Unraveling Dark Matter: A Cosmic Exploration
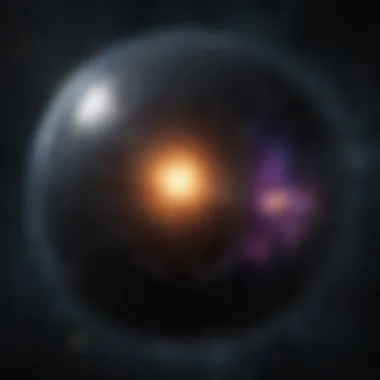
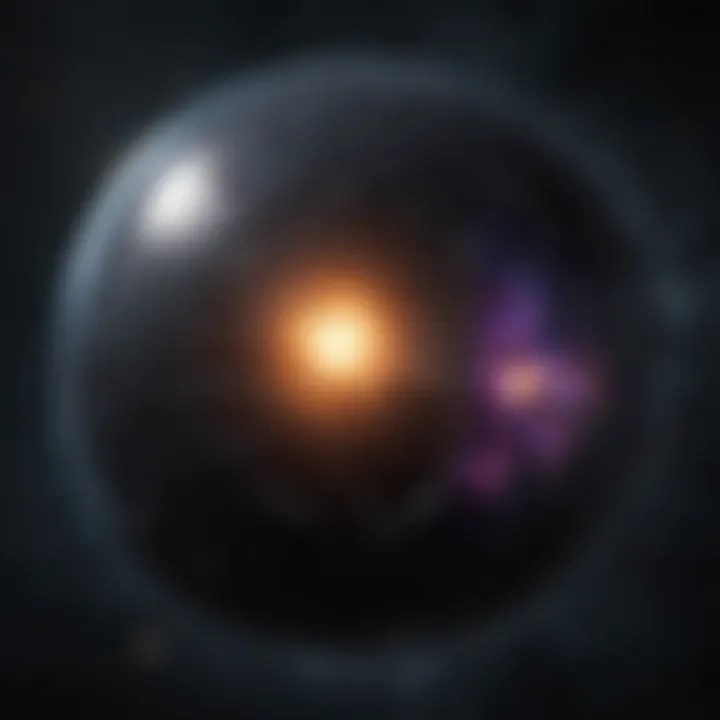
Intro
The quest for dark matter is one of the most compelling challenges in modern astrophysics. Dark matter is thought to account for approximately 27% of the universe’s mass-energy content, yet it remains invisible to current detection methods. This paradox motivates ongoing research, as the implications of dark matter touch upon fundamental aspects of cosmology, including the evolution and structure of the universe.
Research into dark matter not only seeks to uncover this elusive substance but also aims to enhance our understanding of the forces shaping cosmic structures. As we traverse through this complex journey, the pursuit of dark matter raises questions that challenge existing theories and methodologies, compelling researchers to explore new frontiers in physics.
Intro to Dark Matter
Dark matter is one of the most intriguing subjects in contemporary astrophysics. It represents a significant part of the universe's mass yet remains largely elusive. Understanding dark matter is essential for grasping various cosmic phenomena, from galaxy formation to large-scale structure. This section aims to clarify the concept of dark matter, trace its historical discoveries, and highlight its critical importance in cosmology.
Defining Dark Matter
Defining dark matter involves more than just identifying an unknown substance. It refers to a form of matter that does not emit, absorb, or reflect light, making it virtually undetectable through traditional astronomical observations. Its presence is inferred through gravitational effects on visible matter, radiating energy, and the structure of the universe itself. Researchers have estimated that dark matter constituted about 27% of the universe's total mass-energy content, making it a focal point for understanding cosmic dynamics. Clarity in its definition encourages precise discussions regarding its role and properties.
Historical Context
The journey to uncover dark matter began in the early 20th century. Fritz Zwicky first suggested its existence in 1933 while studying the Coma galaxy cluster. Zwicky observed that visible galaxies’ mass could not account for the cluster's gravitational binding. He proposed the idea of an unseen mass affecting the cluster's dynamics. Over subsequent decades, evidence in favor of dark matter accumulated, with significant contributions from studies of galaxy rotation curves and gravitational lensing effects. Historical milestones underscore how scientific consensus evolved around the idea and importance of dark matter.
Importance in Cosmology
Dark matter plays a pivotal role in cosmological models. It influences the formation and behavior of galaxies and galaxy clusters, enhancing our understanding of the universe's evolution. Theories surrounding the expansion of the universe increasingly rely on models that incorporate dark matter. Without it, the observed large-scale structure of the universe and the cosmic microwave background radiation would not be comprehensible. Thus, dark matter is not merely a curiosity; it is a cornerstone for modern cosmology, shaping our interpretation of the universe.
"Understanding dark matter is crucial for explaining the universe’s composition and fate."
Through these insights, readers can appreciate the significance of dark matter as both a mystery and an essential component of our cosmos. Before further exploring theoretical models and breaking new ground in detection methods, one must recognize dark matter’s foundational role in astrophysics.
Theoretical Framework
The theoretical framework holds immense significance in understanding dark matter. It serves as the conceptual foundation upon which researchers build their hypotheses and experiments. By exploring the underlying principles of cosmology, scientists can better grasp the role of dark matter in the universe. This framework addresses essential questions about the nature of dark matter and its interactions with ordinary matter.
Standard Model of Cosmology
The Standard Model of Cosmology integrates our knowledge of the universe's expansion and structure. Established theories like the Big Bang and cosmic inflation are vital elements. They provide a backdrop for discussing dark matter's effect on cosmic evolution. Dark matter facilitates an accurate explanation for observed structures, such as galaxy rotation curves. Without this model, it would be challenging to account for the universe we observe today.
Dark Matter Candidates
Dark matter candidates are theoretical particles that could explain the missing mass in the universe. Understanding these candidates enhances our search for dark matter, presenting viable solutions to complex problems in cosmology. Key candidates include Weakly Interacting Massive Particles, axioms and sterile neutrinos, and massive primordial black holes. Each category offers distinct features and merits.
Weakly Interacting Massive Particles (WIMPs)
WIMPs are prime candidates in the search for dark matter. Their weak interaction with normal matter sets them apart from other particles. This characteristic makes WIMPs an attractive choice because they can be detected indirectly through their interactions with regular particles. A notable feature of WIMPs is their predicted mass range, which aligns with cosmological observations. However, they also present challenges; for instance, their low interaction rates create difficulties in detection. Nevertheless, WIMPs remain a central topic in dark matter discussions.
Axioms and Sterile Neutrinos
Axioms and sterile neutrinos offer insights into the possible composition of dark matter. They are lightweight and proposed to have negligible interactions with ordinary matter. This unusual property makes them appealing for dark matter research. Sterile neutrinos, in particular, may address anomalies in astrophysical observations. However, their detection remains a substantial challenge due to their elusive nature. Despite potential complications, these candidates still play a critical role in the ongoing quest for understanding dark matter.
Massive Primordial Black Holes
Massive primordial black holes propose an alternative explanation for dark matter's existence. Generated during the early universe, they can account for the observed gravitational effects attributed to dark matter. A key characteristic of these black holes is their diverse formation scenarios. This diversity leads to a variety of potential masses. While they potentially explain some unexplained phenomena, they also raise questions about their longevity and formation mechanisms. Their discussion provides a broader perspective on dark matter candidates.
The Role of Dark Energy
Dark energy is a critical factor in cosmology, influencing the universe's accelerating expansion. While less directly related to dark matter, understanding dark energy can offer insights into cosmic structure and evolution. The relationship between dark matter and dark energy remains a focal point of current research. Investigating how they interact may help uncover the mysteries governing the cosmos. The interplay between the two is vital for a holistic comprehension of gravitational phenomena.
Detection Methods
Detection methods for dark matter are fundamental to our understanding of its nature and properties. As dark matter does not emit or absorb light, traditional observational techniques are ineffective. Therefore, scientists utilize a range of specialized methodologies to detect and study dark matter. These methods can be classified into three primary categories: direct detection techniques, indirect detection techniques, and collider experiments. Each category offers different insights and faces unique challenges. This section will detail these detection techniques and their significance in unlocking the mysteries surrounding dark matter.
Direct Detection Techniques
Direct detection techniques aim to observe dark matter particles as they interact with ordinary matter. This is essential for confirming the existence of dark matter and providing insights into its characteristics.
Underground Detectors
Underground detectors play a critical role in direct detection of dark matter. Their main advantage is that they are placed deep underground to shield them from cosmic rays and other background radiation that can create noise and interfere with measurements. A key characteristic of underground detectors is their ability to detect minute energy deposits from dark matter interactions. For example, the LUX-ZEPLIN experiment aims to reduce noise and maximize the likelihood of detecting weakly interacting massive particles (WIMPs).
Unique features of underground detectors include the use of large volumes of highly purified materials, such as liquid argon or xenon, which further minimizes interference. However, these experiments often require substantial investments in infrastructure and resources. The challenge lies in the extremely low interaction rates expected from dark matter, making it a time-consuming and resource-intensive process.
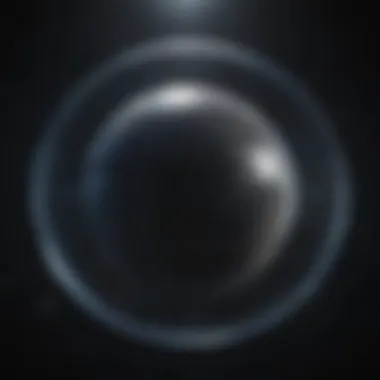
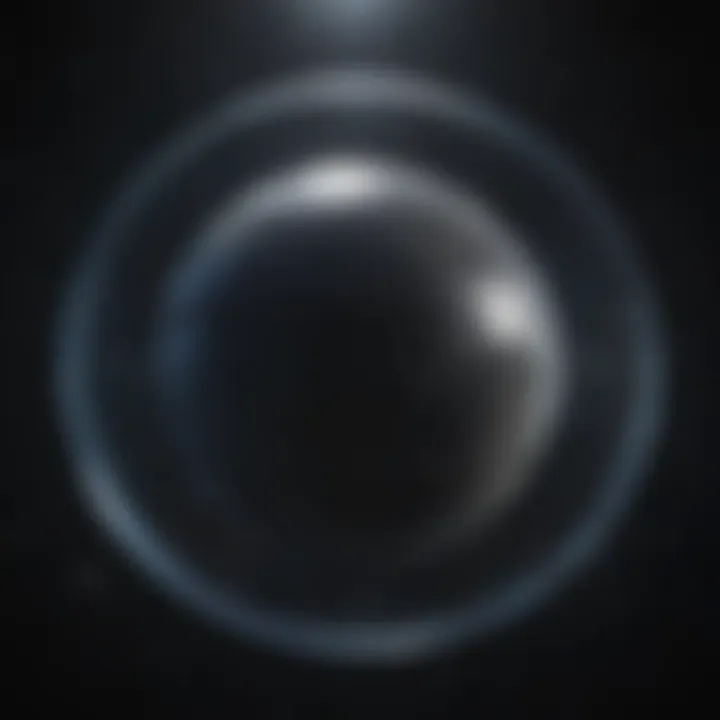
Liquid Noble Gas Detectors
Liquid noble gas detectors are an innovative approach within direct detection methods. This technology utilizes noble gases, such as xenon or argon, in liquid form to identify potential dark matter interactions. The primary characteristic of these detectors is their high sensitivity to low-energy events, which is crucial in the search for dark matter. This method is beneficial because liquid noble gas detectors can potentially achieve very low thresholds for energy detection.
One unique feature of liquid noble gas detectors is their dual-phase technology, where both liquid and gas phases can be employed to amplify the signals from potential dark matter interactions. While highly sensitive, these detectors also face challenges, as they require cryogenic temperatures and must maintain purity to avoid contamination that could mask signals from dark matter.
Indirect Detection Techniques
Indirect detection techniques seek to infer the presence of dark matter through its interactions with standard matter and through the cosmic environment. This approach relies on observations related to the outcomes of dark matter annihilations or decays.
Astrophysical Observations
Astrophysical observations provide valuable insights into the presence of dark matter through the study of cosmic phenomena. These involve looking for signatures that may suggest the existence of dark matter, such as the distribution of galaxies and clusters in the universe. A key characteristic of this method is that it allows scientists to gather information from large-scale structures without requiring direct interactions with dark matter.
Astrophysical observations are beneficial due to their ability to cover vast areas of space and time, yielding information about dark matter's gravitational effects. However, interpretations of these observations can be complex, as they are influenced by numerous other factors, including baryonic matter behavior. Discerning between different cosmological models becomes crucial.
Gamma-ray Anomalies
Gamma-ray anomalies represent another indirect detection method. This approach examines high-energy gamma rays that may result from dark matter interactions or annihilations. A characteristic of studying gamma-ray anomalies is the search for excess gamma ray emissions from regions with high dark matter density, like the center of galaxies. This method is advantageous due to advancements in gamma-ray observatories like the Fermi Gamma-ray Space Telescope.
The unique aspect of gamma-ray anomalies lies in their potential to reveal hidden properties of dark matter, such as mass and cross-section. Despite its promise, reliance on gamma-ray signatures can lead to challenges, as background noise from standard astrophysical sources can complicate the interpretation of results.
Collider Experiments
Collider experiments explore dark matter by using high-energy particle collisions to create conditions where dark matter particles may be produced. These setups can also offer critical information on the properties of dark matter.
LHC Searches for Dark Matter
The Large Hadron Collider (LHC) is at the forefront of collider experiments searching for dark matter. It operates by smashing protons at extremely high speeds, creating a myriad of particles. A notable aspect of the LHC searches for dark matter is its ability to probe the energy scales where dark matter particles might exist. The LHC's finding of the Higgs boson has also opened doors to studying its potential interactions with dark matter.
The LHC is a popular choice as it has the infrastructure to perform a wide range of collisions, allowing scientists to test various theories concerning dark matter. However, collider experiments depend heavily on theoretical predictions, and distinguishing dark matter signals from background processes can be particularly challenging.
Future Collider Prospects
Future collider prospects aim to extend current research into dark matter. Proposed designs, such as the Future Circular Collider, intend to surpass the energy capabilities of the LHC. A key feature of future colliders is their promise to explore regions of parameter space that remain inaccessible today. These facilities can enhance the understanding of dark matter candidates significantly.
Yet, these large-scale facilities face hurdles, including funding and technological advancements required for construction. Continued research and investment will be essential to translating theoretical predictions into viable experimentation.
The search for dark matter is ongoing, relying on a diverse array of detection methods combining theoretical insight and experimental precision.
Through these detection methods, researchers progress in their quest to unveil the elusive nature of dark matter, contributing to a more profound understanding of the universe.
Astrophysical Evidence
Astrophysical evidence provides critical insights into the existence and nature of dark matter. This field serves as a bridge between theoretical predictions and observations made in the cosmos. By analyzing various astrophysical phenomena, scientists aim to understand the unseen components of the universe that contribute to its structure and dynamics. Here, three key aspects are detailing how scientists gather evidence for dark matter: galaxy rotation curves, gravitational lensing, and the cosmic microwave background.
Galaxy Rotation Curves
Galaxy rotation curves are one of the most pivotal pieces of evidence supporting the dark matter hypothesis. Observations indicate that the outer regions of galaxies rotate at unexpectedly high speeds.
In a typical scenario, one would anticipate that stars on the outskirts of a galaxy should rotate more slowly than those closer to the center due to gravitational pull from the galaxy’s visible mass. However, measured speeds show little to no decrease as distance from the core increases. The rotational velocities remain constant, suggesting a substantial mass influence beyond the visible matter. This discrepancy implies the existence of an unseen component—dark matter—that exerts gravitational force but does not emit light or energy that we can detect.
Gravitational Lensing
Gravitational lensing occurs when massive objects like galaxy clusters bend the light from more distant objects behind them. This effect, as described by Einstein’s theory of general relativity, allows scientists to study mass distribution in the lensing object without directly observing it.
The degree of lensing is dependent on the total mass of the galaxy cluster, including dark matter. Observations from telescopes notably show how light from background galaxies is smeared and distorted as it passes through the gravitational field of clusters. Variations in the lensing effect across different wavelengths provide vital data on the presence and behavior of dark matter.
Cosmic Microwave Background
The cosmic microwave background (CMB) serves as a remnant heat signature from the early universe, its uniformity and slight fluctuations crucial for understanding cosmic evolution. Variations in the CMB reveal information about the density and distribution of matter, including dark matter.
Different models of cosmic evolution suggest that if we could measure how density fluctuations have evolved over time, we could better comprehend the role dark matter plays in the formation of large-scale structures within the universe. Studies analyzing the CMB support the notion that dark matter constitutes a significant portion of the universe's mass-energy content.
"The evidence drawn from astrophysical phenomena strengthens the foundation of dark matter research and presents physics with profound questions about the universe's composition and origin."
In summary, the study of astrophysical evidence is fundamental to grasping the complexity surrounding dark matter. The behaviors of galaxy rotation curves, aspects of gravitational lensing, and observations related to the cosmic microwave background all provide essential insights, guiding researchers in their quest to understand this enigmatic component of our universe.
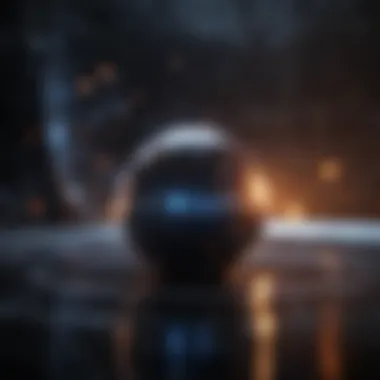
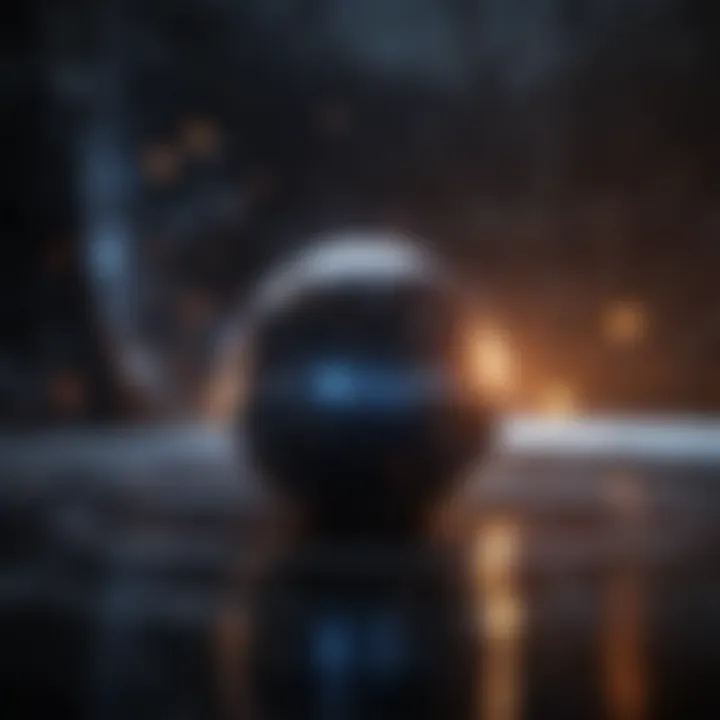
Current Research Initiatives
The quest to uncover the nature of dark matter is driving numerous research initiatives around the world. These initiatives play a crucial role in advancing our understanding and the search for dark matter. Each initiative has its unique methodology, technological focus, and collaborative efforts that contribute to the larger body of knowledge. Exploring these current research initiatives highlights the interconnected nature of this field and the shared goals among various research bodies.
Global Collaborations
In the realm of astrophysics, global collaborations are essential for pooling resources and expertise. Different regions have unique sites that lend themselves to specific experiments.
Experiments in Europe
Experiments conducted in Europe, particularly those associated with CERN, focus on cutting-edge particle physics. The European continent has a reputation for hosting high-profile experiments like the Large Hadron Collider, which seeks to explore particle interactions related to dark matter.
- Key Characteristic: A significant advantage of European experiments is their extensive funding and collaboration network that includes multiple countries.
- Unique Feature: Many facilities in Europe also benefit from access to diverse terrains, which is beneficial for detecting cosmic rays and conducting low-background experiments.
However, the competition for funding can sometimes restrict the scope of certain initiatives, leading to delays in progress.
Expeditions in South America
In contrast, expeditions in South America, particularly in places like the Andes mountains, offer a different approach. These regions are significant for their isolation and altitude, making them ideal for experiments that minimize cosmic radiation interference.
- Key Characteristic: The natural surroundings provide a quieter backdrop for sensitive detectors, improving the chance of directly observing signals from dark matter particles.
- Unique Feature: Projects like the DEAP-3600 experiment in Canada and South American initiatives are increasingly gaining traction due to their unique positioning and environmental factors.
Challenges include logistics and local political considerations, which can sometimes complicate long-term research efforts.
Emerging Technologies
The development of emerging technologies is a vital aspect of current research initiatives. Advances in detector technologies, computing algorithms, and data analysis techniques enhance the potential for discoveries in dark matter research. These innovations often stem from interdisciplinary collaborations, bringing together experts from physics, engineering, and computer science.
Notable Research Entities
CERN
CERN is perhaps the most recognized institute for particle physics research. Its contribution to dark matter studies lies in its ability to create collisions at unprecedented energy levels. This research has the potential to reveal new fundamental particles or interactions that are pivotal in the dark matter discourse.
- Key Characteristic: CERN's extensive infrastructure enables various experiments under one umbrella, enhancing collaborative flexibility.
- Unique Feature: The ability to bring together scientists from around the world fosters an environment of innovation but can also result in bureaucratic delays.
LBL
The Lawrence Berkeley National Laboratory (LBL) is noted for its focus on direct detection experiments. These efforts aim to identify potential dark matter signals through unique detection methods that exploit low-background conditions.
- Key Characteristic: LBL has a strong emphasis on cross-disciplinary research which opens new avenues in dark matter detection.
- Unique Feature: Its advanced facilities provide significant advantages for precision measurements, although such high-precision experiments often demand extensive funding and time.
FERMILAB
FERMILAB's role is significant within the U.S. for dark matter research, particularly its focus on neutrino experiments that can indirectly inform about dark matter interactions.
- Key Characteristic: Its emphasis on neutrinos provides a unique perspective that complements traditional dark matter search efforts.
- Unique Feature: FERMILAB's research also faces challenges due to political and funding fluctuations that affect ongoing projects.
The collaborative efforts across these institutions represent the forefront of dark matter research. Their combined expertise and resources create an ecosystem where new ideas can flourish, although challenges remain in achieving coherent progress.
Such initiatives demonstrate the global commitment to unravel the enigma of dark matter. Each program and partnership carries its unique strengths and obstacles, but all contribute towards the greater goal of understanding the universe.
Challenges in Detection
The quest for dark matter presents numerous hurdles that researchers must overcome. These challenges are not merely technical but deeply intrinsic to the characteristics of dark matter itself. Understanding these challenges is essential as they affect the methodologies employed in detection and dictate the course of research in this field.
Technological Limitations
One of the foremost challenges is related to current technological limitations. Many existing detection methods heavily rely on sophisticated equipment that is still in developmental stages. Detectors must be sensitive enough to pick up signals from dark matter interactions, which are incredibly weak. For instance, devices like the LUX-ZEPLIN (LZ) dark matter detector require ultra-low temperatures and sophisticated shielding to isolate signals from background noise.
Each technological element, from materials used to signal processing techniques, must be optimized. Scientists are continuously innovating to enhance capabilities, yet the process is slow. The advancements in materials science and electronic engineering are crucial for the development of detectors that can provide more reliable results. These limitations pose significant delays in our understanding of dark matter.
The Nature of Dark Matter
The very nature of dark matter creates significant challenges for detection. Its properties influence how it interacts with other particles, complicating the monitoring or measurement of its presence.
Low Cross-Section Interactions
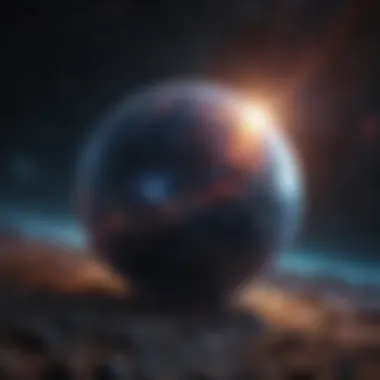
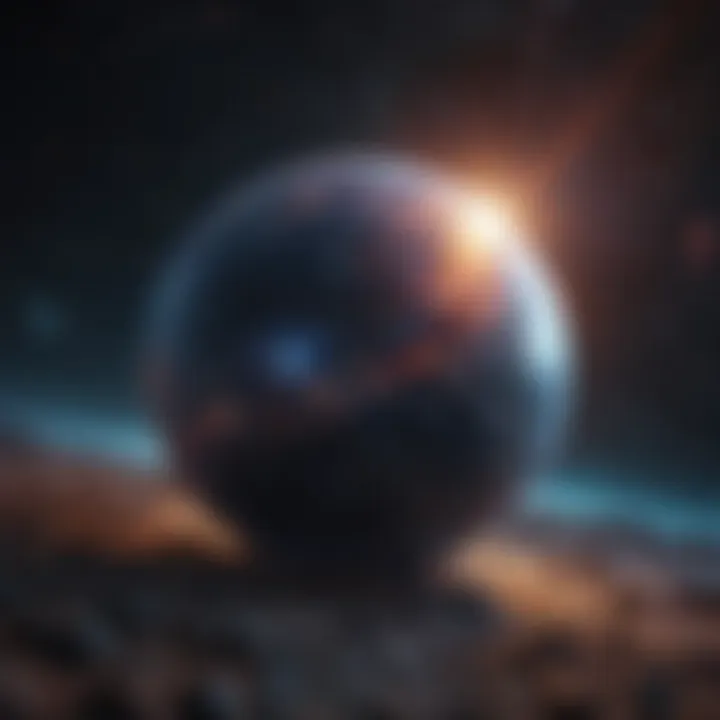
One aspect of dark matter that presents a challenge is low cross-section interactions. This term refers to the likelihood of dark matter particles interacting with normal matter. The low interactions mean that when dark matter particles pass through detectors, they may not produce any detectable signals at all. This characteristic is essential because any successful detection method must account for these interactions.
A key feature of low cross-section interactions is its inherent rarity. Because dark matter is hypothesized to interact very weakly, researchers struggle to collect sufficient data for analysis. While beneficial in theoretical frameworks, this property makes measuring and studying dark matter interactions extremely difficult.
Decaying and Annihilating Particles
Another significant aspect is the existence of decaying and annihilating particles. When dark matter particles collide, they can produce standard particles, potentially observable signals in detectors. However, this process introduces further complexity. The rates at which these events occur are highly variable. Anti-matter generation depends on multiple factors, including the type of dark matter candidate being studied and environmental conditions.
A prominent characteristic of decaying and annihilating particles is that they can lead to identifiable signals in gamma-ray, neutrino, or other spectra, which may indicate dark matter interactions. Yet, these signals are often indistinguishable from cosmic background radiation, complicating observational efforts significantly.
The uncertainty surrounding these characteristics illustrates the depth of the challenges faced in the search for dark matter. Each of these elements underscores the necessity for ongoing research and innovation in detection methodologies.
Implications for Physics
Understanding dark matter has profound implications for our perceptions of fundamental physics. The quandary of dark matter touches upon core principles that govern our universe, prompting a reevaluation of long-standing theories and models. As researchers delve deeper into its nature, they are forced to confront the limitations and adequacy of existing frameworks, such as the Standard Model of particle physics.
Revising Existing Theories
Dark matter's mystery lies not only in its absence but also in its elusive characteristics. Researchers must reassess theoretical constructs that have held sway for decades. For one, the possible existence of new particles challenges the standard model. If particles like WIMPs or axions are found to exist, this could necessitate a significant paradigm shift in our understanding of particle interactions. Additionally, the possibility of dark matter influencing the evolution of cosmic structures suggests that current models of cosmological evolution may need enhancement or revision.
- The discovery of new phenomena could lead to:
- Extended models beyond the Standard Model.
- Insights into the interactions between matter and dark matter.
- Reevaluation of quantum field theories.
The implications are broad-reaching, influencing not only theoretical physics but also experimental approaches in particle physics and cosmology.
Impact on Standard Model
The Standard Model provides a comprehensive framework for understanding particle physics, but the question of dark matter pushes its boundaries. Current hypotheses propose that dark matter does not interact via electromagnetic force, which introduces complications. It raises challenging questions about the particles’ nature and origin within the Standard Model framework.
"If dark matter is indeed made up of new types of particles, it could indicate the need for a new theoretical framework entirely."
Key considerations include:
- Potential for New Physics: The discovery of dark matter may introduce new particles or forces that extend beyond what the Standard Model currently describes.
- Gravitational Interaction: Dark matter’s gravitational effects are critical for understanding the broader universe. As such, new insights could reshape the cosmological constant and theories about cosmic inflation.
- Particle Colliders: Future experiments at facilities like CERN may provide evidence of dark matter particles and consequently reshape physics education and technology.
The quest to uncover dark matter’s secrets is not merely an academic exercise. It has practical consequences that could redefine various areas of physics. The outcome of this investigation into dark matter holds significant potential to reshape our understanding of the universe at both microscopic and cosmological levels.
Future Directions in Research
The pursuit of understanding dark matter is at a pivotal juncture, with many researchers exploring new strategies and technologies. Recognizing the necessity of advancing this field is essential for unraveling the cosmos' enigmas. Future directions in research present opportunities to enhance methodologies, refine theoretical frameworks, and develop better detection techniques. As we aim for a deeper comprehension of dark matter, these directions will prove vital in guiding our efforts.
Upcoming Experiments
Several experiments are set to launch in the coming years that aim to delve deeper into the mystery of dark matter. High-profile projects such as the LUX-ZEPLIN (LZ) experiment in the United States intends to utilize ultra-sensitive technology to detect potential dark matter interactions within massive liquid xenon detectors. This experiment could significantly lower the threshold for detection of Weakly Interacting Massive Particles (WIMPs), enhancing our understanding of their properties.
In addition, the PICO collaboration is working on the use of bubble chambers to capture interactions between dark matter and normal matter. This approach provides a unique method of detecting rare interactions, potentially offering new insights into dark matter candidates. Furthermore, the Cherenkov Telescope Array (CTA) is set to engage in indirect detection through high-energy gamma-ray observation, helping us to identify products of dark matter annihilations or decays.
These experiments hold promise not just for the discoveries they may make, but for the technological advancements they foster. Collaboration among global scientists enhances innovation, generating solutions to existing challenges in detection.
Theoretical Predictions
Theoretical predictions related to dark matter are continually evolving. As experiments progress, the predictions can guide how data should be interpreted. For instance, ongoing work in particle physics suggests modifications in the Standard Model, proposing additional particles or forces that could interact with dark matter.
Another significant area of theoretical research is the study of alternative models to dark matter. Concepts such as Modified Newtonian Dynamics (MOND) provide alternatives to the existence of dark matter. While traditional models face scrutiny, exploring these theories could illuminate new pathways in understanding gravitational phenomena.
Moreover, predictions regarding dark energy's role in the universe can impact interpretations of dark matter. Understanding the interplay between dark matter and dark energy might provide holistic insights into the universe's structure and expansion.
In summary, as upcoming experiments and theoretical predictions shape the landscape, they will function as cornerstones for future research in dark matter. Each step taken will contribute to unraveling the complex nature of this elusive substance, bringing us closer to a fuller understanding of the cosmos.
End
The conclusion of this article serves as a critical reflection on the intricate search for dark matter. It underscores the significance of understanding dark matter, an elusive component of our universe, and the implications it has on both cosmology and particle physics. The journey through dark matter research highlights not only the achievements but also the continuous challenges researchers face.
Summary of Findings
In reviewing the findings from this article, several key points emerge. Dark matter, though invisible and undetectable through conventional means, plays a crucial role in the formation and structure of galaxies. Its gravitational effects can be observed indirectly through phenomena such as galaxy rotation curves and gravitational lensing. Throughout various sections, we discussed the main candidates for dark matter, including Weakly Interacting Massive Particles (WIMPs) and sterile neutrinos, showcasing the ongoing theoretical endeavors to identify the correct particles.
Research initiatives, both collaborative and independent, drive the search for dark matter. Significant investments in technology and experimental methods, including underground detectors and collider experiments at facilities like CERN, have paved the way for more sophisticated searching techniques. The importance of global cooperation cannot be emphasized enough, as scientists pool their resources and knowledge to tackle this cosmic mystery.
The Continuing Quest for Understanding
The search for dark matter is far from over. Researchers are constantly reevaluating and refining their approaches. The implications of discovering dark matter are profound, potentially leading to new physics that alters our understanding of the universe. Ongoing advancements in technology and theoretical models present an optimistic outlook for future explorations.
"The relentless pursuit of dark matter is not merely about addressing a scientific enigma; it serves as a testament to human curiosity and the desire to comprehend the cosmos."