ATP in Biology: The Core of Cellular Energy Dynamics
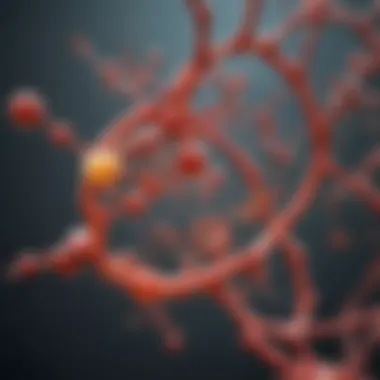
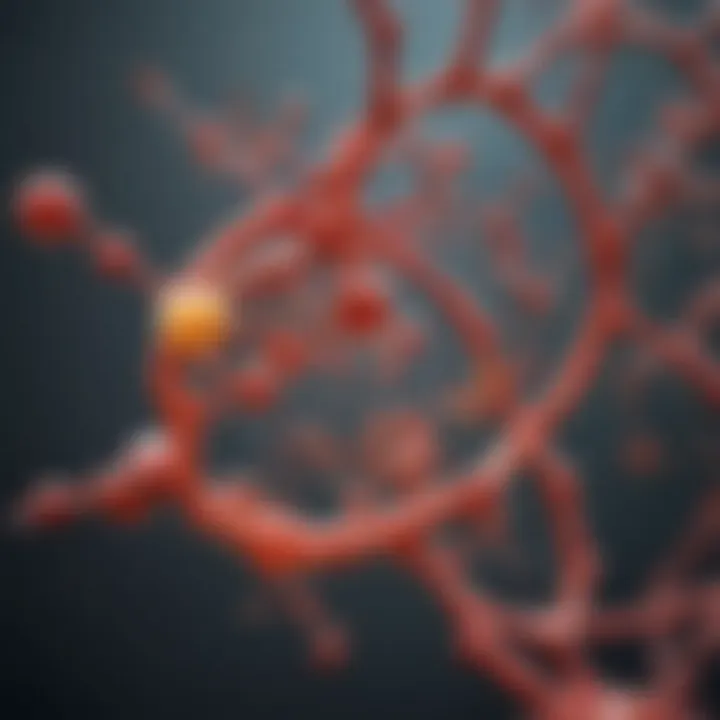
Intro
Adenosine triphosphate, or ATP, is essential in biology. It serves as the primary energy currency of all living organisms. ATP is indispensable for various cellular processes, including metabolism, signaling, and maintaining cellular homeostasis. The detailed understanding of ATP's structure and function provides insights into its numerous roles in biological systems. This article will examine ATPโs significance, exploring its biosynthesis, metabolic roles, and how it interplays with other biomolecules.
Research Overview
Summary of Key Findings
ATP is a molecule composed of adenosine and three phosphate groups. The bonds between these phosphate groups hold significant energy. When these bonds break through hydrolysis, energy becomes available for cellular processes. Key findings indicate that ATP is involved in:
- Energy transfer during metabolic reactions
- Regulation of enzymatic activity
- Signal transduction in cells
- Synthesis of nucleic acids and proteins
This underscores the central role of ATP by highlighting its multifaceted functions in various metabolic pathways.
Research Objectives and Hypotheses
The objectives of this research include investigating:
- The structural characteristics of ATP and how they contribute to its energy transfer capabilities.
- The biosynthetic pathways of ATP, specifically in aerobic and anaerobic conditions.
- The impact of ATP on different metabolic pathways.
The hypothesis states that variations in ATP concentrations can significantly influence metabolic rates and enzymatic activities in cells.
Methodology
Study Design and Approach
The design of this study involves a thorough literature review and analysis of experimental studies on ATP. Various approaches, such as structural analysis and thermodynamic assessments, are used to understand ATP's roles.
Data Collection Techniques
Data has been collected from various reputable sources. Techniques include:
- Comprehensive reviews from scientific journals
- Meta-analyses of existing research studies
- Experimental data from laboratory studies examining ATP function and energy dynamics
"ATP is not just an energy molecule; it is the very lifeblood of cellular activities across all forms of life."
Through these methodologies, the article aims to present a well-rounded discussion on ATP's significance in cellular biology.
Prelims to Adenosine Triphosphate
Adenosine triphosphate, commonly known as ATP, is essential in the study of cellular energy and metabolism. Its significance transcends mere chemical properties; ATP is central to almost all biological processes. Understanding its role is crucial for anyone delving into cellular biology, biochemistry, or related fields. ATP serves as the primary energy currency of the cell. It is involved in critical functions such as muscle contraction, nerve impulse propagation, and biochemical synthesis. Therefore, the exploration of ATP is key to grasping the fundamentals of life itself.
ATP consists of three main components: adenine, ribose, and a chain of three phosphate groups. Each component contributes to the overall function of ATP in energy transfer and metabolism. The ability of ATP to release energy during hydrolysis underpins its conceptualization as an energy currency. This energy is harnessed by various cellular processes to fuel actions within the cell. With the varied roles it plays, ATP is a cornerstone in the grand scheme of biological function.
Further, ATP's discovery and understanding highlight a historical evolution that reflects scientific progress. Tracing the journey of ATP from its discovery to its varied applications in modern science reveals much about the development of biological research. Understanding this historical context provides a deeper appreciation of ATP's significance.
Understanding ATP as Energy Currency
ATP acts as the primary energy carrier in cells. Its structure allows for easy transfer of energy, which is vital for the survival of organisms. When ATP is hydrolyzed, it loses one of its phosphate groups, converting to adenosine diphosphate (ADP) and releasing energy. This energy is what drives various cellular activities such as muscle contraction, transport of molecules across membranes, and biochemical transformations required for metabolism.
The cycle of ATP synthesis and hydrolysis is ongoing within the cell. Each time ATP is used, it needs to be replenished through metabolic pathways like oxidative phosphorylation or substrate-level phosphorylation. This cycle is efficient and ensures that the cell has a constant supply of energy, maintaining homeostasis.
Historical Context of ATP Discovery
The discovery of ATP can be traced back to the early 20th century, specifically to scientists like Karl Lohmann and his colleagues, who first isolated ATP in 1929. Their work laid the groundwork for understanding the respiration processes in cells. Later, in 1941, Dr. Otto Warburg demonstrated the role of ATP in cellular respiration, solidifying its importance within the metabolic processes of living organisms.
As research progressed, ATP emerged as not just a biochemical curiosity but as a pivotal factor in understanding various physiological functions. Each advancement in ATP research has spurred further exploration, leading to new insights about energy transfer mechanisms in different biological contexts. This history of ATP discovery highlights the interconnectivity between scientific inquiry and advancements in our understanding of life processes.
Chemical Structure of ATP
The chemical structure of ATP is crucial in understanding its function as the primary energy currency within cells. ATP, or adenosine triphosphate, consists of three main components: ribose sugar, phosphate groups, and adenine base. Each of these elements plays a significant role in how ATP stores and releases energy during cellular processes.
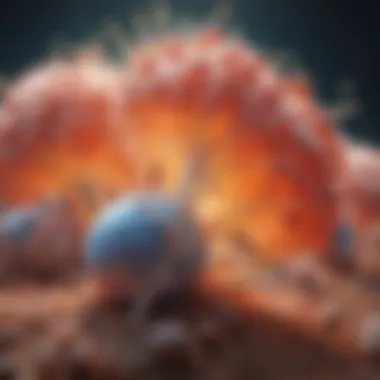
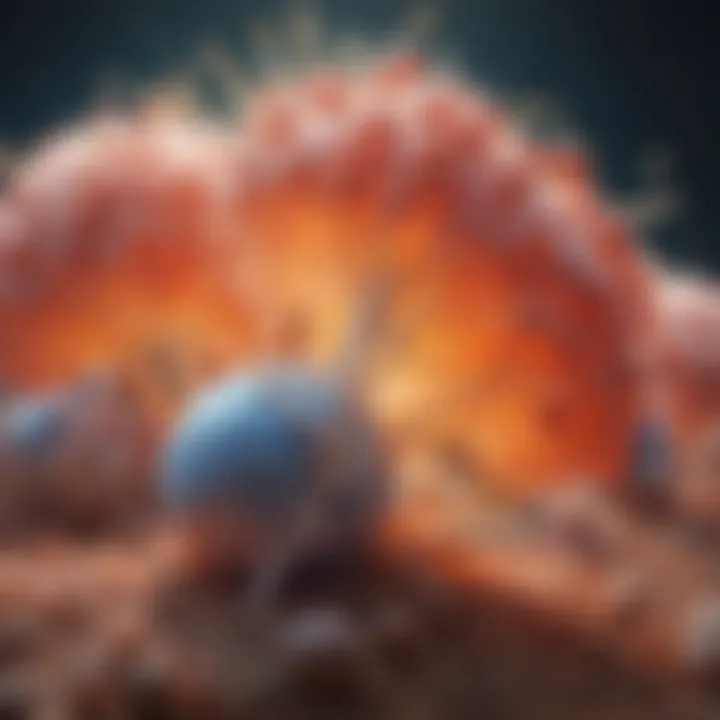
Components of ATP
Ribose Sugar
Ribose sugar is a five-carbon sugar that forms the backbone of the ATP molecule. It contributes to ATP's overall structure and stability. The key characteristic of ribose is its ability to bond with other molecules, allowing it to act as a scaffold for both the adenine base and the phosphate groups. This particular structure makes ribose a popular choice in nucleotides, enhancing the molecule's ability to participate in various biochemical reactions. A unique feature of ribose is its hydroxyl groups (-OH), which influence the reactivity of the molecule. This leads to both advantages, in terms of energy transfer, and disadvantages, such as susceptibility to hydrolysis.
Phosphate Groups
Phosphate groups are integral to ATP's functionality. Each ATP molecule contains three phosphate groups, linked by high-energy bonds. The most significant aspect of these phosphate groups is their ability to release energy upon hydrolysis. When one phosphate group is removed, ATP is converted to ADP (adenosine diphosphate), releasing energy that powers cellular processes. This property of phosphate groups makes them beneficial for ATP, as they play a crucial role in energy transfer. Moreover, the unique feature of these groups is their negative charge, which creates repulsion between them, contributing to the energy stored in the bonds. However, this also means ATP is relatively unstable in high concentrations, which is a disadvantage in certain contexts.
Adenine Base
The adenine base is a nitrogenous compound that completes the structure of ATP. It is essential for the identification of ATP as a nucleotide. The key characteristic of adenine is its ability to form hydrogen bonds with other nucleotides, facilitating cellular communication and regulation. This makes adenine an important component in the overall structure of ATP. A unique feature of adenine is its involvement in various biochemical pathways, such as DNA and RNA synthesis. This interconnectedness is an advantage, as it reflects the multifunctional role of ATP in cellular functions and energy exchange.
Stability and Reactivity of ATP
ATP is known for being both stable and reactive, which is crucial for its role in cellular energy metabolism. The molecule maintains stability under physiological conditions, allowing it to exist in a dynamic equilibrium within the cell. However, its reactivity is what allows it to effectively transfer energy. The configuration of the phosphate groups contributes to this delicate balance. ATP can easily hydrolyze to ADP and inorganic phosphate in the presence of water, liberating energy essential for various cellular activities. This dual nature positions ATP as the cornerstone of cellular energy management.
ATP Synthesis Mechanisms
The process of ATP synthesis is essential for maintaining the energy balance within cells. These mechanisms ensure that ATP is continually produced to meet the cellular demands for energy. Understanding how ATP is synthesized is crucial for grasping its role in metabolic pathways, energy transfer, and overall cellular functionality.
Oxidative Phosphorylation
Electron Transport Chain
The Electron Transport Chain (ETC) is a series of complexes located in the inner mitochondrial membrane. It plays a vital role in the process of oxidative phosphorylation by facilitating the transfer of electrons derived from NADH and FAD, which are produced during earlier metabolic processes like glycolysis and the Krebs cycle. The key characteristic of the ETC is its ability to generate a proton gradient across the mitochondrial membrane. This gradient is what drives ATP synthesis via ATP synthase.
The ETC is a beneficial choice for ATP synthesis due to its high efficiency in producing ATP. Each transport complex contributes to energy conservation and produces water as a non-toxic byproduct, which is crucial for cellular homeostasis. However, the system does have vulnerabilities; for instance, it is sensitive to inhibitors such as cyanide, which can severely disrupt ATP production.
Chemiosmosis
Chemiosmosis refers to the movement of protons across a membrane, which occurs due to the established proton gradient generated by the ETC. The unique feature of chemiosmosis lies in its driving force for ATP production. When protons flow back into the mitochondrial matrix through ATP synthase, this process catalyzes the conversion of ADP and inorganic phosphate into ATP.
This mechanism is widely considered a key component of cellular respiration because of its effectiveness in synthesizing large quantities of ATP. One of the main advantages of chemiosmosis is that it links energy metabolism and ATP production, emphasizing its importance in cellular energy supply. However, dysregulation of this process can lead to issues such as oxidative stress and cell damage, which highlights the need for tight regulation in cellular environments.
Substrate-Level Phosphorylation
Glycolysis Process
Glycolysis marks the initial stage of glucose metabolism and occurs in the cytoplasm. This process is crucial, as it breaks down glucose into pyruvate, yielding a net gain of two ATP molecules. The key aspect of glycolysis is its anaerobic nature; it does not require oxygen, making it a vital energy source in hypoxic conditions. It is a well-known mechanism due to its rapid ATP production.
One unique feature of glycolysis is that it occurs in several steps, allowing the cell to capture and utilize energy efficiently. While glycolysis is advantageous in quickly yielding ATP, its limitation lies in the lower number of ATP produced compared to oxidative phosphorylation.
Krebs Cycle Contribution
The Krebs cycle, also known as the citric acid cycle, plays a significant role in energy metabolism within the mitochondrial matrix. It generates electron carriers such as NADH and FAD, which feed into the Electron Transport Chain. The key characteristic of the Krebs cycle is that it produces both ATP and various metabolites used in biosynthesis and energy generation.
This cycle is beneficial for ATP production because it fosters the complete oxidation of nutrients, maximizing energy yield. Its unique feature is its integration into broader metabolic pathways, allowing cooperation among different cellular processes. Nevertheless, unlike glycolysis, it requires oxygen and is thus less adaptable in anaerobic conditions.
Photophosphorylation in Photosynthesis
Photophosphorylation takes place in the chloroplasts of plant cells during photosynthesis. This process involves the conversion of light energy into chemical energy, culminating in the production of ATP and NADPH. The key characteristic of photophosphorylation is its reliance on light-driven electron transport, similar to oxidative phosphorylation. This connection underscores the fundamental importance of ATP across different biological systems. The unique feature is its role in capturing solar energy, which is essential for all life on Earth.
Photophosphorylation has significantly impacted ecological and biological systems, ensuring a steady supply of energy at the foundation of food webs. However, it depends on environmental conditions such as light intensity, presenting a challenge for ATP production in fluctuating conditions.
The synthesis of ATP is not just a biochemical reaction; it stands at the core of life's energy transactions, interlinking various metabolic pathways critical for survival.
Role of ATP in Cellular Metabolism
Adenosine triphosphate (ATP) is central to many cellular processes, acting as a dynamic energy source that facilitates vital functions within the cell. The significance of ATP in metabolism cannot be overstated; it effectively couples energy release with energy use across numerous biochemical pathways. Understanding how ATP operates in cellular metabolism reveals insights into energy balance, cellular efficiency, and overall organismal health.
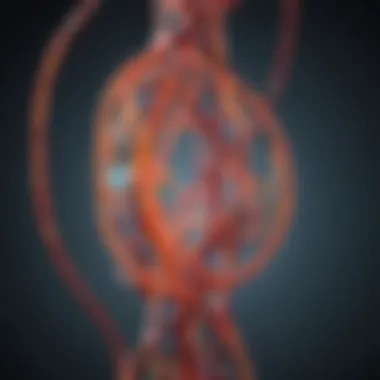
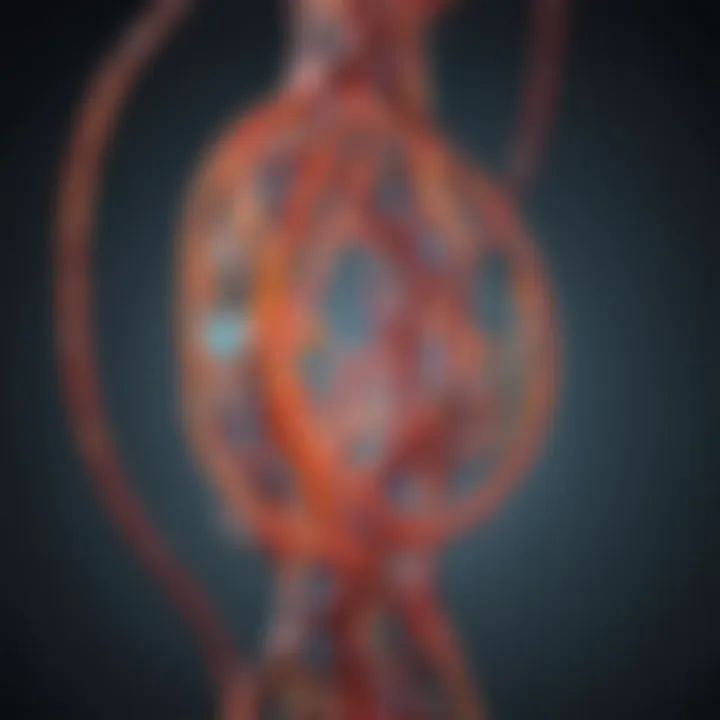
ATP Hydrolysis and Energy Release
ATP hydrolysis plays a crucial role in energy release for cellular activities. The process involves the breakdown of ATP into adenosine diphosphate (ADP) and an inorganic phosphate (Pi). This reaction is highly exergonic, meaning it releases a significant amount of energy which is used by the cell for various functions. The breakdown occurs through enzyme-catalyzed reactions, where the enzyme ATPase facilitates the release of the terminal phosphate.
Key aspects of ATP hydrolysis include:
- Energy Yield: Each molecule of ATP releases approximately 7.3 kcal/mol of energy upon hydrolysis. This energy is fundamental for critical cellular processes such as muscle contraction, ion transport, and biosynthesis.
- Regenerative Cycle: ATP is constantly regenerated from ADP and Pi through cellular respiration and photophosphorylation, ensuring a continuing supply of energy to meet cellular demands.
By allowing cells to harness energy swiftly and effectively, ATP hydrolysis acts as an indispensable mechanism that supports metabolism.
ATP and Enzyme Function
ATP is often referred to as the "molecular switch" that regulates enzyme activity. It binds to various enzymes, influencing their conformation and activity. The binding of ATP can activate or inhibit enzymes, thereby modulating metabolic pathways according to cellular needs.
Crucial points regarding ATP's role in enzyme function include:
- Allosteric Regulation: ATP acts as an allosteric regulator for many enzymes. Changes in ATP concentration can affect how aggressively an enzyme catalyzes a reaction. High ATP levels usually indicate energy abundance, promoting catabolic pathways. Conversely, low levels signal a need for energy production.
- Phosphorylation: ATP provides a phosphate group that can phosphorylate other molecules, a modification that can activate or deactivate enzymes. Phosphorylation cascades are critical in signaling pathways, influencing many processes, from metabolism to cell division.
In essence, ATP serves as more than just an energy currency; it is a crucial participant in the control of enzyme dynamics and cellular responses.
ATP in Biosynthetic Reactions
Biosynthesis refers to the metabolic pathways that build complex molecules from simple precursors. ATP is vital for many biosynthetic processes, providing not only energy but also phosphate groups necessary for the synthesis of nucleic acids, proteins, and lipids.
Some important roles of ATP in biosynthetic reactions include:
- Nucleic Acid Synthesis: ATP is one of the four nucleotides needed for the synthesis of RNA and DNA. In nucleotide polymerization, ATP contributes both energy and the adenine residue, linking nucleotides together energetically and chemically.
- Protein Synthesis: ATP is involved in the initiation and elongation phases of protein synthesis. It activates amino acids for incorporation into polypeptides, ensuring that proteins are formed efficiently.
- Lipid Formation: ATP drives the synthesis of lipids through fatty acid activation, allowing for the construction of membrane structures essential for cellular organization.
Overall, ATP's involvement in biosynthesis is paramount, as it supplies the necessary energy and building blocks for the formation of biomolecules essential for life.
ATP's Role in Cellular Signaling
Adenosine triphosphate (ATP) extends its importance beyond being merely an energy molecule, playing a crucial role in cellular signaling. This aspect of ATP encompasses various physiological and pathological processes, facilitating communication between cells. Signaling pathways that involve ATP influence processes such as inflammation, immune responses, and neurotransmission, indicating the versatility of this molecule.
ATP acts as a signaling molecule by participating in several intricate pathways that provide crucial information to cells to respond appropriately to their environment. This dynamic role shows how ATP not only powers cellular processes but also coordinates them through signaling mechanisms.
ATP as a Signaling Molecule
Pathways Involving ATP
Pathways that involve ATP are essential for cell communication and regulation. ATP is released from cells and can activate purinergic receptors on the surface of neighboring cells. The primary characteristic of these pathways is their ability to mediate rapid responses to external stimuli. This makes them a beneficial choice for connecting physiological states to cellular behavior.
One unique feature of these pathways is their ability to propagate signals quickly due to the widespread distribution of purinergic receptors. The presence of ATP allows for a fast relay of information, which is vital for maintaining homeostasis. However, there are disadvantages as well, such as potential desensitization of receptors with constant stimulation, leading to decreased cell responsiveness over time.
Receptor Interaction
Receptor interactions involving ATP primarily concern purinergic receptors, which are integral to cellular signaling. These receptors enable cells to sense ATP levels in their vicinity, influencing diverse functions like neurotransmitter release and inflammation. The key characteristic of these interactions is their ability to initiate cascading biochemical events, effectively amplifying the cellular response to ATP. This makes them a popular choice for understanding signal transduction in various biological contexts.
A notable unique feature of receptor interaction is the concept of allosteric modulation. This means that ATP can modify the activity of certain receptors indirectly, enhancing or inhibiting their function based on the cellular context. While this adds versatility to cellular responses, it can also complicate the interpretation of signaling pathways, as the effects of ATP can vary significantly depending on the surrounding environment.
Extracellular ATP and Cell Communication
Extracellular ATP plays a pivotal role in facilitating communication between cells. When released from damaged or activated cells, ATP serves as a danger signal, alerting nearby cells to potential harm. This signaling can influence a broad spectrum of cell types, affecting processes from immune activation to tissue regeneration.
ATP's role in cell communication highlights its importance in both healthy and diseased states. For instance, in chronic inflammation, excessive ATP release can lead to continuous immune activation, which may exacerbate tissue damage. Understanding how extracellular ATP functions in different contexts is vital for exploring therapeutic avenues in various diseases.
ATP is not just an energy currency; it is a versatile signaling molecule crucial for maintaining cellular communication and responses.
ATP in Pathophysiology
The role of ATP in biological systems extends beyond its fundamental function as an energy currency. In pathophysiological contexts, ATP is integral to disease processes. Its levels can dictate cellular health and functionality, and deviations can signify or even contribute to numerous conditions. Understanding these relationships can enhance our comprehension of disease mechanisms and potentially lead to new therapeutic approaches.
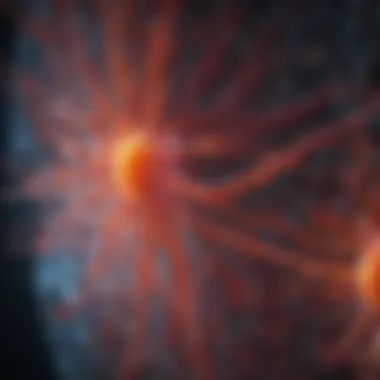
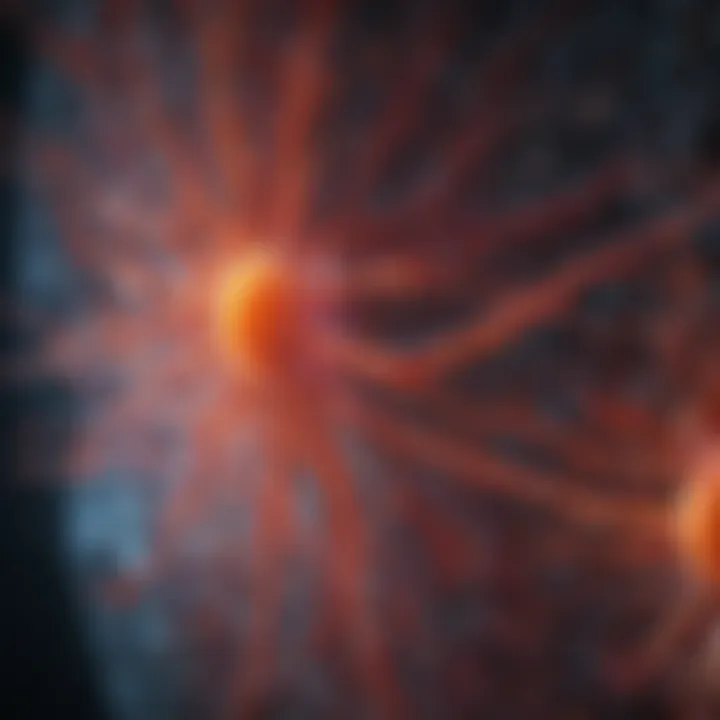
Role of ATP in Disease Mechanisms
ATP is crucial in several cellular pathways. Disruption in ATP synthesis can lead to impaired cellular functions.
- Some diseases like
- Diabetes
- Neurodegenerative disorders
- Cancer
All exhibit altered ATP metabolism. For instance, in ischemic conditions, oxygen deprivation leads to decreased ATP production, resulting in cell death due to failure in maintaining ion gradients.
Furthermore, ATP's hydrolysis is central to apoptosis, or programmed cell death. A lack of ATP prevents cells from undergoing this critical process, contributing to tumor growth and survival in harmful environments. In contrast, excessive ATP release can act as a danger signal triggering inflammation. The duality of ATP highlights its complex role in both promoting and combating disease states.
Therapeutic Targets Involving ATP
Exploration of ATP's role in diseases offers potential therapeutic avenues. Targeting ATP metabolism can influence various treatments.
- In cancer therapy, agents that disrupt ATP availability can hinder cancer cell proliferation.
- Treatments that modulate ATP levels in neurodegenerative diseases may offer neuroprotective effects.
- Developing inhibitors that target ATP-sensitive potassium channels can improve heart function in certain cardiovascular conditions.
Recent advancements in drug design focus on ATP-related pathways, highlighting the need for continued research. By targeting ATP in specific cellular processes, we can tailor treatments for optimal patient outcomes.
The intricate balance of ATP in health and disease underscores its significance.
Thus, further investigations into ATP's multifaceted roles can lead to novel interventions, improving our understanding of pathophysiology and enhancing patient care.
Research Frontiers in ATP Studies
Research on adenosine triphosphate (ATP) continues to evolve, revealing new insights about its multifaceted role in cellular functions. Understanding ATP extends beyond its classical definition as the energy currency of the cell; it delves into its involvement in signaling pathways, metabolic processes, and disease mechanisms. Recent studies suggest that investigating the nuanced behaviors of ATP can lead to breakthroughs in various fields, including medicine, agriculture, and biotechnology.
Innovative Techniques in ATP Analysis
Novel techniques have emerged to analyze ATP with greater precision, contributing significantly to various scientific fields. Technologies such as mass spectrometry, high-performance liquid chromatography, and nuclear magnetic resonance spectroscopy enable detailed investigations into ATP's dynamics in living cells.
- Mass Spectrometry provides high sensitivity and specificity for ATP detection. This method allows researchers to analyze changes in ATP levels in response to cellular stress or environmental stimuli.
- High-Performance Liquid Chromatography (HPLC) can separate ATP from other nucleotides, offering a clearer view of ATP concentration within different cellular compartments.
- Nuclear Magnetic Resonance (NMR) Spectroscopy allows scientists to track ATP interactions with proteins or other molecules, giving insights into its role in cellular signaling.
"Understanding ATP's intricate behaviors requires advanced analytical techniques that provide clarity and precision."
These innovative methods help scientists accurately quantify ATP levels, observe real-time changes, and understand ATP's role in various biological processes. As tools improve, so does the potential to uncover ATP's hidden mechanisms in health and disease.
Future Directions for ATP Research
Future research on ATP aims to address several key areas that can enhance our understanding of biology and medicine.
- Drug Development: Research is exploring how ATP and its derivatives can serve as potential drug candidates or targets for new therapies.
- Cellular Communication: Investigating how ATP functions as a signaling molecule in intercellular communication will likely reveal novel pathways involved in health and disease.
- Energy Metabolism Disorders: Understanding ATP's role in energy metabolism may lead to new treatments for diseases associated with dysfunctional energy production, like diabetes or metabolic syndrome.
- Synthetic Biology: The integration of ATP in synthetic biological systems could improve engineering biological pathways for industrial applications, including biofuels and pharmaceuticals.
The ongoing exploration in ATP studies is poised to not just enhance our understanding of this essential molecule, but also to pave the way for innovative approaches to complex health challenges that humanity faces today.
The End
The conclusion serves as a critical synthesis of key insights from the article, emphasizing the multifaceted role of ATP in biological systems. Understanding the importance of adenosine triphosphate encompasses not just its function as an energy currency, but also its significance in cellular signaling and metabolism. In summary, ATP is at the heart of energetic transactions in living organisms, facilitating processes such as muscle contraction, biosynthesis of molecules, and active transport across membranes.
Summary of ATP's Biological Significance
ATP's biological significance is highlighted by its central role in metabolic pathways. This molecule bridges catabolic and anabolic reactions within cells, ensuring that energy flows efficiently through various biochemical processes.
- ATP hydrolysis provides energy required for physiological functions.
- It participates in enzyme catalysis, impacting reaction rates and pathways.
- ATP involvement in signaling events underlines its role in communication between cells.
Overall, ATP is indispensable for the survival and functioning of all living things, demonstrating its universal importance in both simple and complex organisms.
The Ongoing Importance of ATP Research
The study of ATP remains a dynamic area of scientific investigation. Continued research provides novel insights into how ATP contributes to health and disease.
Researchers explore:
- New techniques for ATP measurement, enhancing our understanding of its dynamics in real-time cellular environments.
- The connection between ATP levels and various diseases, including cancer and metabolic disorders.
- Potential therapeutic approaches targeting ATP pathways for disease treatment and prevention.
As our knowledge of this critical molecule expands, the implications for medicine and biology grow, confirming ATP's enduring relevance in life sciences.