Understanding Tesla MRI Scans: Innovations & Implications
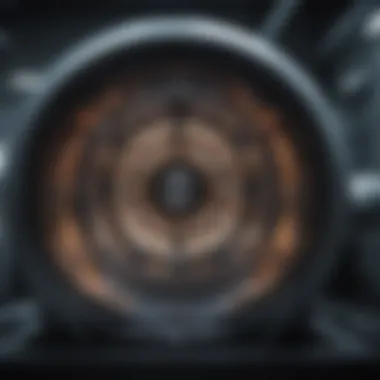
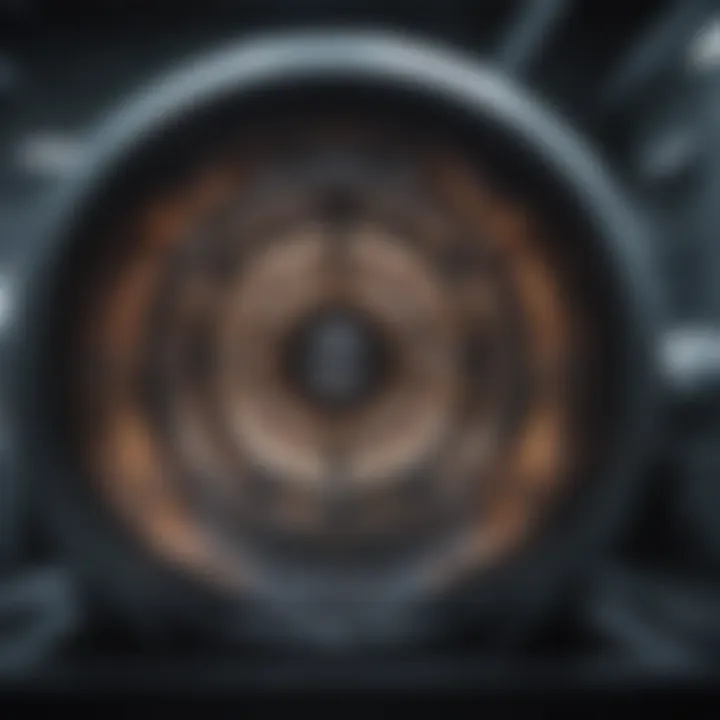
Intro
The field of medical imaging has undergone significant transformations, particularly with the advent of Tesla MRI scans. These high-field magnetic resonance imaging techniques are reshaping how clinicians view and interpret anatomical structures within the human body. By utilizing powerful magnetic fields measured in Tesla, these MRI systems enhance image resolution and diagnostic capabilities. As the demand for clear and precise imaging escalates, understanding Tesla MRI scansβalong with their innovations and implicationsβbecomes paramount for medical professionals, researchers, and educators.
Tesla MRI technology operates on complex principles that hinge on electromagnetic fields and nuclear magnetic resonance. Such a dynamic interplay not only improves visual clarity but also broadens the spectrum of applications in clinical settings. Ultimately, the implications of this technology extend beyond mere imagingβthey echo throughout the realms of diagnostic accuracy, patient safety, and healthcare efficiency.
In this article, we will explore the advancements in Tesla MRI scans, critically assessing their safety, image quality, and overall clinical importance. Through a comprehensive examination, we aim to unveil the future of medical imaging technology and its profound impact on healthcare.
Research Overview
Summary of Key Findings
The research into Tesla MRI scans highlights several key advances:
- Enhanced Image Resolution: High Tesla values correlate with improved signal-to-noise ratios, leading to clearer images.
- Rapid Imaging Techniques: New sequences allow for faster scans, reducing patient discomfort.
- Broader Clinical Applications: Tesla MRI scans are making headway into areas like functional MRI and diffusion tensor imaging.
- Safety and Regulatory Standards: Continuous scrutiny ensures that the rise in magnetic field strength does not compromise patient safety.
Research Objectives and Hypotheses
This article aims to:
- Analyze the innovations in Tesla MRI technology and their clinical relevance.
- Examine safety considerations linked with the use of high-field MRI scanners.
- Explore the potential future directions for MRI technology as shaped by ongoing research.
Methodology
Study Design and Approach
The study revolves around literature review combined with empirical analysis of recent advancements in MRI technology. By assimilating data from peer-reviewed journals, conference proceedings, and manufacturer reports, this article presents a cohesive narrative. The methodology is not merely descriptive; it also dissects the interplay of technology with clinical practices in various healthcare settings.
Data Collection Techniques
Data for this research have been obtained through:
- Systematic Reviews: Analyzing outcomes from various studies related to Tesla MRI scans.
- Clinical Trials: Evaluating the effectiveness of high Tesla systems compared to traditional lower-field scanners.
- Surveys and Questionnaires: Gathering insights from clinicians who employ Tesla MRI in practice, thereby understanding user experiences and challenges.
"The understanding of Tesla MRI scans signifies more than technological prowess; it embodies a commitment to advancing medical imaging and enhancing patient care."
The landscape of Tesla MRI scanning continues to develop, necessitating continuous learning and adaptation among professionals in the field. As we proceed, we will delve deeper into the operational principles that underline this innovative technology.
Prolusion to MRI Technology
Magnetic Resonance Imaging (MRI) has evolved significantly since its inception. It stands as a cornerstone of modern medical diagnostics, providing invaluable insights into human anatomy and physiology without the risks associated with ionizing radiation. This section introduces the fundamental aspects of MRI technology, which are vital for understanding its applications and implications.
Definition and Purpose of MRI
Magnetic Resonance Imaging is a non-invasive imaging technique that utilizes magnetic fields and radio waves to generate detailed images of organs and tissues inside the body. Primarily, the purpose of MRI is to aid in the diagnosis of various medical conditions, thereby facilitating effective treatment plans. For instance, MRI is pivotal in diagnosing conditions like tumors, brain disorders, and injuries to soft tissues. Its ability to produce high-contrast images of organs is one of its key attributes, making it more effective than traditional imaging techniques such as X-rays or CT scans in certain scenarios.
Overview of Magnetic Resonance Imaging
The MRI process begins with the patient being placed inside a circular magnet. This magnet emits a strong magnetic field that aligns the protons in the bodyβs hydrogen atoms. Radiofrequency pulses are then applied to disturb this alignment. When the radio waves are turned off, the protons return to their original position, releasing energy in the form of signals. These signals are detected by the MRI scanner and converted into images through complex algorithms.
The importance of MRI in clinical settings cannot be overstated. It allows for the visualization of internal structures with remarkable precision. Different MRI sequences can be employed to highlight various tissues, aiding in diagnosis and treatment planning. The technology continues to advance, with innovations focusing on increasing image quality, reducing scan times, and enhancing patient safety.
MRI technology is a pivotal tool in modern medicine, revolutionizing how we view and understand human anatomy.
Understanding Tesla Measurement in MRI
The measurement of Tesla in MRI is vital for understanding how magnetic resonance imaging technology operates. The Tesla (T) is the unit of measure for magnetic field strength, directly affecting image quality and resolution. Higher Tesla measurements indicate stronger magnetic fields, which enhance the clarity of images produced within MRI scans. This is especially important in clinical settings where accurate diagnoses are crucial.
When discussing Tesla measurements, it's significant to note that they influence not only the clarity of images but also the overall efficiency of the scanning process. With advancements in MRI technology, there is a growing emphasis on using higher Tesla systems to meet clinical and research demands. This article will address how these advancements impact patient outcomes, enhance screening processes, and facilitate more effective treatments.
What Does Tesla Mean?
Tesla, named after the famous inventor Nikola Tesla, quantifies magnetic field strength. One Tesla is defined as one weber per square meter. To put it into perspective, the Earth's magnetic field is approximately 0.00005 Tesla. In the context of MRI, the strength of the magnetic field influences the ability to excite hydrogen atoms in the body, allowing for detailed imaging of soft tissues.
MRI machines typically operate in various Tesla ranges. For instance, low-field MRI machines might operate around 0.2 to 0.5 Tesla, while clinical high-field MRI machines typically range from 1.5 to 3.0 Tesla. Ultra-high field MRI scanners are even stronger, surpassing 3.0 Tesla under certain conditions. Understanding these distinctions is essential for students and professionals alike, as they illustrate the intricate relationship between Tesla measurements and imaging techniques.
The Role of Magnetic Field Strength
Magnetic field strength plays a crucial role in MRI functionality. It affects not only image quality but also the specifics of the scanning process. Higher magnetic fields can produce clearer images and reduce the time needed for scans. Conversely, lower field strengths can result in noisier images and longer scanning times, which may not be suitable in urgent medical settings.
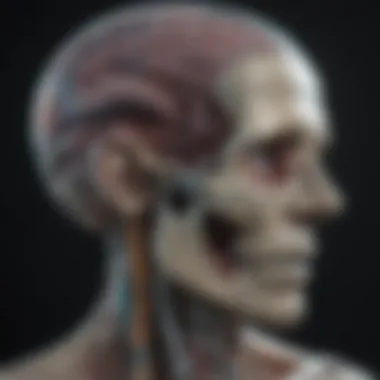
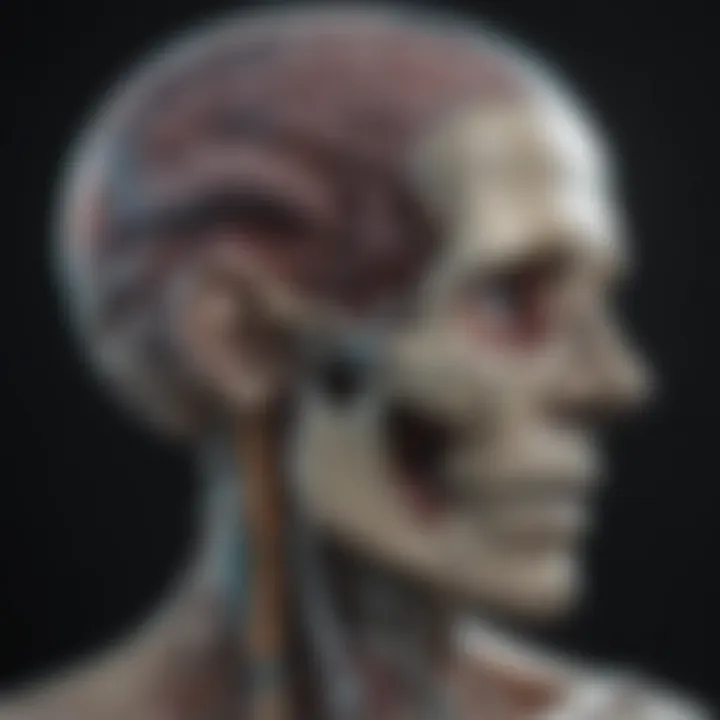
In practical terms, here are several impacts of magnetic field strength on MRI:
- Image Resolution: Higher Tesla levels improve image detail, allowing for better assessment of abnormalities.
- Signal-to-Noise Ratio: Increased field strength enhances the signal intensity, leading to sharper images with fewer artifacts.
- Scan Duration: Stronger fields can provide faster acquisitions of images, improving workflow in a clinical environment.
- Functional Imaging: High-field scanners facilitate advanced functional techniques, such as functional MRI, to assess brain activity.
"High Tesla MRI reduces time and increases clarity, transforming patient care in essential ways."
In summary, understanding Tesla measurements and their implications in MRI is crucial for professionals in the field. Knowledge of these elements contributes to more informed decisions in clinical practice, research, and patient management.
Types of MRI Scans by Magnetic Field Strength
Understanding the types of MRI scans based on magnetic field strength is crucial for comprehending how Tesla MRI systems function. Different configurations of magnetic fields provide varying levels of image quality and diagnostic capabilities. The classification into low and high Tesla MRI scans informs both clinical applications and research directions.
The choice between low and high Tesla MRI systems influences the precision of imaging outcomes. Each system has its strengths and considerations. These factors play a significant role in patient safety, image resolution, and diagnostic effectiveness. Thus, this segment aims to elucidate the distinctions between these MRI systems in detail.
Low Tesla MRI Systems
Low Tesla MRI systems typically operate at strengths of 1.5 Tesla or lower. These machines are often favored for their affordability, portability, and compatibility with various clinical settings. They make an effective option for general diagnostic purposes. Low Tesla systems deliver satisfactory imaging quality for many applications. This includes routine scans for neurological conditions and musculoskeletal assessments.
However, the image resolution can sometimes be less detailed than that of higher Tesla systems. This trade-off is a vital consideration when determining the appropriateness of a low Tesla system for specific diagnostic needs. Additionally, patient comfort may be improved due to the shorter scan times and reduced noise levels associated with these systems. Other advantages include lower operational costs. This is attractive for facilities with limited budgets.
Some notable points about low Tesla MRI systems include:
- Cost-effective solutions for various healthcare providers.
- Adequate imaging quality for many clinical applications.
- Reduced magnetic field strength leading to fewer safety concerns.
High Tesla MRI Systems
High Tesla MRI systems operate at strengths above 1.5 Tesla, reaching up to 7 Tesla in research settings. These systems are gaining popularity for their superior image quality and advanced diagnostic capabilities. High Tesla MRI offers enhanced resolution and contrast, allowing for detailed visualization of anatomical structures. This specificity is particularly beneficial in complex cases, such as when evaluating tumors or intricate neurological conditions.
However, higher Tesla strengths come with considerations of cost and patient safety. The operational expenses are significantly greater, which can be a barrier for many facilities. Patient screening becomes more critical, as some individuals with implants or certain health conditions may not be suitable candidates for high Tesla scans due to the powerful magnetic fields.
Some key aspects of high Tesla MRI systems are:
- Superior image clarity leading to improved diagnostic accuracy.
- Increased scanning time for comprehensive imaging protocols.
- Advanced applications in research and specialized clinical settings.
High Tesla MRI systems are not universally necessary. However, their presence enriches the landscape of MRI diagnostics, allowing for precise assessments in specialized medical contexts.
Clinical Applications of Tesla MRI Scans
The clinical applications of Tesla MRI scans are vast and encompass various fields within medical imaging. Understanding these applications is crucial as they enhance diagnostic capabilities and treatment planning. Tesla MRI scans provide important insights into diseases and conditions that are otherwise difficult to assess. The benefits of using higher Tesla systems include improved resolution and contrast, leading to better diagnostic accuracy.
In the context of neurological imaging, musculoskeletal imaging, and cardiac imaging, Tesla MRI scans play a significant role. Each of these areas requires distinct approaches and considerations, directly impacting patient outcomes. By focusing on the capabilities of Tesla MRI systems in these domains, one can appreciate the nuanced advantages they bring to contemporary medicine.
Neurological Imaging
Tesla MRI scans offer excellent detail in neurological imaging. The high field strength improves the visualization of brain structures and can aid in detecting abnormalities such as tumors, strokes, or degenerative diseases. For instance, a 3Tesla MRI machine can differentiate among small lesions that would be difficult to see with lower field strength machines. This is crucial in neurology, where timely diagnosis can significantly influence treatment options and outcomes.
Furthermore, functional MRI, which assesses brain activity, benefits from high Tesla systems. It provides a window into the dynamic functioning of the brain, enabling researchers and clinicians to understand neurological disorders better.
Musculoskeletal Imaging
In musculoskeletal imaging, the advantages of Tesla MRI scans are evident in their ability to produce detailed images of bones, cartilage, and soft tissues. High Tesla MRI systems, such as 3Tesla, can identify subtle injuries or pathological changes that may not be visible with traditional imaging modalities.
This clarity is especially beneficial in sports medicine, where accurate diagnosis of injuries is crucial for effective rehabilitation. For example, a higher Tesla scan can reveal meniscal tears in the knee or rotator cuff injuries in the shoulder with greater precision. This precision facilitates tailored treatment plans, enhancing recovery for patients.
Cardiac Imaging
Cardiac imaging with Tesla MRI scans represents a transformative step in how cardiovascular conditions are assessed. Magnetic resonance imaging is increasingly used for non-invasive evaluation of the heart's structure and function. High-field MRI systems provide improved contrast in cardiac tissues, enabling better visualization of cardiac chambers, valves, and surrounding tissues.
Advanced cardiac imaging techniques, including myocardial perfusion and viability assessment, thrive in high Tesla environments. The detailed images produced help cardiologists make informed decisions regarding interventions or management of heart diseases. The ability to visualize blood flow and heart wall motion can reveal complexities in conditions such as cardiomyopathy or coronary artery disease.
Conclusion: The clinical applications of Tesla MRI scans, particularly in neurological, musculoskeletal, and cardiac imaging, underscore the revolutionary impact of this technology on diagnostic medicine. They offer clinicians superior capabilities to detect and diagnose various conditions, thereby improving patient care.
Technical Aspects of Tesla MRI Scans
The technical aspects of Tesla MRI scans are essential for grasping how advancements in imaging technology are changing medical diagnostics. They encompass a range of crucial elements, including image acquisition techniques, image processing and reconstruction, and the use of contrast agents. Each of these components plays a significant role in enhancing image clarity and diagnosing conditions more accurately.
Image Acquisition Techniques
Image acquisition in MRI involves the methods and protocols used to capture detailed images of the body's internal structures. Various techniques influence the quality and speed of the imaging process. Common techniques include:
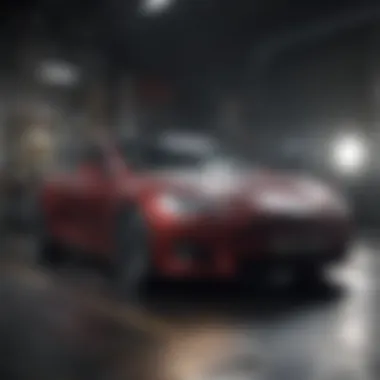
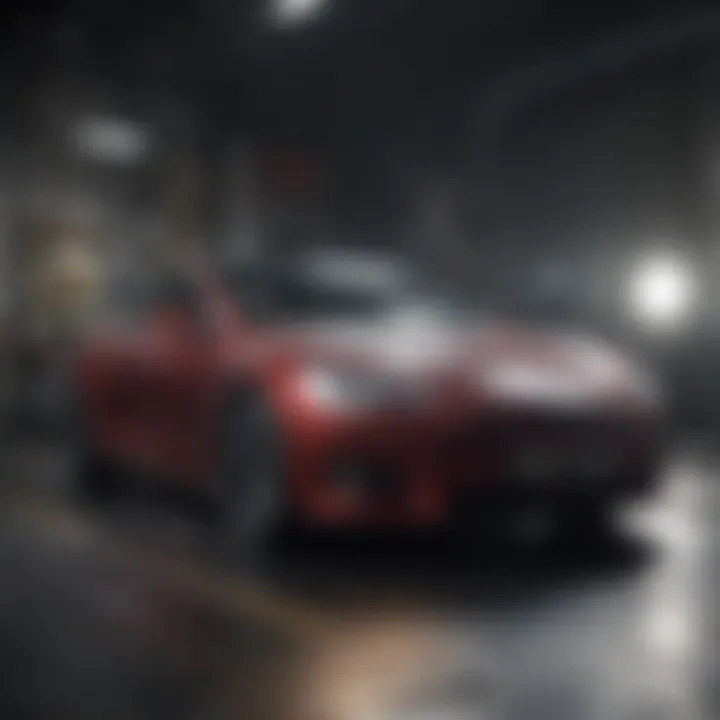
- Spin Echo: This is a widely used method that produces high-quality images, though it can be time-consuming.
- Fast Spin Echo: This variation is faster and helps reduce scan times, making it efficient for clinicians and patients alike.
- Gradient Echo: This technique captures images quickly, which is useful in dynamic imaging scenarios.
These methods utilize different sequences and timing of radiofrequency pulses and magnetic field gradients to optimize the images produced. The choice of technique can depend on the anatomical area being scanned as well as the specific clinical question at hand.
Image Processing and Reconstruction
Once the images are acquired, they undergo a rigorous processing and reconstruction phase. This is critical for transforming raw data from the MRI scanner into coherent and interpretable images. Key processes include:
- Fourier Transformation: This mathematical process converts the frequency data acquired by the MRI into spatial images.
- Noise Reduction Algorithms: These techniques enhance image quality by minimizing interference and improving the clarity of the images.
- Image Post-Processing Software: Advanced software allows radiologists to manipulate images, adjust contrast, or even generate 3D reconstructions for better visualization.
The quality of the final images depends highly on both the acquisition techniques used and the sophistication of the processing tools. Thus, staying current with innovations in this field is important for effective diagnostic practices.
Contrast Agents in Tesla MRI
The use of contrast agents in MRI is vital for improving the visibility of certain tissues and blood vessels. Contrast agents enhance the difference in signal between normal and abnormal tissue, making it easier to identify lesions or abnormalities. Common contrast agents include:
- Gadolinium-Based Agents: These are the most frequently used in MRI. They help delineate tumors and assess vascular conditions.
- Iron Oxide Particles: These are sometimes used for liver imaging and can be beneficial in specific scenarios.
Understanding the safety and effectiveness of these agents is crucial. For instance, the use of gadolinium has raised concerns regarding nephrogenic systemic fibrosis in patients with severe kidney disease. Hence, protocols for patient screening must also include consideration of the risks associated with contrast agents.
Key Consideration: Informed consent and thorough patient history are pivotal when planning for the administration of contrast agents during MRI scans.
Overall, the technical aspects of Tesla MRI scans significantly influence diagnostic capabilities. A deep understanding of these elements ensures that healthcare professionals can maximize the potential of MRI technology, leading to better patient outcomes.
Safety Considerations for Tesla MRI Scanning
The safety of patients and staff during Tesla MRI scanning is paramount in modern medical imaging. Understanding the specific safety considerations in this context helps to minimize risks associated with high magnetic fields and other operational factors. The implications of failing to address these safety aspects can be significant, leading to potential harm to individuals and affecting the integrity of imaging results.
Magnetic Field Safety
Magnetic fields generated by Tesla MRI machines can reach extraordinarily high levels. These fields can affect not only the imaging equipment but also any metallic objects in proximity. It is critical to establish safe boundaries to ensure that patients and healthcare providers are not harmed. The following points illustrate the importance of magnetic field safety:
- Impact of Strong Magnetic Fields: Strong magnetic fields can interact with certain medical implants, such as pacemakers and clips. This interaction may lead to device malfunction or injury to the patient.
- Personnel Safety: Staff members, particularly those who may have metal in their bodies, need to be cautious. Training and protocols must be established to protect them from unintended exposure.
- Emergency Situations: In the case of an emergency, removing a patient from the magnet may become complicated. Specific procedures should be developed and practiced regularly to ensure efficient response.
"Assessment of magnetic field safety in hospital settings is an ongoing necessity. It is not just about technology but about human lives."
Patient Screening Protocols
Patient screening is a critical phase before undergoing a Tesla MRI scan. Proper protocols help identify potential risks that may affect patient safety and imaging effectiveness. Mistakes in this preliminary assessment can lead to adverse reactions or misinterpretations of scans. Effective screening protocols include:
- Detailed Medical History: Patients must provide complete information regarding medical history. This includes any implants, surgeries, or conditions that may preclude safe MRI use.
- Physical Assessment: Physical examination may indicate potential contraindications for the MRI scan. It is essential to evaluate palpable abnormalities or signs that require additional caution.
- Informed Consent: Clear communication regarding the procedure, along with possible risks, must be provided to the patient. This fosters trust and ensures that patients are aware of their rights and the process they are undergoing.
Challenges and Limitations of High Tesla MRI Scans
High Tesla MRI scans present compelling advantages, but they also reveal several challenges and limitations that should not be overlooked. When addressing this topic, it is important to consider both technical and financial aspects that can impact the implementation and accessibility of this technology. Understanding these challenges provides context for navigating current innovations and potential future developments.
Technical Constraints
The technical constraints of high Tesla MRI scans can directly affect their effectiveness in clinical settings. Higher magnetic fields, such as those at 7 Tesla or more, may yield better image resolution and sensitivity. However, this also leads to increased complexity in the imaging process. Not all existing coils can effectively transmit and receive signals in ultra-high-field environments. This can result in suboptimal image quality if the appropriate technology is not employed.
In addition, high Tesla systems are often more susceptible to image artifacts. Such artifacts typically arise from patient movement or magnetic field inhomogeneities. This means that achieving consistent results may be more difficult compared to traditional scanners. The instability of the imaging environment requires designers and operators to develop protocols that account for these potential errors. This can include more rigorous patient preparations and tailored imaging sequences.
Moreover, the availability of trained personnel to manage advanced high Tesla systems can be limited. The knowledge required goes beyond standard MRI training, demanding specialized skills and understanding.
Cost Implications
Another aspect to consider is the cost implications of high Tesla MRI scans. The installation of 7 Tesla machines is significantly more expensive than standard 1.5 or 3 Tesla MRI systems. In addition to the initial hardware costs, maintenance and operational expenses can escalate quickly. These systems often demand advanced cooling solutions and specialized shielding, which can also add to total costs.
Moreover, research indicates that reimbursement rates for high Tesla scans may not fully align with the expenses incurred by healthcare providers. This creates economic disincentives for hospitals or clinics to invest in this technology, particularly those serving populations with limited insurance.
On the patient side, the costs related to higher Tesla scans can be passed onto individuals, making access to this advanced imaging option uneven. Thus, while high Tesla MRI technology offers some benefits, the financial burdens may limit widespread clinical adoption.
"Understanding the challenges behind High Tesla MRI is critical as it shapes the future of medical imaging technology."
In summary, the challenges and limitations associated with high Tesla MRI scans necessitate a comprehensive understanding. The technical constraints may hinder image quality and operational processes, while the cost implications can limit accessibility and sustainability in clinical practices. Recognizing these factors is crucial for any future advancements in MRI technologies.
Innovations in MRI Technology
The field of MRI technology is advancing rapidly. The innovations in this area have notable implications for both clinical practices and research methodologies. High Tesla MRI scans enable the acquisition of clearer images, providing a more nuanced view of human anatomy. The integration of new technologies contributes to improved diagnostic capabilities, ultimately influencing patient outcomes. This section delves into significant advancements that define the current landscape of MRI technology.
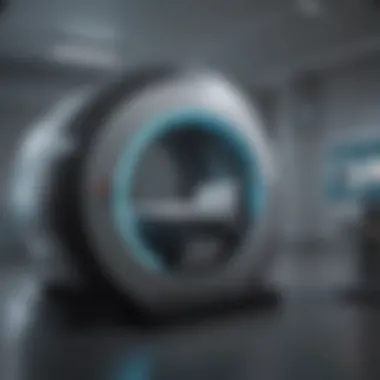
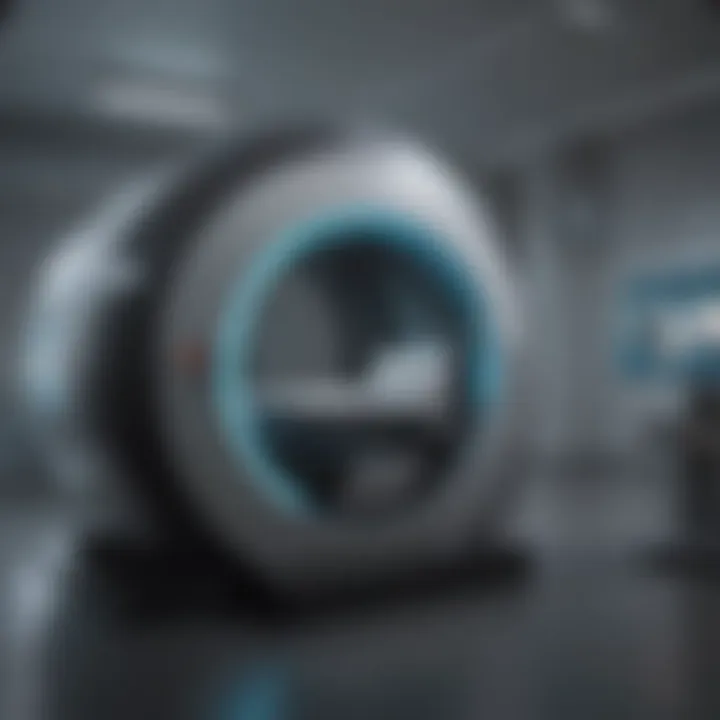
Advancements in Coil Technology
Coil technology is a cornerstone in the enhancement of MRI systems. The coils are crucial for signal reception and transmission. Recent advancements have concentrated on increasing the sensitivity and specificity of coils, leading to better image resolution and quality. For example, higher-density coils have been developed that allow for substantial improvements in signal-to-noise ratio, essential for creating high-quality images.
Moreover, phased array coils, which consist of multiple elements, enable simultaneous data collection from various anatomical positions. This dramatically decreases scan times and increases comfort for patients. Innovations in design and materials also contribute to flexibility in coil placement, optimizing the patientβs positioning during scans.
"Improved coil designs can lead to significantly enhanced image quality, which is essential for accurate diagnostics."
A notable example is the use of novel materials that reduce artifact generation. With reduced artifacts, radiologists can interpret images more reliably, leading to correct diagnoses and treatment plans. As coil technology progresses, it continues to redefine the scope of MRI applications, especially in specialized fields like cardiac imaging and neurological studies.
Integration of Artificial Intelligence
Artificial Intelligence (AI) is reshaping the landscape of medical imaging, including MRI technology. The integration of AI algorithms enhances the analysis of images, allowing for quicker and more accurate diagnoses. For instance, deep learning techniques enable computers to identify patterns in imaging data that might be missed by human eyes.
AI-driven tools can assist in auto-segmentation of anatomical structures, which reduces time spent by radiologists on manual tasks. Such automation leads to more efficient workflows in clinical settings. By utilizing AI, the reliance on standard protocols is diminished, and tailored solutions can be developed for a variety of clinical scenarios.
In addition, predictive analytics extend beyond imaging to forecasting patient health outcomes based on historical data combined with MRI findings. This predictive capability can enhance preventive measures, ensuring more proactive healthcare delivery.
The ongoing research into AI applications in MRI technology points toward a future where complex image interpretation becomes easier and more precise.
In summary, both advancements in coil technology and the integration of AI signify a pivotal shift in MRI capabilities. These innovations improve diagnostic accuracy, enhance patient experience, and promise to revolutionize future healthcare practices.
As these technologies continue to evolve, the implications for both patients and medical professionals become increasingly significant, warranting close study and engagement.
Future Directions in MRI Research
The direction of MRI research is pivotal as it shapes the future of medical diagnostics and treatment. Continuing to innovate in this field enhances the capabilities of magnetic resonance imaging while addressing complex medical scenarios. The evolution is driven by the need for improved accuracy, precision, and patient outcomes. It is essential to explore two key components in this realm: ultra-high field MRI and advancements in functional MRI techniques.
Exploration of Ultra-High Field MRI
Ultra-high field MRI refers to systems operating typically above 7 Tesla. These devices provide substantially clearer images compared to their lower Tesla counterparts. The increased magnetic field strength significantly improves the signal-to-noise ratio. This is crucial for delineating fine anatomical structures and detecting subtle pathologies.
The advantages of ultra-high field MRI include:
- Enhanced Resolution: It enables the imaging of smaller structures, which is especially beneficial in neurological studies.
- Better Contrast: Improved differentiation between tissues assists in accurate diagnoses.
- Functional Studies: Enhanced imaging allows for better observation of dynamic processes within the body, especially in brain research.
Researchers face challenges, however. Operating at higher field strengths can lead to increased susceptibility artifacts, potentially complicating image interpretation. Thus, despite its promise, further investigation is necessary to optimize these systems for clinical use.
Developments in Functional MRI Techniques
Functional MRI (fMRI) is a brain imaging technique that measures and maps brain activity by detecting changes in blood flow. The advancements in this area have been profound, allowing for a better understanding of brain functions and disorders.
Key developments include:
- Higher Temporal and Spatial Resolution: Newer methodologies enable capturing brain activity closely to real-time, providing insights into various cognitive tasks and responses.
- Machine Learning Integration: Leveraging machine learning algorithms improves the ability to analyze complex data sets, enhancing diagnostic capabilities and predictive modeling.
- Applications in Neuroscience: fMRI now plays a role in studying various neurological conditions and understanding brain connectivity, leading to strides in personalized medicine.
The journey of MRI technology continues to be dynamic and promising. As researchers explore and refine these advanced techniques, the implications for clinical practice will be significant, making it imperative to stay informed and engaged in this field.
The future of MRI research offers abundant possibilities to enhance diagnostic capabilities and optimize patient care through innovative imaging technologies.
Culmination
The conclusion section serves as a pivotal opportunity to synthesize the key discussions from the article. It distills the complex information regarding Tesla MRI scans into essential insights. Understanding the implications of these advancements not only clarifies the types of MRI technology available but also emphasizes their significance in clinical practices.
This summary encapsulates the key points discussed throughout the previous sections. The exploration of Tesla measurements reveals their vast impact on image quality and diagnostic capabilities. Notably, the advancements in technology enhance the ability to visualize intricate anatomical structures, leading to more accurate diagnoses. Furthermore, continued research points towards a future with even more sophisticated MRI applications, raising the standard of patient care.
"The ongoing developments in MRI technology promise a transformative impact on imaging practices within medicine."
The considerations surrounding safety and technical limitations are also emphasized in this conclusion. The importance of adhering to strict screening protocols and understanding the limitations of high Tesla MRI scans cannot be overstated. A comprehensive grasp of these factors is vital for both practitioners and patients to ensure optimal safety and efficacy.
This section ultimately reinforces the essential role that Tesla MRI scans play in the medical field, highlighting the ongoing need for innovation and research in this domain.
Summary of Key Insights
In this article, several key insights have emerged regarding Tesla MRI scans.
- Tesla Measurement Impact: The Tesla measurements fundamentally influence the resolution and quality of images. Higher Tesla levels generally correlate with better imaging results.
- Clinical Applications: Different fields, such as neurology and cardiology, greatly benefit from advancements in MRI technology. This includes improved diagnostic capabilities that can guide treatment decisions.
- Safety Protocols: The safety of patients undergoing MRI scans is paramount. Understanding the magnetic properties and developing methodical screening processes can mitigate risks.
- Technological Innovations: Advancements in techniques, such as coil technology and AI integration, are redefining what MRI can achieve, offering richer, clearer images in less time.
- Future Directions: Research in ultra-high field MRI and functional imaging techniques suggests an exciting trajectory for the discipline of medical imaging.
These insights provide a robust framework for understanding where MRI technology stands today and where it might go in the future.
The Path Ahead for MRI Technology
The pathway forward for MRI technology is both exciting and challenging. As with any field, constant innovation is essential to keep pace with the growing demands of medical imaging. Several areas stand out as particularly relevant.
- Ultra-High Field MRI: Research into ultra-high field MRI technologies promises improved resolution and contrast in imaging. This could allow for visualizations that were previously unattainable, potentially transforming diagnostic accuracy.
- Functional MRI Advances: Developments in functional MRI techniques will strengthen the understanding of brain functions and could lead to breakthroughs in how neurological disorders are diagnosed and treated.
- Integration of AI: Artificial intelligence's incorporation into MRI processing and analysis has the potential to significantly enhance image interpretation, aiding radiologists in more efficient and accurate diagnoses.
- Personalized Imaging Protocols: Future research may focus more on tailoring MRI protocols to individual patients, ensuring optimal imaging based on specific clinical needs.