effectively in industrial environments that were previously unsuitable.
Therapeutic Potential
The therapeutic potential of esterases is another area of growing interest in scholarly use. Researchers are investigating the role of esterases in drug metabolism and their interaction with specific pharmaceuticals. By understanding these mechanisms, scientists hope to mitigate adverse drug reactions and improve patient outcomes.
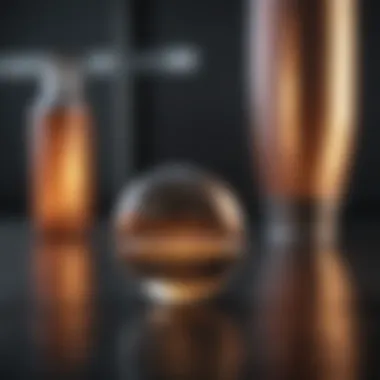
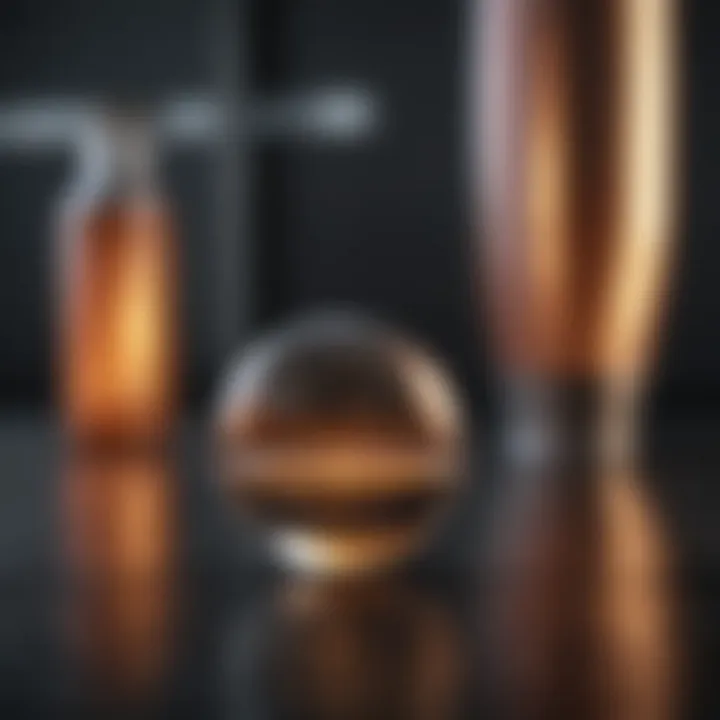
- Drug Metabolism: Esterases are involved in the hydrolysis of ester bonds in various drugs. This process can alter the pharmacokinetics of the compounds, potentially leading to either more effective treatments or harmful side effects. A better understanding of this interaction can inform safer drug design.
- Esterase Inhibitors: These compounds have the potential to modulate the activity of esterases in therapeutic contexts, such as in the treatment of neurodegenerative diseases. For instance, donepezil, an acetylcholinesterase inhibitor, has shown promise in managing symptoms of Alzheimer's disease, emphasizing the role esterase inhibition can play in medicinal chemistry.
"The focus on therapeutic applications, alongside continuous improvements in enzyme technology, showcases the dynamic nature of esterase research."
Overall, staying glued to these trends not only lays a pathway to innovate existing products but also reveals the many ways esterase studies intertwine with pressing challenges in biochemistry and medicine. As new techniques emerge and understanding deepens, the future of esterase research looks bright, with the promise of significant impacts across various sectors.
Challenges in Esterase Research
The exploration of esterase enzymes presents a variety of challenges that can impede both academic inquiry and practical applications. Identifying and addressing these challenges is crucial for advancing our understanding and implementation of these enzymes. The complexities involved shape the research landscape and dictate the future direction of studies in this vital field.
Characterization Difficulties
When scientists embark on the journey to study esterases, they often hit a wall due to the characterization difficulties associated with these enzymes. The hurdle lies in the diverse structural and functional aspects of esterases. With various subclasses, determining the specific characteristics of a particular esterase can be like searching for a needle in a haystack.
Key aspects contributing to these challenges include:
- Heterogeneity in Enzyme Properties: Esterases display a range of kinetic properties and substrate specificities. This variability makes it challenging to develop universal characterization methods.
- Purification Issues: Isolating esterases in sufficient quantities for study demands meticulous techniques. The lack of robust methods can lead to unrepresentative samples and skewed data.
- Detection Limitations: The existing assays for detecting esterase activity might not be sensitive enough for some applications, making it hard to quantify activities reliably.
Overall, these characterization difficulties can stall experimental progress, leading to gaps in our understanding of their biological roles and potential uses.
Regulatory Hurdles
As the field of biochemistry faces increasing scrutiny, regulatory hurdles have emerged as significant barriers in esterase research. Navigating the labyrinth of regulations demands a comprehensive understanding of legal frameworks, compliance, and ethical considerations.
Challenges include:
- Approval Processes: Rigorous approval processes can slow down research and development cycles in biotechnological applications that utilize esterases. Familiarity with these procedures is essential for researchers.
- Environmental Assessments: Esterases proposed for bioremediation efforts may require exhaustive environmental impact assessments. These assessments can be time-consuming and expensive.
- Intellectual Property Issues: In a competitive landscape, securing intellectual property rights can complicate collaborations and limit innovative approaches to esterase usage.
Together, these regulatory hurdles create a climate of uncertainty for researchers, slowing down advancements in this area. Addressing these could help foster a more conducive environment for innovation and discovery in the realm of esterase enzymes.
Future Directions in Esterase Research
As the study of esterases progresses, numerous enlightening pathways emerge that hold promise for future exploration. Recognizing the significance of these enzymes in biological systems, industries, and health-related fields fuels the need for ongoing research. This section delves into emerging technologies and cross-disciplinary collaborations that are set to shape the trajectory of esterase research over the coming years.
Emerging Technologies
The rise of novel technologies in biotechnology and enzyme sciences is pivotal in opening new avenues for esterase investigations. By utilizing methods such as CRISPR-Cas9 gene editing, scientists can tailor esterase genes for enhanced functionalities. This genetic engineering allows researchers to produce esterase variants that exhibit improved stability or substrate specificity, addressing constraints found in natural enzymes.
Microbial consortia, especially those from extreme environments, present another exciting frontier. Isolating esterases from such organisms, which thrive in unusual conditions, can reveal enzymes with remarkable properties useful in industrial applications. The exploration of metagenomics further expands the toolkit. Researchers can now sift through vast genetic data to find novel esterase genes, offering fresh insights into enzyme diversity.
Technologies such as mass spectrometry and high-throughput screening for enzyme assays have streamlined the process of discovering new esterases. These advancements allow for rapid validation of enzyme efficiency and activity profiles, making it simpler to identify candidates for specific applications in chemical processes or therapeutic developments.
Potential Cross-Disciplinary Collaborations
The future of esterase research is not restricted to a single field; in fact, collaboration among multiple disciplines is increasingly seen as essential for breakthroughs. One particularly fruitful area is the intersection of biochemistry, material science, and data analytics. Researchers can integrate machine learning approaches with biological data, resulting in predictive models for enzyme performance under varying conditions. Such collaboration can significantly speed up the development of engineered esterases tailored for specific industry needs, be it in biodegradable plastics or environmentally friendly solvents.
"Harnessing the collective expertise across disciplines can lead to transformative solutions, enhancing the utility of esterases in addressing critical global challenges."
Additionally, partnerships with environmental scientists can yield insights into the role of esterases in ecosystems, particularly concerning their involvement in bioremediation. Studying esterases that break down pollutants like pesticides or oil spills demonstrates how coordination across fields can lead to sustainable practices.
Moreover, input from medical researchers can guide the exploration of esterases as drug targets or delivery systems, paving the way for novel therapeutic strategies. By leveraging insights from pharmacology, esterase studies may inform developments in targeted drug design, optimizing drug metabolic pathways while minimizing side effects.
In summary, the future directions in esterase research hinge on groundbreaking technologies and robust interdisciplinary collaborations. With these approaches, the potential for innovative discoveries and applications seems boundless. As we continue to peel back the layers of esterase functions and capabilities, the biochemistry landscape will undoubtedly evolve, yielding new responses to longstanding challenges.
Ending
In wrapping up our discussion on esterases, itβs crucial to recognize their undeniable importance in various realms, from basic biology to extensive industrial applications. This article illuminates the multifaceted roles esterases play, not just as biological catalysts facilitating chemical reactions but also as integral components of larger physiological processes.
Summary of Key Findings
Throughout the article, several key elements have emerged that underscore the significance of esterases:
- Esterases are vital enzymes: They catalyze the hydrolysis of ester bonds, a reaction essential for metabolism and other biological tasks.
- Diverse classifications: Esterases can be categorized richly by both their functionality and structural characteristics, which suggests diverse applications across different fields.
- Mechanisms of action: Understanding how esterases work on a molecular level reveals the specificity and efficiency of these enzymes, paving the way for innovative uses.
- Influence in biotechnology: From genetic engineering to bioremediation, the applications of esterases showcase their versatility beyond natural roles.
- Emerging research trends: The attention directed toward esterase studies in possibility of therapeutic applications highlights a promising avenue for future discoveries.
These findings not only portray esterases as dynamic entities within biological systems but also as promising agents in various industrial and environmental contexts.
Final Thoughts on Esterase's Significance
The significance of esterases cannot be overstated. Their roles encompass critical physiological elements, such as the metabolism of lipids and neurotransmission, highlighting their necessity for healthy biological function. Moreover, the potential of esterases in industrial applications, such as their safe use in pharmaceuticals and various manufacturing processes, demonstrates their broad relevance.
Moreover, as research continues to evolve, the possibility of esterase engineering opens doors to enhancing their efficiency and expanding their utility. In light of the environmental challenges we face, leveraging these enzymes for bioremediation presents an exciting frontier worth exploring.
The journey of understanding esterases is far from complete. As scientific exploration unfolds, these enzymes are likely to reveal even more secrets, affirming their place as crucial players in both nature and technology.
Esterases not only bridge biological processes but also enhance industrial innovations, embodying a perfect blend of nature and technology.