Batteries in Electric Cars: An In-Depth Exploration
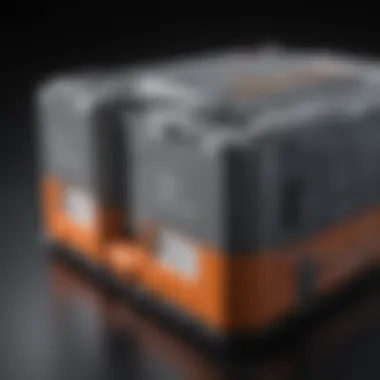
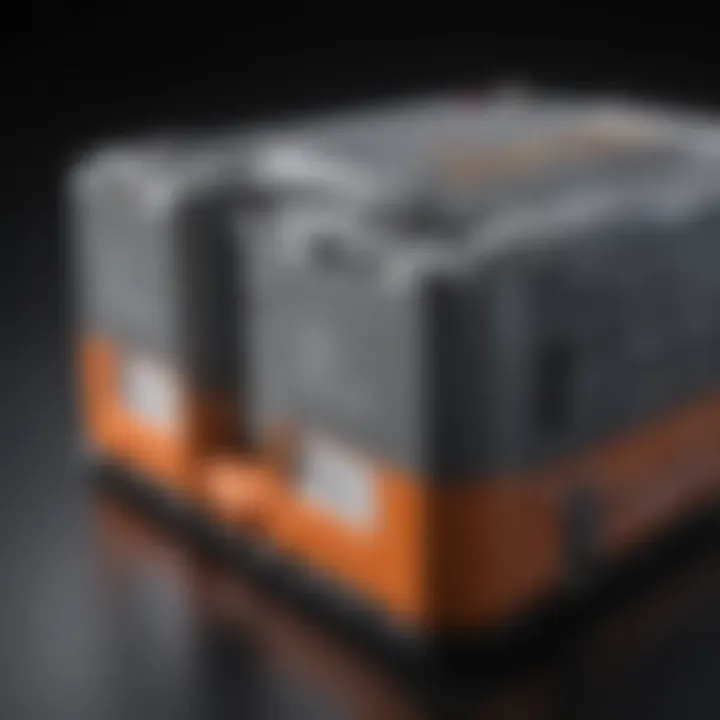
Intro
The role of batteries in electric vehicles (EVs) is pivotal, shaping the future of transportation. As the global push for sustainability intensifies, the importance of efficient and environmentally friendly battery systems grows. Understanding the nuances of battery technology is essential for students, researchers, educators, and professionals alike, as they seek to comprehend how it influences everything from vehicle performance to ecological impact.
As we delve into the various types of batteries used in electric vehicles, we explore their efficiency, lifecycle, and the potential for future innovations. This comprehensive examination outlines the technological advancements in the field, providing insights that can inform decision-making and further research.
Research Overview
Summary of Key Findings
Batteries used in electric cars have evolved tremendously in recent years. Lithium-ion batteries are now the standard due to their balance of weight, capacity, and efficiency. However, alternative chemistries, such as solid-state and sodium-ion batteries, are gaining attention due to their potential advantages in energy density and safety.
Efficiency is measured not just in energy storage but also in terms of lifecycle impact and recycling capabilities. The industry faces challenges related to resource extraction, especially concerning cobalt and lithium, which necessitates a shift toward sustainable practices throughout the battery lifecycle.
Research Objectives and Hypotheses
The primary objective of this research is to evaluate current technologies and their implications for EV performance and environmental impact. Specific hypotheses include:
- Advancements in battery technology will lead to improved vehicle efficiency.
- The transition to sustainable materials will enhance the lifecycle of EV batteries.
- Public acceptance of electric vehicles is correlated with improvements in battery performance and charging infrastructure.
Methodology
Study Design and Approach
This examination combines qualitative and quantitative methodologies to provide a well-rounded analysis. Literature reviews from academic journals and industry reports form the backbone of this research, complemented by case studies on recent EV models. These diverse data points illustrate the current state of battery technology in relation to market demand and environmental pressures.
Data Collection Techniques
Data are collected from various reputable sources, including:
- Industry reports from organizations like the International Energy Agency.
- Academic publications focusing on chemical engineering and sustainability.
- Online discussions in forums such as Reddit, which offer insights from users regarding their experiences with different EVs.
"Batteries are the heart of electric vehicles; understanding their technology is crucial for the advancement of sustainable transport."
This layered approach allows for a comprehensive understanding of battery technology and its implications for the future of electric vehicles.
Intro to Electric Vehicle Batteries
Batteries are at the heart of electric vehicles, serving as their primary power source. Understanding the role of batteries in electric cars is fundamental to grasp the broader implications of electric mobility. With the automotive industry evolving rapidly toward electrification, battery technology is pivotal in determining the performance, efficiency, and sustainability of electric vehicles.
Overview of Electric Vehicles
Electric vehicles (EVs) are designed to operate using electric energy stored in batteries rather than traditional fossil fuels. They fall into distinct categories such as battery electric vehicles (BEVs) and plug-in hybrid electric vehicles (PHEVs). The rise of electric cars is largely driven by advancements in technology, decreasing production costs, and increasing environmental regulations.
As consumers become more conscious about climate change, electric vehicles are seen as a cleaner alternative to conventional vehicles, significantly reducing greenhouse gas emissions. The growth of this market also intensifies the demand for better battery technologies that provide greater range, longevity, and efficiency.
Importance of Battery Technology
Battery technology determines not only how far an electric vehicle can travel on a single charge but also influences overall vehicle design and performance. Key factors of battery technology include energy density, charge time, and cycle life.
In the context of electric vehicles, battery performance directly correlates with user experience. For instance, longer range capabilities lead to greater convenience for drivers. Furthermore, innovations in battery technology can reduce costs, making electric vehicles more accessible to a broader audience.
"The transition to electric mobility is fundamentally linked to advancements in battery technology, which will shape the future of how we transport goods and people."
In sum, understanding the importance of batteries in electric vehicles is essential not only for consumers but also for manufacturers and policymakers. It enables better decisions that address sustainability, economic viability, and technological advancement.
Types of Batteries Used in Electric Cars
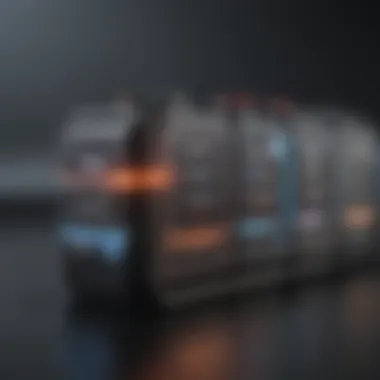
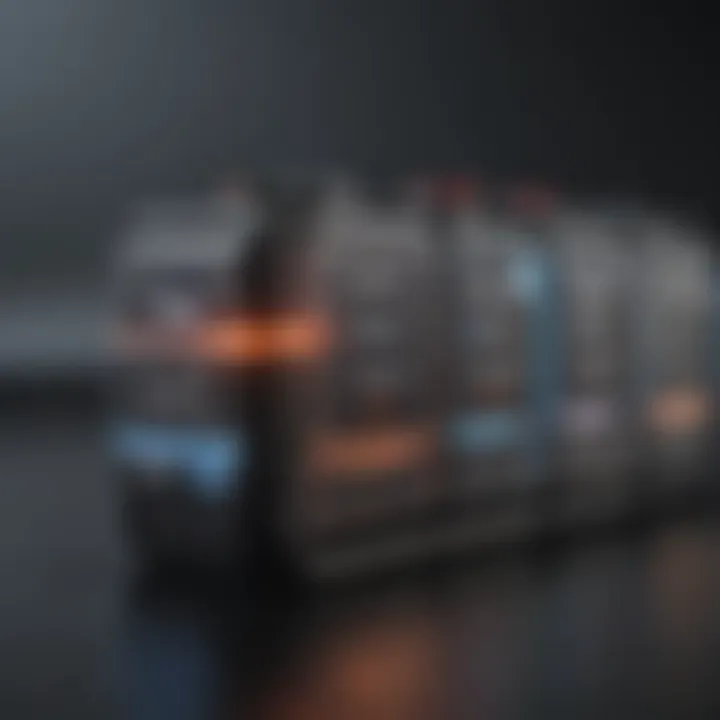
Understanding the types of batteries used in electric cars is crucial for grasping their overall performance, efficiency, and environmental impact. Each type of battery carries unique advantages and disadvantages. The choice of battery can significantly affect the vehicle's range, charge time, longevity, and safety. Therefore, a clear understanding of these battery types aids in making informed decisions regarding electric vehicle technology and infrastructure.
Lithium-Ion Batteries
Lithium-ion batteries dominate the electric vehicle market today. Their high energy density and relatively light weight make them a preferred choice for most manufacturers. A typical lithium-ion battery consists of a positive electrode, a negative electrode, and an electrolyte. This design facilitates efficient electron mobility, contributing to faster charging times and greater range.
One key advantage is their ability to undergo a large number of charge cycles without significant performance degradation. They can typically last anywhere from 8 to 15 years, depending on usage and climate. This longevity minimizes the need for premature replacements. Notably, numerous electric cars, such as the Tesla Model 3, rely on lithium-ion technology.
However, they are not without limitations. Lithium-ion batteries can experience overheating issues, which raises safety concerns. Additionally, their production requires significant mining of lithium, cobalt, and nickel, raising environmental issues. Nevertheless, ongoing improvements in battery management systems and cooling technologies aim to address these concerns effectively.
Solid-State Batteries
Solid-state batteries represent an innovative step forward in battery technology. Unlike traditional lithium-ion batteries that use liquid electrolytes, solid-state batteries use solid electrolytes. This design enhances safety, as it reduces the risk of fires and leaks, common challenges with liquid-based batteries.
The energy density of solid-state batteries can be significantly higher than that of lithium-ion counterparts. This suggests a greater potential driving range for electric vehicles. Furthermore, solid-state batteries typically offer faster charging times and a longer lifecycle. The combination of these factors presents considerable advantages, making solid-state technology a focal point of research and investment in the electric vehicle sector.
Despite their promising advantages, production is still in experimental stages. Manufacturing techniques for solid-state batteries remain complex and costly, limiting widespread adoption. Many industry leaders, like Toyota, are actively researching this technology, aiming to overcome these hurdles.
Nickel-Metal Hydride Batteries
Nickel-metal hydride batteries have been used in electric vehicles for several decades but are slowly being phased out in favor of lithium-ion and solid-state technologies. Their main benefit is that they are more resilient to temperature variations, making them suitable for diverse climates.
These batteries can offer a longer lifespan in some applications compared to lithium-ion batteries. However, they generally have lower energy density. This means that vehicles with nickel-metal hydride batteries typically have shorter ranges. One prominent case of this technology in action was with the Toyota Prius.
Environmental concerns are present as well. Nickel extraction and processing can lead to negative ecological impacts. Moreover, while recycling processes exist, they are not as streamlined as those for lithium-ion batteries.
In summary, the types of batteries used in electric cars play a pivotal role in defining the capabilities and environmental impact of electric vehicles. The evolution of battery technology continues to shape the future of transportation, guiding research and investment towards sustainable solutions.
Battery Performance Metrics
In the realm of electric vehicles, battery performance metrics serve as critical benchmarks that determine the success of battery technologies. These metrics influence not only the operational efficiency but also the user's overall experience with electric vehicles. Understanding these metrics is vital for assessing the viability of various battery types in real-world applications. The three main performance metrics include energy density, charge time, and cycle life.
Energy Density
Energy density is a measure of how much energy a battery can store per unit of weight or volume. High energy density means that a battery can store more energy without significantly increasing its weight or size. This is particularly important in electric cars, where reduced weight directly correlates with improved efficiency and range. With advancements in lithium-ion technology, modern batteries can achieve energy densities that allow electric vehicles like the Tesla Model 3 to travel over 300 miles on a single charge.
The significance of energy density extends beyond just range. Lower weight helps in enhancing vehicle handling and performance. As automakers seek to make electric vehicles more competitive with internal combustion engine vehicles, improvements in energy density are a key target for research and development.
Charge Time
Charge time refers to the duration required for a battery to reach full capacity from a depleted state. This metric is essential for the convenience of electric vehicle users. Long charge times can deter potential buyers from transitioning to electric vehicles since they may be seen as less practical than traditional cars.
Charging technology can significantly affect this aspect. For instance, fast chargers can replenish a battery to about 80% capacity in under 30 minutes. Models such as the Porsche Taycan utilize ultra-fast charging capabilities to drastically reduce wait times. Thus, advancements in charge time will likely have a profound impact on the overall adoption of electric vehicles.
Cycle Life
Cycle life indicates how many complete charge and discharge cycles a battery can undergo before its capacity diminishes to a certain percentage of its original capacity. This metric is essential for understanding the longevity and durability of a battery. Typically, lithium-ion batteries exhibit cycle lives ranging from 500 to 2,000 cycles, depending on various factors like temperature, depth of discharge, and charge rates.
The economic implications of cycle life are significant for both consumers and manufacturers. Batteries with higher cycle lives reduce replacement costs over time, making electric vehicles more appealing. Furthermore, understanding cycle life can also enhance battery management systems, enabling better maintenance strategies.
"Battery performance metrics not only affect the cost and efficiency of electric vehicles but also their market acceptance and consumer trust in the technology."
To conclude, energy density, charge time, and cycle life represent essential aspects of battery performance metrics. These metrics are vital for assessing the current and future capabilities of electric vehicle batteries, influencing design considerations and consumer perceptions. As technology advances, we can expect continued improvements in these areas, further enshrining electric vehicles in the automotive landscape.
Environmental Considerations
Environmental considerations play a crucial role in the discussion surrounding electric vehicle batteries. Understanding the environmental impact involves analyzing both the sourcing of raw materials and the end-of-life options for batteries. These aspects are essential for evaluating the sustainability and responsibility of battery technology in promoting greener transportation solutions.
Raw Material Sourcing
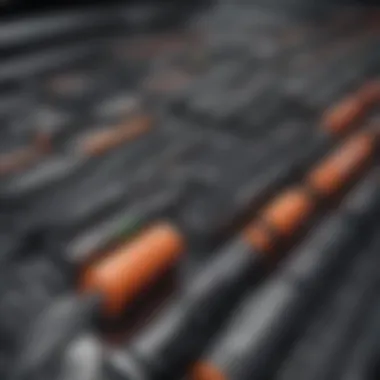
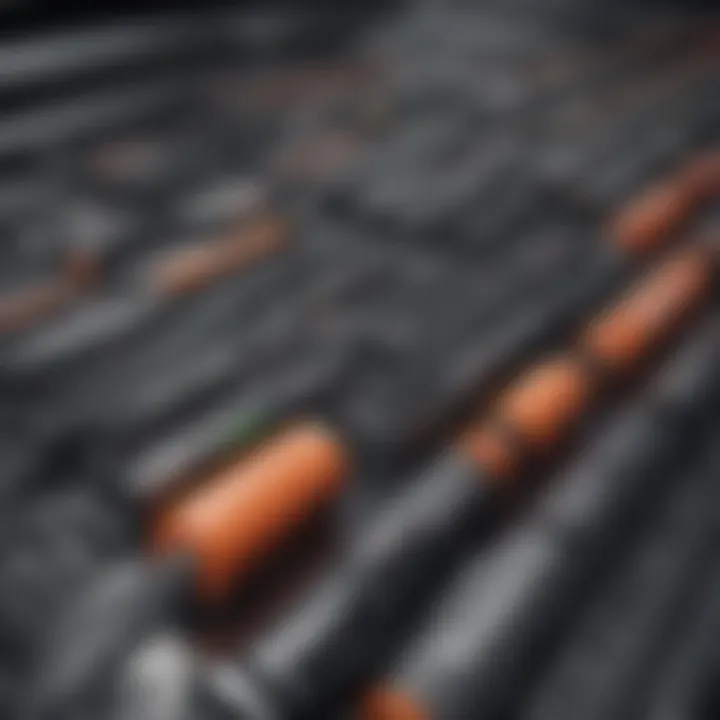
The production of batteries necessitates various raw materials, including lithium, cobalt, nickel, and graphite. The extraction and sourcing of these materials must be approached with caution.
- Environmental Impact: Mining can lead to habitat destruction, soil degradation, and water pollution. Cobalt, for instance, is often mined in regions that engage in practices detrimental to the environment and the local communities.
- Ethical Sourcing: Concerns regarding the sourcing of materials have raised questions about human rights violations in some mining practices, especially in countries where regulations are lax. Companies are facing pressure to ensure that their supply chains are transparent, ethical, and sustainable.
- Innovative Practices: To address these challenges, some manufacturers are exploring alternative sources and recycling methods. The use of advanced technologies in extraction and the development of synthetic alternatives can potentially reduce reliance on mined materials.
Battery Disposal and Recycling
The life cycle of a battery does not end once it serves its purpose in a vehicle. Proper disposal and recycling are vital to mitigate environmental harm and reclaim valuable materials:
- Recycling Processes: Each battery type requires specific recycling processes. Lithium-ion batteries, commonly used in EVs, hold significant recyclable components, including lithium and cobalt. Effective recycling methods can reclaim up to 95% of these materials.
- Environmental Benefits: Recycling batteries helps reduce the demand for newly sourced materials, lessening the environmental impact associated with mining. Furthermore, it decreases the volume of waste that ends up in landfills, protecting ecosystems and maintaining local biodiversity.
- Legislative Framework: Governments around the world are beginning to implement regulations and incentives aimed at promoting recycling practices. With the adoption of circular economy principles, the focus is not only on production efficiency but also on material recovery and reuse.
"The recycling of electric vehicle batteries is not just an environmental necessity; it is an opportunity for responsible innovation in an evolving industry."
By addressing raw material sourcing and implementing effective battery disposal and recycling methods, the electric vehicle sector can take significant strides toward minimizing its ecological footprint while fostering sustainable practices.
Future Trends in Battery Technology
The evolution of battery technology is crucial for the advancement of electric vehicles (EVs). As the demand for sustainable and efficient transportation rises, the future of batteries in electric cars takes center stage. These advancements not only aim to improve performance but also address concerns related to cost, environmental impact, and safety. Understanding these trends allows researchers, educators, and professionals to adapt to a rapidly changing landscape.
Emerging Technologies
In recent years, several emerging technologies have captured attention in the realm of electric vehicle batteries. Solid-state batteries are at the forefront of innovation. They promise higher energy density and improved safety compared to traditional lithium-ion batteries. This technology replaces the liquid electrolyte with a solid electrolyte, which reduces the risk of leakage and fire.
Additionally, lithium-sulfur batteries are gaining recognition for their potential to significantly increase energy capacity. They have the advantage of using sulfur, an abundant and inexpensive material, which could lead to lower production costs. As these technologies mature, they are likely to make electric vehicles more accessible and attractive to consumers.
"Emerging battery technologies hold the potential to redefine the electric vehicle market by improving efficiency and reducing costs."
Industry Innovations
The automotive industry is also responding to the need for enhanced battery performance through various innovations. Tesla, for instance, is working on the development of high-capacity battery cells that promise longer range and shorter charging times. Their work on a new battery architecture could facilitate faster production and contribute to wider EV adoption.
Moreover, manufacturers like General Motors are investing in research to improve the recyclability of batteries. This includes designing batteries that can be easily disassembled and reused, which addresses environmental concerns related to battery disposal. The focus on sustainability not only involves the production side but extends to lifecycle management as well.
- Key Innovations:
- High-capacity battery cells from Tesla.
- Enhanced recyclability initiatives by General Motors.
Through these innovative approaches, the automotive sector aims to propel electric vehicles into mainstream adoption by resolving historical challenges, such as range anxiety and ecological impact.
Impact of Batteries on Vehicle Design
The evolution of battery technology plays a crucial role in shaping the design of electric vehicles. Batteries are not merely a component; they are integral to a vehicle's architecture. The design decisions driven by battery attributes affect safety, performance, and efficiency of electric cars.
Weight Distribution
Weight distribution is one core aspect influenced by battery placement within a vehicle. Electric cars often have heavy batteries, which can alter a vehicle's center of gravity. A lower center of gravity can enhance stability, especially during turns. Proper placement helps maintain balanced handling, giving drivers more control and a better overall driving experience.
The majority of EV batteries are positioned low in the chassis, which is advantageous. This setup not only contributes to stability but also minimizes rollover risks. On the contrary, an improper weight distribution can lead to compromised vehicle dynamics, which can be dangerous at high speeds. Manufacturers design battery housings to ensure optimal weight distribution, catering to the need for both performance and safety.
"The correct weight distribution of batteries in electric vehicles directly correlates with their driving characteristics and overall safety."
Space Optimization
Space is another significant consideration. Electric vehicles require efficient use of space to house batteries, electric motors, and other components. Unlike traditional cars with internal combustion engines, where engines take up significant space under the hood, electric motors are more compact. This distinction allows for more flexible interior designs and better utilization of available space.
Designers are increasingly innovating ways to integrate battery packs within the vehicleβs frame, often under the floor. This practice not only maximizes cabin space but also contributes to a more spacious and comfortable passenger experience. The challenge is to create a design that protects the battery without sacrificing aesthetics or usability.
- Integration Strategies:
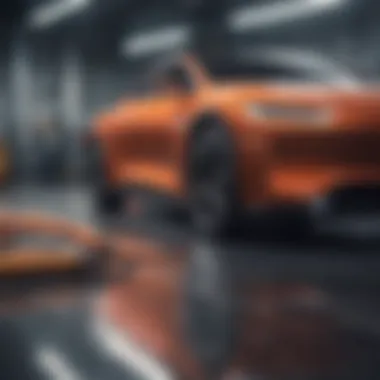
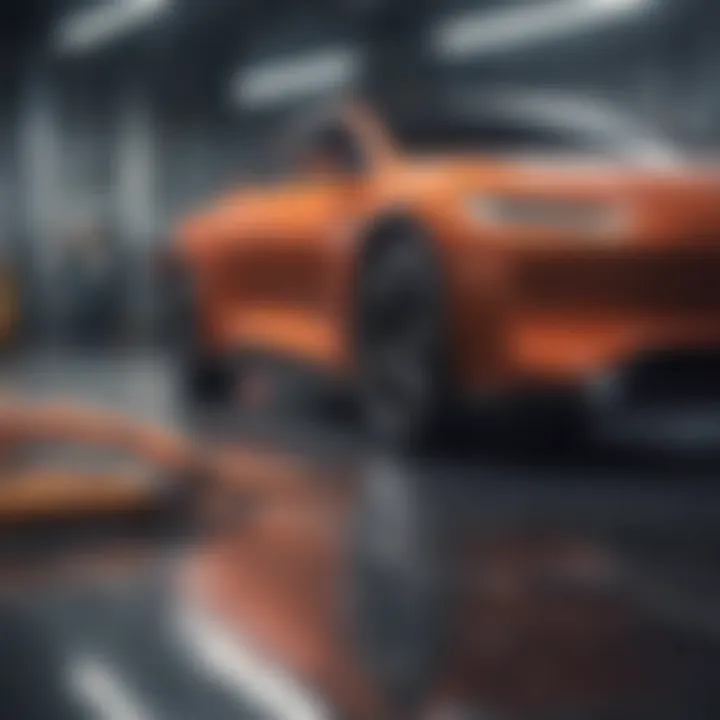
- Under-floor battery placement
- Use of modular battery designs
- Incorporation of battery packs into structural elements
Through such innovations, manufacturers enhance the vehicle's efficiency while maintaining spacious interiors, thereby appealing to environmentally conscious and practical consumers alike.
Challenges in Battery Development
The development of batteries for electric vehicles encompasses a multitude of challenges that can significantly influence the efficiency and viability of electric cars. As the need for sustainable transportation grows, understanding these challenges is essential. Each aspect of battery development, from cost efficiency to safety concerns, plays a crucial role in shaping the future of electric vehicles. \ Several factors, including rising material costs, safety standards, and technology adoption rates, must be addressed to fully realize the potential of electric vehicle batteries.
Cost Efficiency
Cost efficiency represents a vital challenge within battery development. As the electric vehicle market expands, the demand for batteries increases. However, the production costs associated with these batteries remain high. Lithium-ion batteries, while prevalent, face concerns regarding the prices of raw materials like lithium and cobalt.
Efforts to reduce costs often focus on advancements in manufacturing techniques. For instance, scaling production through economies of scale can drive prices down. Switching to alternate materials or chemistries is another avenue being explored. Companies are increasingly interested in developing batteries with abundant materials that can still meet performance metrics.
Additionally, a longer lifecycle of batteries can promote cost efficiency in the long run. It lowers the need for replacements and enhances the sustainability of electric vehicles. Innovations such as better recycling processes can also alleviate costs by reclaiming materials from old batteries.
Safety Concerns
Safety concerns are paramount in the realm of battery technology, especially in electric vehicles. Incidents involving battery failures can undermine trust in electric vehicle technology. The risk of thermal runaway in lithium-ion batteries is a significant issue. It can lead to overheating and fires if not properly managed.
To counteract these risks, manufacturers are continually investing in research and development. Battery management systems are being refined to monitor conditions and manage safety protocols. Furthermore, regulatory bodies are establishing stringent guidelines and standards to ensure battery safety throughout their lifecycle.
Improving the chemistry of batteries can also mitigate these safety risks. Solid-state batteries, with their promise of reduced flammability, are one example of how technology can address these safety concerns directly. The transition to safer, more reliable battery options is critical for the continued acceptance of electric vehicles by consumers.
The ongoing evolution of battery technology emphasizes the necessity for continuous improvement in both cost efficiency and safety. Balancing these factors is crucial for the sustainable growth of electric vehicles.
Regulatory Landscape
The regulatory landscape surrounding batteries in electric vehicles (EVs) is a critical aspect of the broader automotive and environmental context. Governments and regulatory bodies play an essential role in shaping the standards and practices of battery production, usage, and disposal. By understanding this landscape, stakeholders can navigate the complex interplay between policy, industry innovation, and environmental safeguarding.
Government Policies
Government policies are central to establishing a framework that supports the growth of electric vehicles. Many nations have set ambitious goals to reduce carbon emissions and improve air quality. These objectives often translate into specific policies that promote the adoption of electric vehicles. For instance, countries such as Norway and the Netherlands offer significant incentives for consumers to purchase EVs. Such incentives can include tax rebates, grants, or reductions in registration fees, which directly impact consumer choices and acceptance.
Moreover, government regulations also focus on the production process of batteries. Policies often target the sourcing of raw materials, ensuring that they are obtained sustainably and ethically. For example, cobalt, used in lithium-ion batteries, has raised concerns due to human rights issues in some mining practices. To combat this, regulations can mandate transparency in supply chains. These comprehensive policies thus support not only the adoption of EVs but also the development of responsible sourcing practices.
Standards and Guidelines
Standards and guidelines are critical in ensuring the safety and efficiency of electric vehicle batteries. International organizations, such as the International Organization for Standardization (ISO), work to establish consistent standards that manufacturers must adhere to. These standards encompass various aspects, including battery performance, safety testing, and environmental impact assessments.
Adherence to these guidelines helps mitigate risks associated with battery failures, which could lead to fires or other hazards. Moreover, standardization facilitates interoperability between different vehicles, charging stations, and battery types. This interoperability is crucial as it encourages infrastructure development, thereby improving charging accessibility for consumers.
In addition to safety standards, there are also environmental guidelines that dictate how batteries should be disposed of or recycled. Proper recycling processes are crucial as they minimize waste and reduce the demand for new raw materials. The circular economy approach, which emphasizes reusing materials and reducing waste, is increasingly becoming a focus of regulatory frameworks worldwide.
Overall, a clear regulatory landscape not only drives market growth but also promotes innovation, responsible sourcing, and environmental stewardship in the electric vehicle sector.
Ending and Future Outlook
The conclusion and future outlook regarding batteries in electric vehicles encapsulates the crucial themes explored throughout this article. As the automotive industry transitions toward electric mobility, the role of battery technology becomes increasingly significant. The potency of batteries influences not only vehicle performance but also the sustainability of this energy transition. Thus, understanding the future trajectory of battery technology is vital for stakeholders ranging from manufacturers to consumers.
Key Takeaways
In summarizing the insights gathered, several key takeaways emerge:
- Battery Types: Lithium-ion batteries remain dominant in the current market. However, emerging technologies like solid-state batteries may redefine energy efficiency and safety in the near future.
- Performance Metrics: Factors like energy density and charge time are critical. Consumers expect rapid charging and long-range capabilities, which drives innovation.
- Environmental Impact: The green credentials of electric vehicles are intertwined with sustainable battery production and recycling processes.
- Safety and Cost: Concerns regarding safety standards must be addressed alongside efforts to reduce manufacturing costs.
By recognizing these key points, stakeholders can better navigate the complex landscape of electric vehicle batteries.
Long-Term Projections
Looking ahead, the trajectory of battery technology in electric vehicles is marked by several projected advancements:
- Increased Energy Density: Future batteries are expected to offer higher energy density. This translates to longer distances between charges, an essential factor for widespread adoption.
- Faster Charging Times: Advancements in battery chemistry and construction may lead to dramatic reductions in charging times. Research aims for charging within minutes rather than hours.
- Circular Economy: Focus will likely shift toward circular economy practices, enhancing recycling technologies. This will impact not only environmental sustainability but also cost efficiencies.
- Safety Measures: Further development in safety protocols and materials will enhance the reliability of batteries, addressing current concerns.
- Regulatory Changes: Anticipated changes in government policies will guide manufacturers on acceptable battery standards, promoting environmentally-friendly practices.
These projections serve as a reminder of the potential trajectory of battery technology, influencing both the automotive industry and the environment.