Understanding UV-Vis Spectrophotometers in Depth
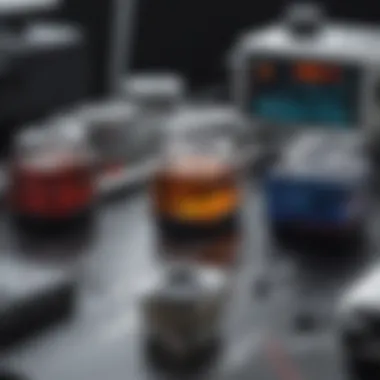
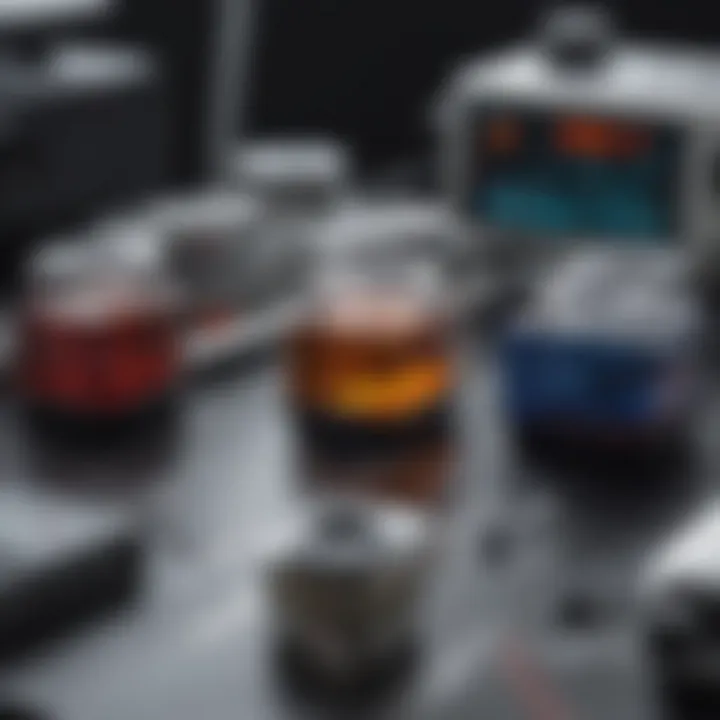
Intro
Ultraviolet-visible (UV-Vis) spectrophotometers are essential instruments in scientific research with various applications. These devices measure how much light is absorbed by a sample at specific wavelengths. Their significance extends across numerous fields, including chemistry, biology, and environmental science. Understanding the functioning principles behind UV-Vis spectrophotometers is important for students, researchers, educators, and professionals alike.
The operational mechanics involve intricate interactions of light, optics, and electronic systems. By analyzing the absorption of light, users can gather valuable data about the chemical composition and properties of samples under investigation. In the sections that follow, we will explore these fundamental concepts in a detailed manner.
Prelude to UV-Vis Spectrophotometry
UV-Vis spectrophotometry is an essential analytical technique widely used in various scientific disciplines. Its ability to provide quantitative and qualitative information about substances makes it a critical tool in both research and practical applications. Understanding this method is vital for students and professionals who aim to grasp the principles of light absorption and the analytical capabilities it offers.
Definition and Purpose
UV-Vis spectrophotometry involves measuring the intensity of light as it passes through a sample. The fundamental concept is based on the absorption of ultraviolet and visible light by molecules. When light encounters a substance, certain wavelengths are absorbed while others are transmitted or reflected. The degree of absorption at specific wavelengths can be correlated to the concentration of the substance in the sample according to Beer-Lambert's Law. This analytical method is widely utilized in areas such as chemistry, biology, and environmental science, providing insights into the properties of materials, reaction kinetics, and molecular structures.
Historical Development
The roots of UV-Vis spectrophotometry trace back to the early 20th century when scientists began to explore the interactions between light and matter. Notably, in the 1940s, advances in optics and mass production of photodetectors led to the development of the first commercial spectrophotometers.
These instruments evolved significantly over the decades, with improvements in precision and sensitivity. In recent years, the introduction of digital technology and computer-based data analysis has further refined this technique, making it accessible to a broader range of applications and users. Such historical developments show a progression from rudimentary instruments to sophisticated devices that cater to complex analytical needs.
Fundamental Principles of Spectroscopy
Understanding the fundamental principles of spectroscopy is essential for grasping the workings of UV-Vis spectrophotometers. This area of study encompasses the interaction of light with matter, a process that is pivotal in numerous applications across various scientific fields. By laying down these basics, we prepare the foundation for evaluating data stemming from spectral instruments.
Spectroscopy encompasses several key concepts, including the nature of light, the mechanisms of absorption and transmission, and the contrasts between different spectroscopic methods. Each of these elements plays a crucial role in the functionality and application of UV-Vis spectrophotometers.
Nature of Light
Light is both a wave and a particle, described by the duality principle in physics. It travels as waves, characterized by their wavelength and frequency. The electromagnetic spectrum covers a wide range of wavelengths, extending from gamma rays to radio waves, with ultraviolet (UV) and visible light situated in between.
In UV-Vis spectroscopy, it is crucial to understand how light behaves when interacting with molecules. Specifically, light can be absorbed, transmitted, or reflected when coming into contact with a substance. The absorbed light corresponds to specific energy transitions in electrons within atoms or molecules. This transition is what allows scientists to deduce information about the composition and concentration of substances.
Absorption and Transmission
Absorption occurs when molecules in a sample capture light energy, causing electrons to jump from a lower energy level to a higher one. This process is selective, meaning specific wavelengths will be absorbed depending on the electronic structure of the molecule.
Conversely, transmission refers to the passage of light through a sample without being absorbed. When light transmits through a solution, it can provide a clear view of what remains after specific wavelengths are absorbed. The ratio of absorbed light to transmitted light is fundamental for calculating absorbance, which in turn aids in quantifying concentration levels.
"The amount of light absorbed by a solution is directly related to the concentration of the absorbing species present within that solution."
This principle is summarized in Beerβs Law, which states that absorbance is directly proportional to concentration. Understanding these aspects of light is vital for interpreting results produced by UV-Vis spectrophotometers.
To encapsulate:
- Light behaves as both a wave and a particle.
- Absorption is selective based on a substance's electronic structure.
- Transmission helps analyze what remains after absorption.
- Beerβs Law establishes a relationship between absorbance and concentration.
These concepts form the bedrock on which spectroscopic analysis is built. They aid in developing a better understanding of how UV-Vis spectrophotometers function and how their data can be interpreted.
Components of a UV-Vis Spectrophotometer
The understanding of UV-Vis spectrophotometers is incomplete without an in-depth exploration of their components. Each part plays a crucial role in the operational efficiency and effectiveness of the instrument. An appreciation of these elements not only aids in comprehending how the device functions but also highlights its applications in various scientific fields. Knowing each component's role emphasizes the importance of accuracy and precision in measurements, particularly when utilized in chemical analysis, biological assessments, and environmental monitoring.
Light Source
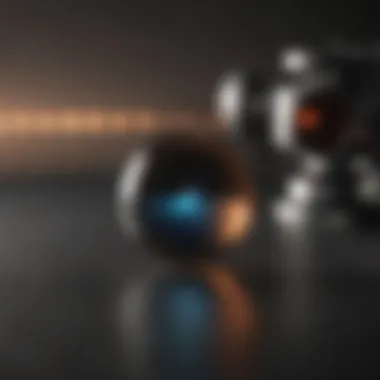
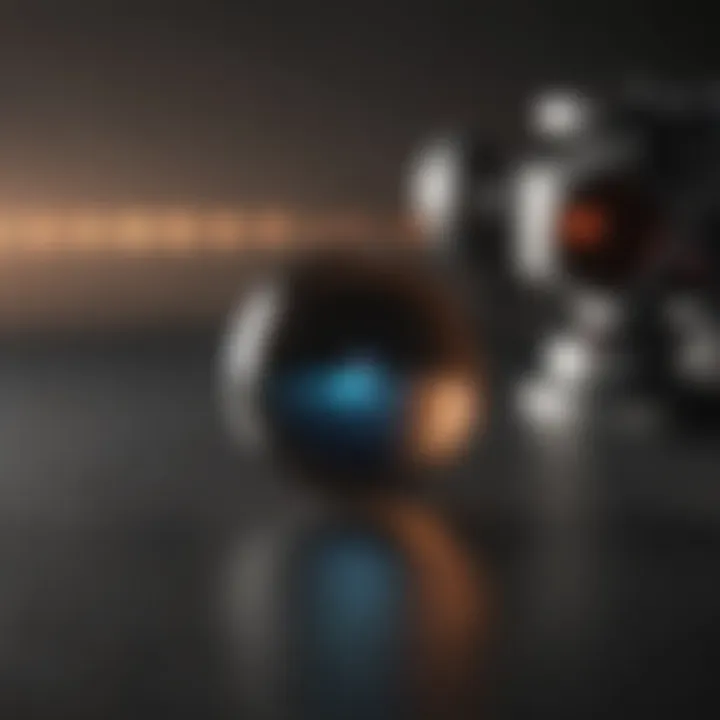
The light source is the first critical component of a UV-Vis spectrophotometer. It provides the necessary photons that interact with the sample being analyzed. Generally, UV-Vis spectrophotometers utilize two types of light sources: deuterium lamps for ultraviolet light and tungsten-halite lamps for visible light. These sources must emit a stable and continuous spectrum to ensure reliable measurements.
The wavelength range is essential. The quality of the source affects signal intensity which directly correlates to the resolution of the spectra collected. Regular calibration and maintenance of the light source are important to avoid fluctuations that may lead to inaccurate results. Moreover, the choice of light source can impact the applicability of the device in different fields, making this component vital for effective analysis.
Monochromator
Following the light source, the monochromator plays a significant role in UV-Vis spectrophotometers. Its function is to isolate specific wavelengths of light before it hits the sample. This isolation is achieved using either prisms or diffraction gratings. The monochromator ensures that only a narrow band of wavelengths is directed toward the sample, which is essential for accurate absorbance measurements.
A high-quality monochromator enhances spectral resolution and enables the detection of subtle peaks in the absorption spectrum. Changes in the alignment or calibration of the monochromator can adversely affect the results. Thus, careful control over its operation is necessary for achieving optimal performance.
Sample Holder
The sample holder is another key component that must be considered. This part ensures that the sample remains stable during measurements, minimizing any physical disturbance that could lead to inaccuracies. Typically, the holder uses cuvettes, which are usually made from glass, quartz, or plastic, depending on the sample's nature and wavelength range being analyzed.
Proper selection of the material for the sample holder is essential. It must be transparent to the wavelengths being measured and chemically inert toward the sample solution. Special attention should be given to cleanliness as impurities can distort the results. Therefore, standardized procedures for inserting samples must be established to ensure consistency in measurements.
Detector
Finally, the detector is responsible for converting light into an electrical signal, which is then processed to give absorbance values. Various types of detectors are used in UV-Vis spectrophotometers, including photomultiplier tubes (PMT) and charge-coupled devices (CCD). The choice of detector can influence the sensitivity and speed of the analysis.
The detector's efficiency in capturing the transmitted light directly impacts the overall sensitivity of the system. If the detector poorly responds to certain wavelengths, it could lead to inaccurate data interpretation. Continuous advancements are being made in detector technology, improving the performance and reliability of UV-Vis spectroscopy. Each of these components works in concert to facilitate accurate light measurement and analysis, making the understanding of their functions essential for anyone engaged in UV-Vis spectroscopy.
Understanding the roles of these components not only allows researchers to utilize UV-Vis spectrophotometers effectively but also aids in troubleshooting issues when they arise.
Operational Mechanism
Understanding the operational mechanism of UV-Vis spectrophotometers is essential for grasping their relevance in various scientific fields. This mechanism outlines how these instruments analyze the light absorption properties of substances, which is a core function of spectrophotometry. Knowing how UV-Vis spectrophotometers operate aids in optimizing their use in experiments and ensures accurate results. A clear view of the operational process reveals specific elements such as calibration and sample analysis, both of which are crucial for reliable measurements.
Calibration Process
The calibration process is an essential step in using UV-Vis spectrophotometers. Calibration ensures that the instrument provides accurate and reliable measurements, which is vital for scientific investigations. The calibration involves several phases, beginning with the selection of a standard solution. This solution typically has a known concentration of an absorbing species. By measuring the absorbance at specific wavelengths, the instrument can create a calibration curve, plotting absorbance against concentration.
Moreover, regular calibration is necessary to account for instrument drift over time, which can affect readings. During this procedure, one uses different concentrations of the standard solution to generate a set of data. From this, the relationship between absorbance and concentration can be established using the Beer-Lambert law, described as follows:
A = Ξ΅ * c * l
Where A is the absorbance, Ξ΅ is the molar absorptivity, c is the concentration, and l is the path length.
Once the calibration curve is established, it can be used for future measurements, allowing the researcher to determine the concentration of unknown samples based on their absorbance readings.
Sample Analysis Procedure
The sample analysis procedure follows calibration and is vital for obtaining data that contributes to scientific understanding. The process begins with the placement of the sample within the sample holder. It is important that the sample is prepared properly, as any impurities or air bubbles can affect the results. After ensuring the sample is ready, the researcher selects the desired wavelength of light using the monochromator.
As the light passes through the sample, a proportion is absorbed, while the remainder is transmitted. The detector captures the transmitted light, providing a measurement of its intensity. This measurement is then compared to the intensity of the light that initially entered the sample, allowing the calculation of absorbance.
The detailed steps include:
- Preparing the sample adequately to avoid contamination.
- Select the wavelength that corresponds to the maximum absorbance of the analyte.
- Measure the blank sample to correct for any background absorbance.
- Record the absorbance of the sample and correlate it with the established calibration curve.
A clear understanding of this procedure helps researchers obtain precise and accurate data from UV-Vis spectrophotometry, ultimately enhancing its applications in fields like chemistry, biology, and environmental science.
Data Interpretation
Data interpretation holds a crucial role in the utilization of UV-Vis spectrophotometers. It transforms raw data from measurements into meaningful insights. Analyzing absorbance and transmittance values is not merely a numerical exercise; it is a fundamental pathway to understanding a material's properties and behaviors. The significance of effective data interpretation is clear when considering the benefits it brings to various scientific fields including chemistry, biology, and environmental science.
Through interpretation, researchers can deduce concentrations of unknown samples, identify numerous components in a mixture, or quantify reaction kinetics. This ability to translate data into practical applications amplifies the utility of UV-Vis spectrophotometry in research settings. Moreover, it contributes to informed decision-making in both academic and industrial applications.
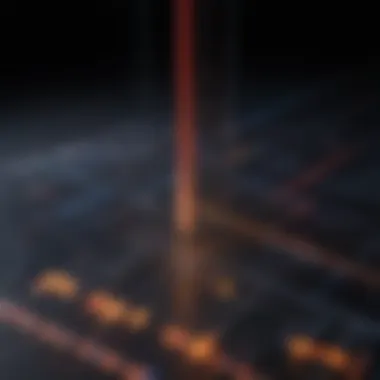
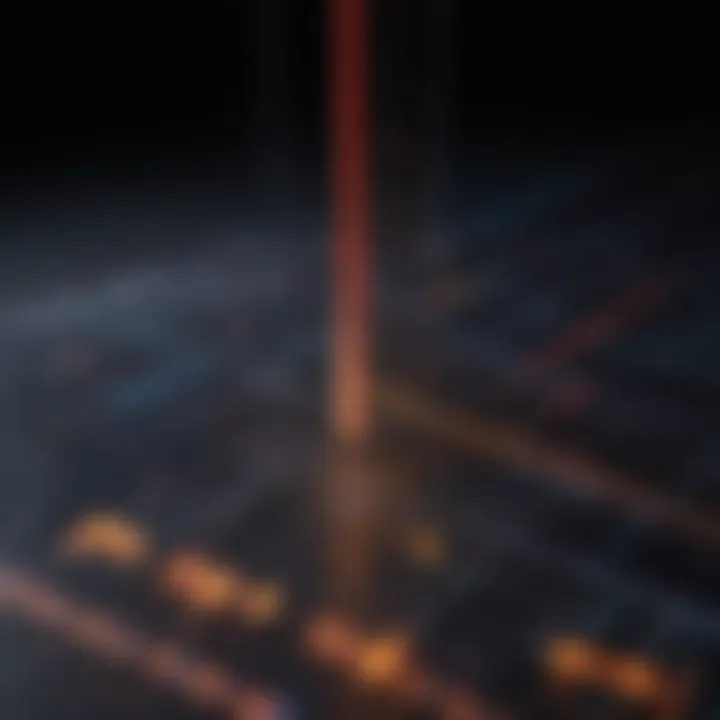
Considering that the data might be influenced by factors such as sample purity, instrument calibration, and environmental conditions, it becomes imperative for practitioners to exercise caution during interpretation. Accurate analysis requires a solid understanding of statistical methods and the specific characteristics of the sample under investigation.
"Correct interpretation of UV-Vis data can unlock insights that are otherwise hidden."
By grasping the nuances of data interpretation, users enhance their ability to conduct meaningful experiments and arrive at reliable conclusions, ultimately advancing scientific inquiry.
Absorbance and Transmittance
Absorbance and transmittance are the core concepts in UV-Vis spectrophotometry. Understanding these concepts is essential for interpreting spectral data accurately. Absorbance (
A
) represents the amount of light absorbed by the sample. It is calculated using the formula:
[ A = -\log_10(T) ]
where T is the transmittance.
Transmittance, on the other hand, indicates the fraction of light that passes through the sample. High absorbance means low transmittance and vice versa. These two parameters are directly related but convey different insights about the material's interaction with light.
Several factors affect absorbance and transmittance including:
- Concentration of the analyte
- Path length of the sample
- Wavelength of light used for measurement
In practical applications, absorbance is commonly used in quantitative analysis. By comparing the absorbance readings of a sample to a standard curve created from known concentrations, one can determine the concentration of an unknown sample effectively. This is especially important in chemistry and biochemistry for determining concentrations of various substances.
Spectral Analysis
Spectral analysis is the process of examining the spectrum of light absorbed or transmitted by a sample at various wavelengths. This analysis provides a fingerprint of the sample, allowing identification of different chemical species. Each substance has a unique absorption spectrum, meaning researchers can distinguish between complex mixtures.
The main steps involved in spectral analysis include:
- Collecting spectra at multiple wavelengths.
- Analyzing peaks in the spectra, as peaks correspond to specific wavelengths of light absorbed.
- Comparing the spectrum with reference spectra to identify substances.
Additionally, spectral analysis offers insights into molecular interactions and environmental factors that affect light absorption. It helps in understanding reaction dynamics and stability of compounds when light interacts with them.
Applications of UV-Vis Spectrophotometry
The applications of UV-Vis spectrophotometry are broad and varied, making it an essential tool in many scientific fields. This section discusses the significance of applying UV-Vis techniques across chemistry, biology, and environmental science. Each area reaps substantial benefits, enhancing research capabilities and contributing to advancements in those disciplines.
Chemical Analysis
In chemistry, UV-Vis spectrophotometry plays a pivotal role in quantitative analysis. It allows researchers to determine the concentration of a solute in a solution by measuring its absorbance at a specific wavelength. The Beer-Lambert Law underpins this technique, stating that absorbance is directly proportionate to concentration.
- Key Benefits:
- High sensitivity enables detection of low concentration samples.
- Rapid analysis reduces time and labor costs compared to traditional methods.
- Non-destructive testing preserves sample integrity.
Common uses include analyzing reaction kinetics, verifying chemical compositions, and studying pharmaceutical formulations. Furthermore, it also aids in quality control processes within the manufacturing sector, ensuring products meet specified criteria.
Biological Applications
The application of UV-Vis spectrophotometry in biology is prominent, especially in analyzing nucleic acids and proteins. The absorbance characteristics of these biomolecules allow for their quantification in solution, which is crucial in molecular biology and biochemistry experiments.
- Considerations:
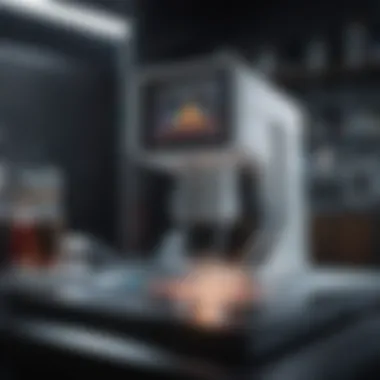
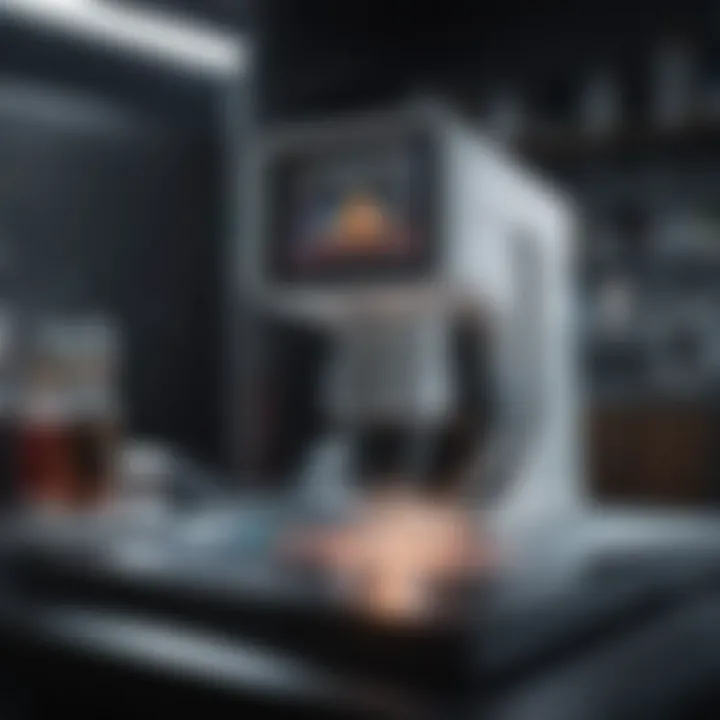
- DNA and RNA strongly absorb UV light, particularly at wavelengths around 260 nm.
- Proteins absorb light at 280 nm, making these measurements essential in assessing purity and concentration.
Additionally, UV-Vis spectrophotometry is utilized in enzyme assays, where enzyme activity can be measured based on the change in absorbance of substrate or product. Thus, it helps researchers gain insight into metabolic pathways and cellular functions.
Environmental Monitoring
In environmental science, UV-Vis spectrophotometry serves as a powerful tool for monitoring water quality. The technique can detect pollutants, organic substances, and harmful chemicals in both surface and groundwater. Measuring the absorbance at specific wavelengths allows for the identification and quantification of contaminants.
- Key Aspects:
- Utilized in determining levels of dissolved organic matter, chlorophyll concentrations, and heavy metals.
- Regular monitoring can prevent ecological damage, supporting conservation efforts.
Furthermore, it is employed in food safety applications, analyzing pesticide residues and additives. The method offers a rapid screening capability essential for regulatory compliance and public safety.
UV-Vis spectrophotometry's versatility across disciplines underscores its vital role in advancing scientific knowledge and ensuring ongoing environmental safety.
Limitations of UV-Vis Spectrophotometry
Understanding the limitations of UV-Vis spectrophotometry is essential for researchers and professionals who rely on this technique for data acquisition. While these instruments offer immense benefits, including speed and ease of use, their limitations can affect analytical results. Addressing these issues is vital for accurate data interpretation. By recognizing the shortcomings, scientists can optimize their methodologies and avoid common pitfalls in their analysis.
Sensitivity Issues
A key limitation of UV-Vis spectrophotometry is its sensitivity to low concentrations of analytes. UV-Vis instruments generally require a certain minimum concentration of a sample to produce reliable data. When analytes are present at very low levels, such as in some biological or environmental samples, detection becomes challenging. This issue can lead to inaccuracies in determining the concentration. Moreover, the instrumental noise can be more significant relative to the signal, making low-concentration analysis problematic.
Some factors that contribute to sensitivity issues include:
- Path Length: The standard path length typically used is one centimeter. However, if lower concentrations need to be measured, longer path lengths are more effective. This modification can help enhance light absorption, thus improving sensitivity.
- Wavelength Selection: Optimal wavelengths are crucial. Analytes exhibit different absorption spectra, and selecting a wavelength where the compound has peak absorbance can significantly enhance sensitivity.
- Matrix Effects: The presence of other substances in a mixture can interfere with the analyte's absorption properties. It may mask or alter responses due to competing interactions, which in turn affects measurement accuracy.
Interference Factors
Various interference factors can also limit the utility of UV-Vis spectrophotometry. These factors may originate from the sample matrix or from external sources, and they can lead to erroneous results. A thorough understanding of these interference factors ensures accurate results in data interpretation.
Common interference factors include:
- Chemical Interactions: Compounds in the sample can interact with the target analyte, altering its spectral properties. Such interactions may lead to the formation of complexes that absorb light at overlapping wavelengths.
- Scattering: In heterogeneous samples, light scattering can occur, leading to inaccuracies in transmittance readings. Scattering is prevalent in colloidal solutions or suspensions, which complicates quantification.
- Environmental Conditions: Fluctuations in temperature, pH, or ionic strength can affect measurements. Variability in these conditions can lead to changes in the absorbance characteristics of both the analyte and the matrix.
Understanding these limitations is crucial for anyone employing UV-Vis spectrophotometry in research. It emphasizes the importance of proper calibration, choice of wavelength, and consideration of sample purity.
In summary, while UV-Vis spectrophotometry is a powerful analytical tool, it is not without its limitations. By acknowledging sensitivity issues and interference factors, researchers can devise strategies to mitigate these effects. This approach helps ensure that UV-Vis spectrophotometry continues to be a reliable method for quantitative analysis across various disciplines.
Future Directions in UV-Visible Spectroscopy
The evolving landscape of UV-visible spectrophotometry shows vast potential. This section discusses future directions that promise to enhance the capabilities and applications of UV-Vis spectrophotometers. Understanding these trends is essential for students, researchers, educators, and professionals engaged in the sciences. As technologies develop, the relevance and functionality of UV-Vis spectroscopy are expected to escalate.
Technological Advancements
New technologies drive the future of UV-Vis spectrophotometry. Currently, advancements include miniaturization and the incorporation of artificial intelligence (AI). Examples of these breakthroughs include handheld devices for fieldwork and AI-based algorithms for data analysis. These developments permit quicker and more precise measurements.
Other notable advancements involve digital signal processing, leading to enhanced resolution and sensitivity. Improved optics in spectrophotometers can generate more accurate spectral data. Moreover, the integration of advanced software solutions, such as Chemometric techniques, allows for more strategic data interpretation. Researchers can analyze complex mixtures with greater ease and accuracy.
"Technological advancements not only improve existing capabilities but also create new avenues for exploration in scientific research."
Emerging Applications
The potential applications of UV-Vis spectrophotometry continue to grow in diverse fields. In environmental science, scientists utilize UV-Vis spectroscopy for monitoring water quality, assessing pollutants, and studying aquatic ecosystems. This technique provides valuable insights into environmental changes affecting biodiversity.
In the field of medicine, researchers explore non-invasive methods for diagnosing diseases. UV-Vis spectrophotometry offers a way to discern biomolecular interactions, making it valuable in drug development. The analysis of blood components using UV light is becoming increasingly prevalent.
Additionally, the agricultural sector benefits from UV-Vis spectroscopy. Rapid assessment of soil quality, crop health monitoring, and detecting plant diseases are gaining importance. Such applications enhance productivity and sustainability in food production.
The future of UV-visible spectroscopy is not limited to current applications. As technology becomes more accessible, interdisciplinary collaborations are expected to increase. This collaboration fosters innovative approaches to problems in various scientific domains, expanding the overall impact of UV-Vis spectrophotometry.