Understanding Square Charges: Implications and Applications
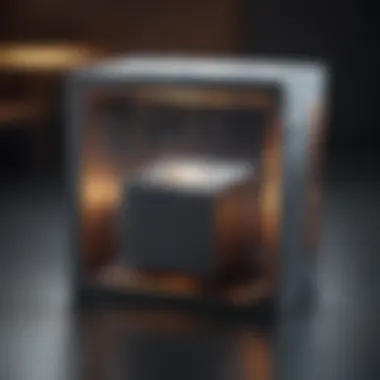
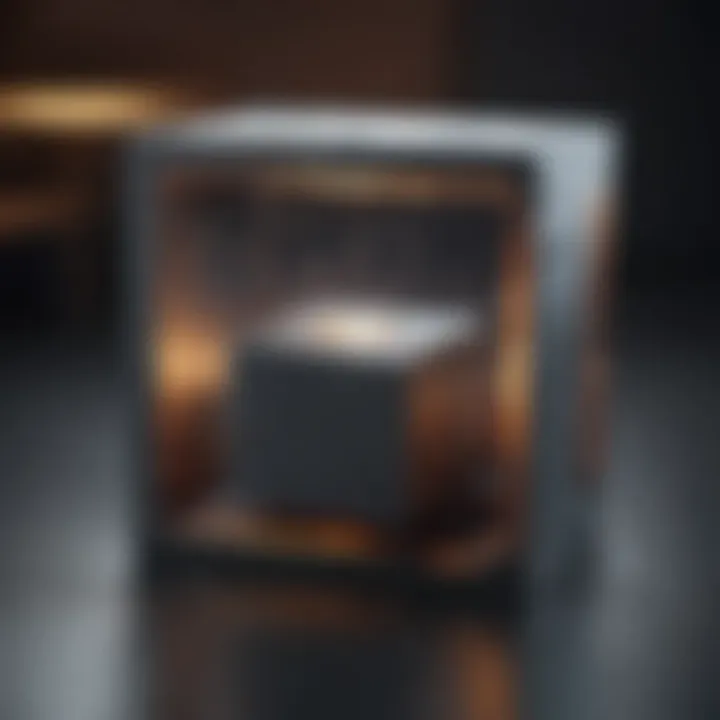
Intro
Square charges represent a fundamental concept within the realm of physics, particularly in the study of electrostatics. Understanding square charges involves delving into the nature of electric forces produced by charged objects under specific configurations. This article aims to unravel the complexity surrounding square charges and their implications in various scientific disciplines. The knowledge of square charges has practical applications not only in physics but also extends to fields such as biology and chemistry, where the principles of electrostatics play a pivotal role.
As we explore this topic, we will begin with an overview of existing research surrounding square charges. This foundation will allow us to dissect key findings, objectives, and hypotheses that drive ongoing investigations. Subsequently, we will examine the methodologies applied to study square charges, highlighting study designs and data collection techniques that enhance our understanding of this phenomenon. By the end of this article, readers will have a holistic perspective on square charges, their relevance, and their potential contributions to technological advancement and education.
Prelims to Square Charges
Square charges are a fascinating concept within the realm of physics, with far-reaching implications across various scientific disciplines. Understanding square charges is not just an academic inquiry; it holds essential insights for practical applications in fields like engineering, chemistry, and biology. Recognizing their importance allows scientists and researchers to harness their potential in innovative ways and to solve complex problems.
The significance of square charges begins with their foundational role in electrostatics. They serve as a crucial element for exploring electric fields and the interactions between charged particles. With the ability to model various real-world scenarios, understanding square charges equips students and professionals alike with the necessary tools to analyze systems involving electric forces.
A closer look at the complexity of square charges reveals their mathematical underpinnings. These charges can be graphed for better visual understanding, aiding comprehension for those navigating the theoretical aspects of physics. When well understood, square charge interactions can lead to technological advancements such as improved battery technology and energy efficiency.
Thus, the investigation into square charges establishes a strong basis for future research and innovation. Key points to consider include their definition, historical development, and the broader implications across scientific disciplines. Understanding square charges paves the way for an enriched comprehension of not only physics but also its applications in technology and education.
Definition and Conceptual Framework
Square charges, often denoted in physics as point charges, represent an idealized model for studying Coulomb's law. At their core, these charges convey a fundamental property of particles related to electric force. Specifically, a square charge implies a uniform distribution of electric charge along a two-dimensional plane, which simplifies the analysis within electrostatic contexts. Through thoughtful mathematical modeling, one can extract meaningful insights about interactions and field behavior.
To grasp the concept fully, it is vital to understand the surrounding framework of electrostatics. A key aspect is Superposition, which states that the total electric field created by multiple point charges equals the vector sum of the electric fields generated by each individual charge without interference. This principle greatly enhances our ability to calculate results across diverse scenarios, as complex electric fields can be broken down into manageable components.
Historical Context
The exploration of square charges is intricately tied to the advancement of electrostatics as a scientific discipline. The historical journey began with pioneers such as Charles-Augustin de Coulomb in the 18th century, who established foundational principles regarding electrostatic forces. His work laid the groundwork for understanding how like and unlike charges interact, setting a course for future research into charge distribution.
As science progressed, the introduction of mathematical models to represent charges became prevalent. The idea of square charges, though abstract, gained traction due to its ability to simplify complex electrostatic problems. By the early 20th century, greater advancements in electromagnetism and quantum mechanics further propelled the study of square charges within physics. Notably, the rise of computational methods enabled more precise modeling of charge interactions, opening up rich avenues for research not only in physics but also in interdisciplinary applications.
In summary, the historical context surrounding square charges reflects a gradual accumulation of scientific knowledge that has significantly shaped the landscape of modern physics. This evolution continues to inform current discussions and applications, making it essential for students and professionals to appreciate their relevance.
Mathematics of Square Charges
Mathematics serves as the backbone for understanding square charges. It provides the tools necessary to analyze and interpret the behavior of charges within different systems. The mathematics related to square charges is not merely theoretical; it has profound real-world applications that touch various disciplines. Insights gained from these mathematical frameworks help predict the interactions and effects of square charges in fields like physics, chemistry, and engineering. Understanding the mathematics of square charges enhances our ability to innovate technologies, improve efficiency, and contribute to scientific exploration.
Theoretical Foundations
The theoretical foundations of square charges are rooted in classical electrostatics. One begins with Coulomb's Law, which states that the force between two point charges is directly proportional to the product of the charges and inversely proportional to the square of the distance between them. In the case of square charges, we often consider charges arranged in a square configuration. The resultant forces acting on these charges must be calculated using vector summation. This complexity highlights the need for a solid mathematical grounding in vector calculus. Establishing a thorough understanding of these foundational principles lays the groundwork for further exploration into applications and innovations.
Unit Charge Calculations
Unit charge calculations involve determining the effects of a single unit charge within a system of multiple square charges. It is essential to understand how each charge influences others, affecting both magnitude and direction. The calculations involve evaluating fields created by combinations of charges and applying superposition principles. These principles allow for a systematic approach to resolving the net charge. By focusing on unit charges, researchers can simplify complex systems, making their study more manageable. This approach facilitates the analysis of potential energy and electric fields generated by square charge distributions.
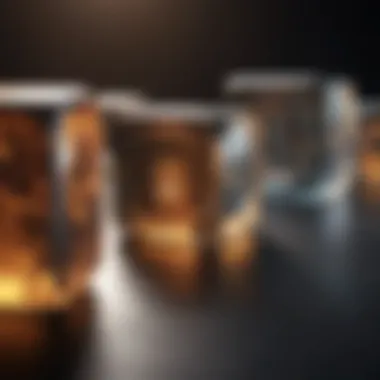
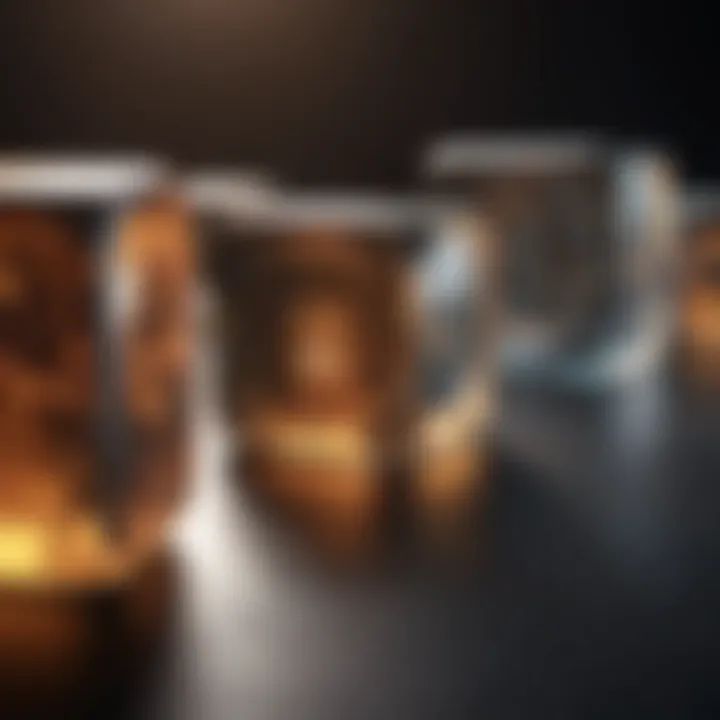
Graphical Representation
Graphical representation is a powerful tool in the study of square charges. Visualizing charge configurations helps in understanding the interactions between different charges. Graphs can illustrate electric field lines and equipotential surfaces, showing both direction and strength of the fields generated by the square charges. These representations allow for an intuitive grasp of spatial relationships and can reveal symmetry properties in charge distributions. Tools such as simulation software enhance this visual approach, enabling more detailed analysis and predictions based on mathematical models. Overall, graphical representations aid in bridging the gap between complex theory and practical understanding.
Understanding the mathematics of square charges is crucial for making informed advancements in scientific fields.
By delving into the mathematics of square charges, we equip ourselves with valuable knowledge. It not only enriches our scientific base but also propels innovation and exploration. The interplay of theoretical foundations, unit charge calculations, and graphical representations creates a comprehensive framework vital for an advanced grasp of square charges.
Applications of Square Charges
The study of square charges offers significant insights and practical applications across various scientific domains. The relevance of square charges cannot be understated, as they bridge theoretical concepts and tangible outcomes that drive advancements in multiple disciplines. In this section, we will explore their applications in physics, chemistry, and biology, focusing on the specific benefits and considerations involved in each field.
In Physics
In physics, square charges are a fundamental concept tied to electrostatics and field theories. The behavior of square charges under specific conditions provides crucial data for understanding larger physical phenomena. For instance:
- Force Calculations: Square charges can produce predictable force interactions which are essential for modeling electric fields. This helps in various applications, from designing circuits to understanding plasma behavior in astrophysics.
- Energy Storage: Square charge frameworks guide the design of capacitors and batteries, influencing efficiency and performance. This relationship has pushed innovations in energy storage systems, particularly under different operational conditions.
Moreover, the graphical representation of square charges helps visualize concepts, benefitting students and researchers alike. The clarity in the depiction of electric fields simplifies complex calculations and enhances instructional methods.
In Chemistry
In the realm of chemistry, square charges play a vital role in molecular interactions and reaction dynamics. Understanding how square charges influence molecular forces allows chemists to predict outcomes in various chemical processes:
- Ionic Compounds: The arrangements of square charges help rationalize the formation of ionic bonds, further aiding in the exploration of crystal structures and solubility.
- Reaction Mechanisms: Square charges clarify the electrostatic interactions between reactants, leading to a better understanding of reaction pathways and energy barriers.
Integrating square charges into chemical education provides students with a framework to better grasp foundational principles. Such integration leads to a more intuitive understanding of chemical bonding and molecular behavior, enriching the educational landscape in STEM.
In Biology
Square charges are also relevant in biological contexts, particularly in understanding cellular processes:
- Cell Membrane Dynamics: The charges associated with cellular components influence membrane potentials, critical for signal transduction and cellular communication.
- Protein Folding: The interaction among charged amino acids in proteins is essential for proper folding and function. The knowledge of square charges allows researchers to manipulate conditions that may lead to misfolded proteins involved in diseases.
As the biological implications of square charges surface, they highlight their necessity in advancing biochemistry and molecular biology. This relevance reinforces the integration of physics and chemistry to better understand complex biological systems.
"The investigations into square charges are not just about theory; they anchor practical solutions in science and technology."
Interdisciplinary Connections
The exploration of square charges offers significant insights across various scientific domains. Understanding how square charges operate facilitates connections not only within physics but also in disciplines such as material science and electrical engineering. This interconnectedness enriches the study of square charges, enabling researchers and practitioners to leverage insights from one field to innovate in another.
Square Charges and Material Science
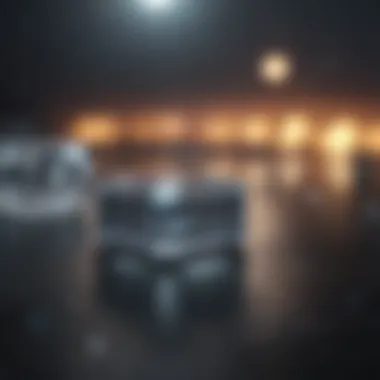
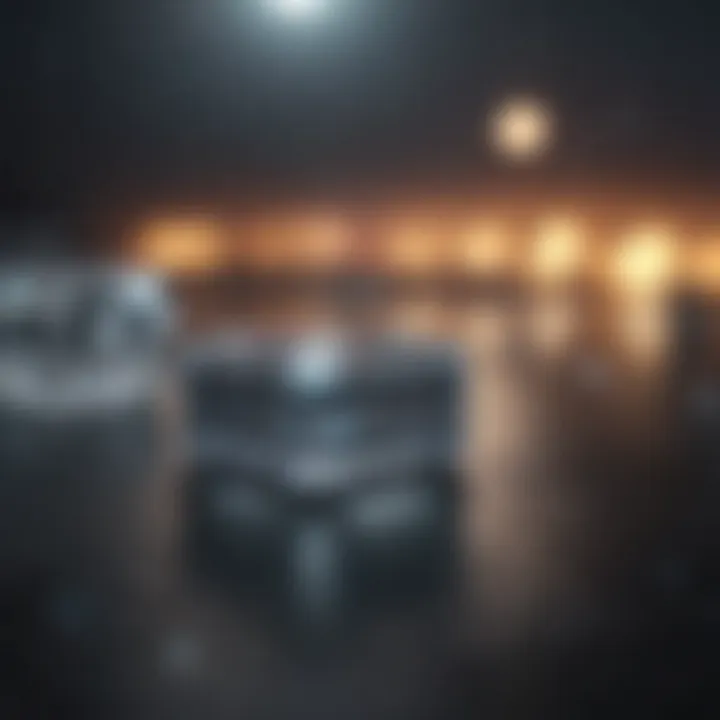
Square charges play an integral role in material science. They help explain phenomena related to the structure and properties of materials. For example, the arrangement of charges within a square can influence the electrical and thermal conductive properties of materials. Researchers examine how alterations in charge distribution can lead to enhanced or diminished performance in materials.
- Charge Distribution: The shape and alignment of square charges can affect how materials interact at atomic levels. Understanding these dynamics aids in designing better materials for specific applications.
- Development of Advanced Materials: By applying square charge principles, scientists can develop materials with tailored properties for electronics, such as superconductors or semiconductors.
- Characterization Techniques: The study involves various methods, such as spectroscopy and microscopy, to analyze how square charges influence material behavior under different conditions.
In sum, incorporating square charge theory into material science fosters a deeper understanding of material behaviors, leading to the discovery of novel applications.
Square Charges in Electrical Engineering
In electrical engineering, square charges are pivotal in understanding circuit designs and electronic component functionality. The principles governing square charges help to elucidate behavior in both passive and active components. Engaging with this concept can lead to advancements in designing efficient circuits and smart devices.
- Circuit Design: By studying square charges, engineers can optimize electrical circuits for performance and reliability. This would include minimizing energy loss due to suboptimal charge distributions.
- Component Design: Square charge analysis provides insight into the development of components like capacitors and resistors. Predicting how these components behave under different charge implementations improves overall system design.
- Signal Transmission: An understanding of how square charges propagate in circuits enhances signal processing technologies. Applications range from telecommunications to signal amplification.
Incorporating square charge theories allows engineers to maintain a competitive edge in innovation. The exploration of these connections between disciplines exemplifies the powerful impact of interdisciplinary studies.
Technological Implications of Square Charges
The study of square charges transforms various technological arenas, especially where electrical phenomena are involved. Square charges are instrumental in enhancing our understanding of fields such as material science and electrical engineering. They present unique challenges and opportunities that can lead to advancements in technology and energy utilization. Understanding the implications of square charges sheds light on future technological innovations and places emphasis on the need for ongoing research.
Innovations Driven by Square Charge Theory
Square charge theory has paved the way for several innovations across different sectors. In electronics, for instance, the realization of square charge behavior can improve the performance of capacitors and semiconductor devices. An understanding of this theory helps in designing more efficient electronic components that operate with reduced energy loss. With a growing emphasis on miniaturization in technology, square charges provide a framework for optimizing component geometries in nanotechnology.
Innovations are not limited to hardware; software algorithms that model square charge interactions also become more sophisticated. These developments enhance simulations and predictive models in scientific research. For example, in the context of designing batteries or energy storage devices, square charges can inform the arrangement and interaction of ions at the molecular level. By realizing these theoretical principles, researchers aim for breakthroughs in efficiency and energy storage capacity.
"Square charges in theory may lead to practical applications we have yet to fully explore. They are key to unlocking future technologies."
Among the notable innovations, consider the advances in photovoltaic cells. Understanding how square charges interact in materials can lead to cells that convert sunlight into electricity more effectively. Ongoing research in this area highlights the dual role of square charges, both as fundamental concepts and as practical assets that drive technological growth.
Efficiency and Energy Consumption
Efficiency and energy consumption are critical factors in today's technological landscape. Square charges play a significant role in enhancing both. By providing insights into charge distribution and field interactions, the theory aids in designing systems that minimize energy loss. The focus here extends beyond just theoretical applications; practical implementations are creating noticeable improvements in existing technologies.
In electric vehicles, for example, the application of square charge principles can optimize battery design and performance. By understanding how square charges behave under different conditions, manufacturers can create batteries with higher energy densities and improved life cycles. These advancements not only improve vehicle efficiency but also reduce overall environmental impact.
Furthermore, in the context of renewable energy systems, square charges inform the advancements in technologies like wind turbines and solar panels. These systems can benefit from improved designs that utilize the charge distribution behavior effectively, leading to reduced energy wastage.
- Understanding square charges leads to:
- Enhanced battery efficiency
- Improved performance of electronic components
- Optimization of renewable energy systems
The synergy between theoretical understanding and practical application of square charges in technology ultimately fosters a more sustainable future. Addressing efficiency and energy consumption through the lens of square charges ensures that upcoming technologies are not only innovative but also economically viable in the long term.
Educational Perspectives on Square Charges
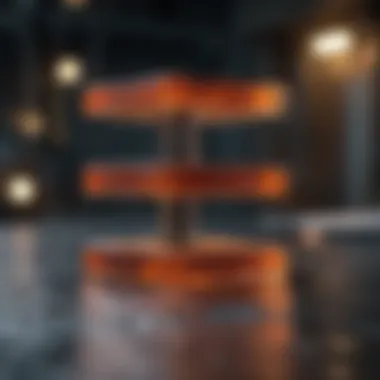
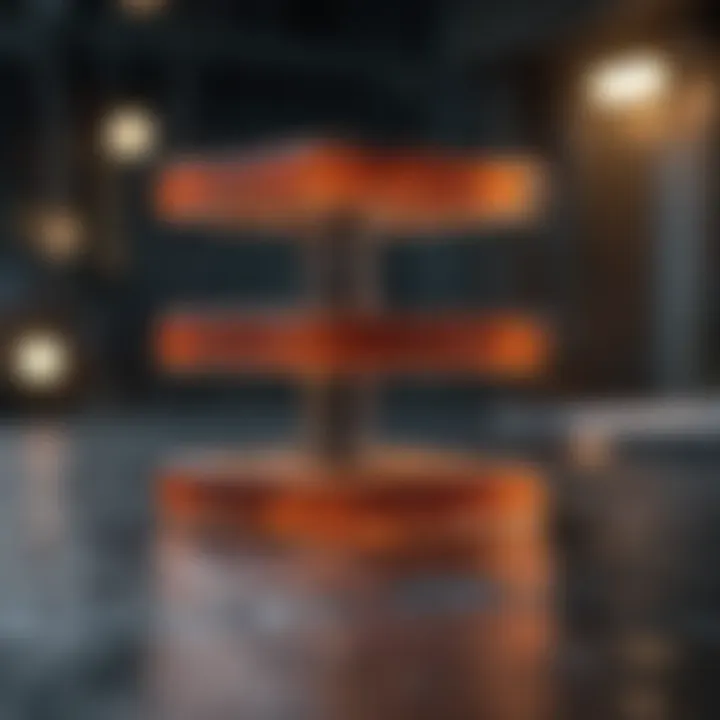
Understanding square charges is crucial in the context of science education. It enables students and professionals alike to grasp complex concepts in physics and beyond. Not only does it enhance critical thinking, but it also builds a stronger foundational knowledge base in various scientific disciplines.
Curriculum Development and Integration
Integrating square charges into the curriculum requires thoughtful planning. Educators need to develop structured syllabi that encompass the theoretical and practical aspects of this topic. Here are some considerations:
- Interdisciplinary Approach: Square charges intersect with physics, chemistry, and biology. A curriculum that highlights these connections can foster a more comprehensive understanding.
- Hands-On Activities: Practical applications and experiments can solidify theoretical knowledge. Laboratory experiments, simulations, and case studies can lead to deeper engagement.
- Adaptability: Education must evolve with new findings. Regular updates to curriculum content will help maintain relevance in a fast-paced scientific landscape.
Incorporating square charges not only enriches studentsβ knowledge but also sharpens their analytical skills. It prepares them for real-world applications in technology and research.
Teaching Methodologies
Effective teaching methodologies are paramount when discussing square charges in educational settings. Here are a few approaches:
- Interactive Learning: Use tools like digital simulations and interactive models to visualize square charges. This engages students and aids retention of complex concepts.
- Collaborative Learning: Foster group discussions and collaborative projects. These setups encourage peer-to-peer learning, facilitating diverse viewpoints.
- Problem-Based Learning: Present real-life scenarios involving square charges. This method cultivates problem-solving skills and enhances critical thinking.
Incorporating these methodologies into the teaching of square charges can create an enriching educational environment. It enhances not just understanding but also appreciation for the implications and applications of the concept in various fields.
Challenges in the Study of Square Charges
Understanding square charges requires grappling with various challenges that influence both research and practical applications. This section identifies key obstacles and insights that define the current landscape of square charge studies. Addressing these challenges enhances not only theoretical understanding but also practical implementations across scientific disciplines.
Research Gaps and Future Directions
The field of square charges faces notable research gaps, particularly in the integration of interdisciplinary methodologies. Many studies have focused on theoretical constructs but often lack empirical testing. This disconnect leaves significant questions unanswered regarding the practical applications of square charges in real-world contexts. A lack of comprehensive databases on experimental results restricts access to critical information necessary for advancement in the field. Researchers need to emphasize collaborative efforts across physics, chemistry, and engineering to bridge these gaps.
Moreover, future directions should involve a greater focus on developing sophisticated models that can better describe the behavior and interactions of square charges under various conditions. The theoretical implications could lead to advancements in material science, especially in developing new materials with finely-tuned electrical properties. In summary, making strides in this area will require academia and industry partnerships to effectively address gaps while pushing the boundaries of current knowledge.
Technological Limitations
Technological limitations present another significant barrier. Many existing tools and methodologies used to study square charges are not equipped to handle the complexity of modern applications. For instance, traditional measurement techniques may fall short in accuracy when applied to nano-sized structures. Additionally, many researchers rely on simulations that may not fully capture the dynamics of square charges in real-time scenarios.
To mitigate these limitations, investing in advancements in measuring technologies is crucial. Innovations such as atomic force microscopy and advanced computational modeling can enhance the understanding of square charge interactions. Furthermore, interdisciplinary approaches that mesh engineering with physics could lead to the development of better experimental setups or materials.
The End
The study of square charges encompasses significant implications across various scientific domains. Understanding their applicability allows researchers and practitioners to develop innovative solutions and enhance existing technologies. In this concluding section, we will outline the importance of the information covered in this article, focusing on specific elements and considerations regarding square charges.
Summary of Key Insights
- Multidisciplinary Relevance: Square charges play a notable role not only in physics but also in material science and engineering. Their understanding aids in exploring new materials and designing improved electrical systems.
- Mathematical Foundations: The theoretical aspects of square charges are anchored in sound mathematical principles. This foundation is crucial for advancing current technology and fostering research in various scientific areas.
- Technological Advancements: Innovations in various fields, such as renewable energy and electronics, are directly impacted by the application of square charge concepts. This underscores the importance of ongoing research in this area.
- Educational Implications: By integrating square charge theories into curricula, educators can cultivate a deeper understanding of complex scientific principles among students. This integration benefits future generations of scientists and engineers.
Final Thoughts and Implications
The future of innovation in technology relies heavily on the foundational understanding of concepts like square charges.
In the coming years, more research is needed to uncover the depths of this topic. Thus, both new and ongoing studies will be vital in addressing gaps in knowledge and maximizing the potential benefits of square charges.
This article serves as a comprehensive guide, highlighting the importance of square charges in modern science and their broader impacts.