Understanding RNA Types and Their Functions in Biology
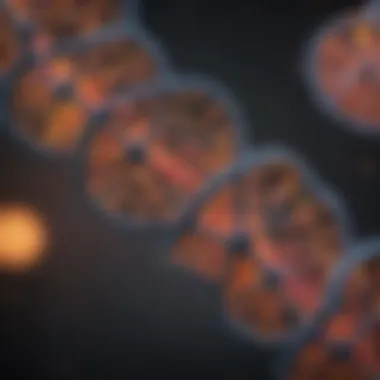
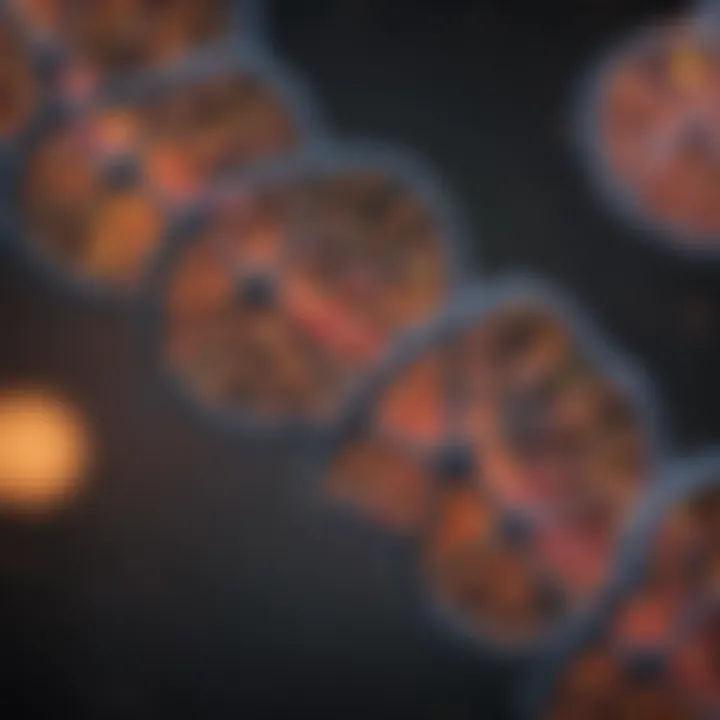
Intro
Ribonucleic acid, commonly known as RNA, serves as a crucial molecule within all living cells. Its multiple forms and diverse functions underpin many fundamental biological processes. Understanding RNA is essential not only for students and researchers within the biological sciences but also for anyone interested in cellular mechanisms and genetics. This article explores the essential types of RNA and their various roles in cellular activities, providing insights into messenger RNA, transfer RNA, ribosomal RNA, and non-coding RNAs. Each type of RNA contributes uniquely to the cellular milieu, influencing mechanisms from protein synthesis to gene regulation.
Research Overview
Summary of Key Findings
RNA is not a simple molecule; it is multifaceted with distinct types that perform specific tasks. Among these, messenger RNA (mRNA) is critical for conveying genetic information from DNA to the ribosome, where proteins are synthesized. Transfer RNA (tRNA) plays an essential role in translating this information, bringing amino acids to the ribosome during protein production. Ribosomal RNA (rRNA) forms the core of ribosome structure and catalyzes protein synthesis. Furthermore, various types of non-coding RNAs, including small interfering RNA (siRNA) and microRNA (miRNA), regulate gene expression and maintain cellular homeostasis.
Research Objectives and Hypotheses
This article aims to clarify the functions of different types of RNA. The key objectives include:
- Identifying the unique roles of mRNA, tRNA, rRNA, and non-coding RNAs.
- Exploring the mechanisms through which these RNAs contribute to cellular functions.
- Discussing the significance of RNA in both health and disease contexts.
The hypotheses posited are that understanding RNA types will facilitate insights into genetic regulation and potential therapeutic applications. This understanding could prove instrumental in the fields of biotechnology and medical research.
Methodology
Study Design and Approach
This article employs a comprehensive literature review approach. By synthesizing information from peer-reviewed studies, textbooks, and reputable online resources, a coherent narrative about RNA types is developed. Each RNA type is examined for its structure, function, and relevance in biological processes.
Data Collection Techniques
Data was gathered through various sources, including:
- Scientific journals and articles that address RNA research.
- Educational resources such as those found on en.wikipedia.org and britannica.com, expanding the understanding of genetic material.
- Community discussions from platforms such as reddit.com to gauge public interest and relevance in RNA research.
Through this structured approach, the article aims to convey a deep understanding of the inherent complexities tied to RNA and its significance in the broader context of organismal biology.
"RNA is integral to the operation of life itself, influencing everything from basic cell function to complex regulatory networks."
Prelims to RNA
Ribonucleic acid, commonly known as RNA, is a fundamental molecule in the realm of molecular biology. It plays various roles that are crucial for cellular function and overall organismal biology. Understanding RNA requires appreciating its diverse types and specific functions.
The significance of RNA lies in its essential contributions to processes such as protein synthesis and gene regulation. Each type of RNA carries out distinct tasks that enable cells to function, adapt, and respond to their environment. As such, RNA is not merely a byproduct of DNA expression but serves as a key player in cellular mechanisms, influencing everything from metabolism to signaling pathways.
In this article, we will explore several types of RNA, including messenger RNA, transfer RNA, ribosomal RNA, and non-coding RNAs. By dissecting the characteristics and mechanisms of each type, we aim to provide not just a description of their functions, but also an understanding of their overall importance in biological systems. This examination offers substantial insights for students, researchers, educators, and professionals alike, fostering a deeper appreciation for the intricate roles of RNA in the cell.
Definition of RNA
RNA, or ribonucleic acid, is a polymer made up of nucleotides, each containing a ribose sugar, a phosphate group, and a nitrogenous base. The primary function of RNA is to serve as a messenger between DNA and the ribosomes, where proteins are synthesized. Unlike DNA, which is double-stranded, RNA is typically single-stranded. This structural difference allows RNA to fold into complex shapes, enabling it to perform a wide range of functions in the cell.
The four types of nitrogenous bases that compose RNA are adenine, uracil, cytosine, and guanine. In RNA, uracil replaces thymine found in DNA. This slight variation allows RNA to play different roles in cellular processes, particularly in gene expression and regulation.
Historical Context
The study of RNA has a rich history that traces back to the early 20th century. Initially, RNA was recognized as a key component linked to protein synthesis. The concept gained momentum in the 1940s when researchers began to uncover the roles RNA plays in the transfer of genetic information. The discovery of messenger RNA in the early 1960s was pivotal; it acted as a crucial link between genes and the proteins they encode.
Later developments in molecular biology led to the classification of various RNA types, including transfer RNA and ribosomal RNA. These revelations advanced our understanding of the central dogma of molecular biology, illustrating how genetic information flows from DNA to RNA and ultimately to proteins. Over the years, research continues to unearth the diverse functions of RNA, particularly non-coding RNAs, that challenge the traditional views of gene expression and regulation.
Messenger RNA
Messenger RNA, often abbreviated as mRNA, serves as a vital component in the processes of gene expression and protein synthesis. Its role is fundamental to the biology of all living organisms. This type of RNA acts as a messenger that transmits genetic information from DNA to the ribosomes, where proteins are synthesized. Without mRNA, the instructions encoded in DNA could not be translated into functional proteins, which are essential for cellular structure and function.
Role in Protein Synthesis
The primary function of messenger RNA is to carry the genetic code from DNA, located in the nucleus, to the ribosome in the cytoplasm. This process begins with transcription, where an mRNA strand is synthesized using a DNA template. The resulting mRNA molecule undergoes several modifications before it becomes fully functional. Once matured, mRNA serves as the template for translation, a process where ribosomal RNA and transfer RNA work together to assemble amino acids in the correct sequence to form a protein. This highlights the crucial link between mRNA and the regulation of cellular activities through protein production.
Structure of mRNA
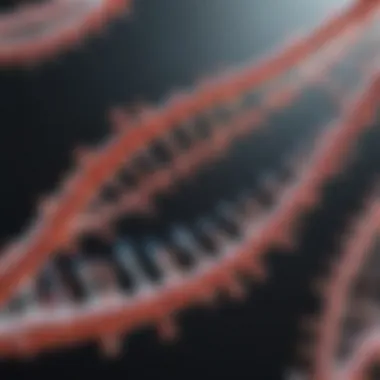
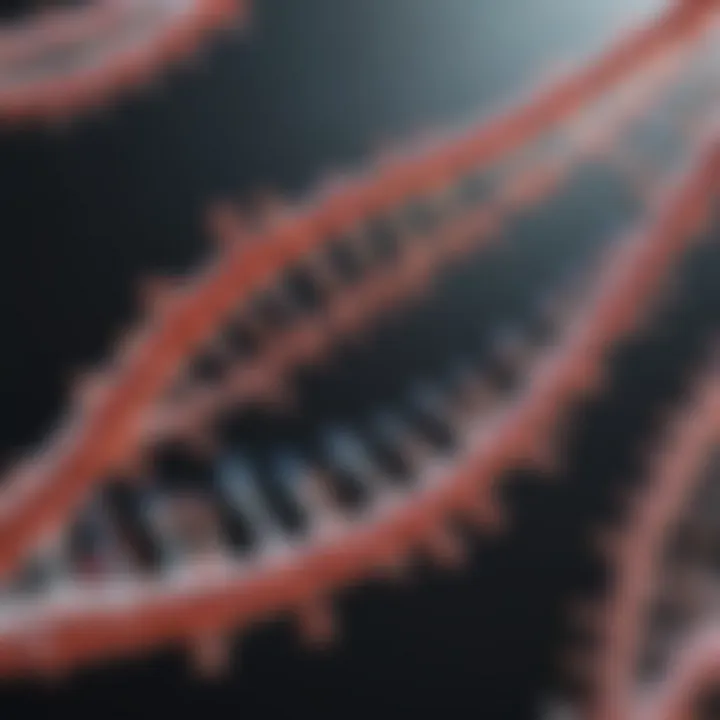
The structure of mRNA is crucial to its function. Typically, mRNA consists of a single-stranded nucleic acid chain, which includes a sequence of nucleotides. These nucleotides are composed of a sugar (ribose), a phosphate group, and one of four nitrogenous bases: adenine, uracil, cytosine, or guanine.
The mRNA molecule has a 5' cap at one end and a poly-A tail at the other end. The 5' cap plays a significant role in ribosome recognition and binding during translation, while the poly-A tail helps protect the mRNA from degradation and enhances its stability within the cell.
Post-Transcriptional Modifications
After transcription, mRNA undergoes important post-transcriptional modifications. These modifications improve the stability and translation efficiency of the mRNA. Some key modifications include:
- Capping: The addition of a 7-methylguanylate cap at the 5' end enhances mRNA stability and promotes ribosome attachment.
- Polyadenylation: The addition of a string of adenine nucleotides (the poly-A tail) at the 3' end aids in the export of mRNA from the nucleus and protects against enzymatic degradation.
- Splicing: Non-coding regions, called introns, are removed from the pre-mRNA transcript, while the coding regions, or exons, are joined together. This results in a mature mRNA ready for translation.
These modifications are crucial as they ensure that mRNA is functional for efficient protein synthesis, thus reinforcing its importance in the central dogma of molecular biology.
Transfer RNA
Transfer RNA, often referred to as tRNA, serves a fundamental role in the process of translation, where messenger RNA guides the synthesis of proteins. Without tRNA, the translation process would be inefficient and prone to errors, dramatically impacting protein production and cellular function.
Function in Translation
tRNA function is crucial for translating the genetic code into functional proteins. Each tRNA molecule is specific to one amino acid and carries it to the ribosomes, where proteins are built. This specificity ensures that the correct amino acid is incorporated into the growing polypeptide chain, matching the codons of the messenger RNA. The efficiency of protein synthesis depends heavily on the action of tRNA. Notably, the interaction between tRNA, mRNA, and ribosomes allows for the assembly of amino acids in the precise order dictated by the genetic code.
tRNA Structure and Anticodon
The structure of tRNA is uniquely adapted for its role in translation. A typical tRNA molecule has a cloverleaf shape, which is essential for its function. The three-dimensional structure consists of an acceptor stem where the amino acid attaches, and an anticodon loop that contains a sequence of three nucleotides complementary to the mRNA codons. This anticodon-codon pairing is crucial for accurate translation and ensures that the correct amino acid is added to the growing peptide chain. Each tRNA's unique structure helps identify its specific amino acid, demonstrating the sophisticated design of molecular interactions at work in the cell.
Amino Acid Attachment
Amino acids are linked to tRNA through a process facilitated by enzymes called aminoacyl-tRNA synthetases. These enzymes charge tRNA with the appropriate amino acid, ensuring that each tRNA molecule carries the correct one. This attachment occurs before translation. The protein synthesis later involves the sequential addition of these amino acids as determined by the mRNA sequence, ultimately building functional proteins. The fidelity of this process is paramount because the wrong amino acid can lead to nonfunctional or even harmful proteins.
Effective amino acid attachment is essential for the integrity of protein synthesis, highlighting tRNA's role in maintaining cellular health.
Ribosomal RNA
Ribosomal RNA (rRNA) is an essential component of ribosomes, the cellular machinery that performs protein synthesis. This type of RNA is not just a structural element; it plays crucial roles in the translation process and the overall functionality of the ribosome. Understanding rRNA is vital for various fields, including genetics and molecular biology, as it provides insight into how proteins, the workhorses of the cell, are assembled.
Components of Ribosomes
Ribosomes consist of two main subunits: the large subunit and the small subunit. Both of these subunits are primarily made of ribosomal RNA and proteins. The small subunit is responsible for reading the mRNA, while the large subunit facilitates the formation of peptide bonds between amino acids. In eukaryotes, the large subunit is called the 60S, and the small one is the 40S. In contrast, prokaryotes have 50S and 30S subunits, respectively. The presence of these multiple parts allows ribosomes to effectively translate the genetic code into functional proteins.
Role in Protein Assembly
The primary function of rRNA is to ensure correct assembly of amino acids into proteins. During translation, mRNA provides the sequence of nucleotides that determines the arrangement of amino acids. The ribosomal RNA helps match the mRNA codons with the appropriate tRNA which carries specific amino acids. The peptidyl transferase activity, primarily driven by rRNA, facilitates the formation of peptide bonds, linking amino acids. This means that rRNA is not merely a passive participant; it actively catalyzes the reaction, contributing to the formation of polypeptide chains.
Interactions with mRNA and tRNA
Ribosomal RNA has specific binding sites for both mRNA and transfer RNA. The mRNA binds to the ribosome at the entry site of the small subunit, and tRNA molecules enter the ribosome at the A site. Each tRNA has an anticodon that is complementary to the mRNA codon, ensuring that the correct amino acid is added. The exit site, or E site, then allows the tRNA to leave after its amino acid has been added to the growing polypeptide chain. These precise interactions are crucial for the accuracy and efficiency of protein synthesis.
The functional supremacy of rRNA in translation makes it a key focus area in understanding antibiotic action, as many antibiotics target bacterial ribosomes, inhibiting protein synthesis selectively.
Ribosomal RNA is fundamental for understanding not just how genes are expressed, but also how cells respond to their environments through protein synthesis. The intricacies of rRNA and its role in cellular processes can unveil potential therapeutic targets, making it invaluable in both basic research and applied biomedical sciences.
Non-Coding RNAs
Non-coding RNAs are significant players in the realm of molecular biology despite lacking the ability to code for proteins. Their roles vary widely, ranging from gene regulation to chromatin remodeling, making them crucial for maintaining cellular homeostasis. Understanding non-coding RNAs enriches our knowledge about genetic regulation and cellular function, highlighting their complexity in biological systems.
Overview of Non-Coding RNAs
Non-coding RNAs encompass various RNA molecules, including microRNAs, long non-coding RNAs, and small nucleolar RNAs. Unlike messenger RNA, which directs protein synthesis, non-coding RNAs have distinct functions that contribute to gene regulation and expression. Their lengths differ, but they all share the characteristic of not being translated into proteins. This segment has gained attention in recent years as research reveals how non-coding RNAs influence cellular pathways and adaptive responses.
Non-coding RNAs play critical roles in modulating biological processes and act as key regulators in diseases such as cancer.
Non-coding RNAs are classified mainly into:
- MicroRNAs: Short sequences about 20-22 nucleotides long, involved in regulating gene expression.
- Long non-coding RNAs: Longer transcripts that can span thousands of nucleotides, playing roles in gene expression regulation and chromatin organization.
- Small nucleolar RNAs: Primarily involved in the modification and processing of rRNA.
MicroRNAs and Gene Regulation
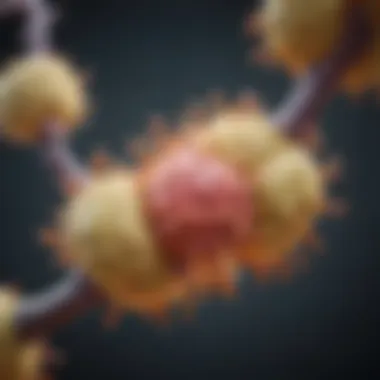
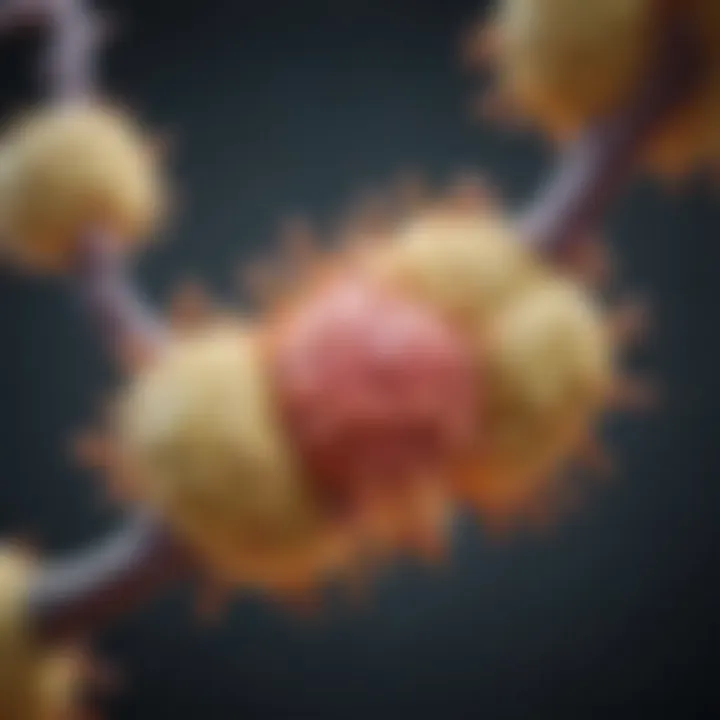
MicroRNAs are small, non-coding molecules that play essential roles in post-transcriptional regulation of gene expression. They bind to complementary sequences in messenger RNA, leading to degradation of the mRNA or inhibition of translation. This effectively reduces the amount of protein produced. The intricate process of miRNA biogenesis involves transcription by RNA polymerase II, followed by the formation of hairpin-like structures, and subsequent cleavage by the enzyme Dicer.
Their functions can modulate various cellular processes, such as:
- Development and Differentiation: MicroRNAs are vital in embryonic development and cellular differentiation.
- Homeostasis: They help maintain balance in metabolic processes.
- Diseases: Dysregulation of microRNAs can lead to cardiovascular diseases and cancers.
Long Non-Coding RNAs: Functions and Roles
Long non-coding RNAs are larger than microRNAs and have multifaceted functions in the cell. They can regulate gene expression at multiple levels, including transcriptional and post-transcriptional controls. Through various mechanisms, long non-coding RNAs can influence chromatin structure, thereby affecting the accessibility of transcription factors to DNA.
Some significant functions of long non-coding RNAs include:
- Gene Activation: They can act as scaffolds to recruit transcription factors to specific gene loci.
- Gene Silencing: Certain long non-coding RNAs can silence gene expression by binding to chromatin modification complexes.
- Behavior in Disease: Long non-coding RNAs have been implicated in various diseases, including neurodegenerative disorders and cancers.
Understanding non-coding RNAs is vital as they not only contribute to fundamental biological mechanisms but also serve as potential biomarkers and therapeutic targets in various diseases.
Small Nuclear RNAs
Small nuclear RNAs (snRNAs) are integral components of the eukaryotic cellular machinery, primarily involved in the splicing process of precursor messenger RNA (pre-mRNA). This function is crucial because it ensures that only mature mRNA is translated into proteins. Given the extensive role of protein synthesis in cellular functions, the study of snRNA reveals insights into gene expression regulation and RNA processing.
Function in Splicing
The primary role of small nuclear RNAs is in the splicing of pre-mRNA. Splicing is the process that removes introns (non-coding sequences) and joins exons (coding sequences) together to form a continuous mRNA molecule. This process is essential for the correct expression of genes.
The snRNAs function as part of the spliceosome, a large and complex molecular machine composed of snRNPs (small nuclear ribonucleoproteins) and various proteins. The snRNAs guide the spliceosome to the appropriate splice sites in pre-mRNA, facilitating the precise excision of introns.
The most studied snRNAs are U1, U2, U4, U5, and U6. Each has specific roles:
- U1 helps recognize the 5β splice site.
- U2 binds to the branch point within the intron.
- U4, U5, and U6 participate in the assembly and stabilization of the spliceosome.
This coordination among snRNAs leads to effective removal of introns and joining of exons. The inefficiency or errors during this process can lead to the production of faulty proteins which may result in various diseases.
Formation of Spliceosomes
The formation of spliceosomes is a dynamic and multi-step process. Initially, the snRNA components assemble with proteins to form snRNPs. The snRNPs then interact with pre-mRNA, leading to the activation of the spliceosome. This process can be broken down into several key steps:
- Recognition: The U1 snRNP binds to the 5' splice site; U2 binds to the branch point sequence in the intron.
- Complex Formation: The U4/U6/U5 tri-snRNP is recruited to form the complete spliceosome.
- Catalysis: The spliceosome undergoes significant conformational changes, enabling the removal of introns and connections of exons.
- Disassembly: After splicing, the spliceosome disassembles, allowing snRNAs and proteins to be recycled for future splicing events.
The intricate nature of spliceosome assembly emphasizes the essential role of small nuclear RNAs in ensuring the fidelity of gene expression. Their precise function underlies not only basic cellular processes but also broader implications in genetics and molecular biology.
The active role of snRNAs in splicing reflects their importance in maintaining the integrity of gene expression within the cell. Errors in this process can lead to serious diseases, including cancers and genetic disorders.
small RNA and Gene Silencing
The field of gene silencing is significantly impacted by small RNA molecules. These short sequences, typically 20 to 25 nucleotides in length, play a critical role in regulating gene expression. By understanding how these small RNAs function, researchers uncover pathways for new therapeutic approaches to complex diseases. The relevance of small RNA in gene silencing lies not only in its ability to modulate gene expression but also in its diverse mechanisms.
siRNA Mechanism
Small interfering RNA, or siRNA, is a vital player in gene silencing. The mechanism begins with the introduction of double-stranded RNA (dsRNA) into the cell. This dsRNA is recognized by an enzyme called Dicer, which cleaves the dsRNA into short fragments, creating siRNA.
The generated siRNA is then incorporated into a protein complex known as the RNA-induced silencing complex (RISC). Within this complex, siRNA unwinds, and the strand complementary to the target messenger RNA (mRNA) guides the complex to its specific mRNA target.
Once aligned with its target, RISC induces cleavage of the mRNA, resulting in its degradation. This degradation prevents translation into protein, effectively silencing the gene from which the mRNA was transcribed. The specificity and efficiency of this silencing process highlight the power of siRNA in controlling gene expression.
Role in RNA Interference
Small RNA plays a pivotal role in a broader process known as RNA interference (RNAi). RNAi is a cellular mechanism that regulates gene expression in response to foreign RNA, such as viral genomes, and also contributes to the regulation of endogenous genes.
In the context of small RNA and gene silencing:
- Pathogen Defense: siRNA mediates the response to viral infections by targeting viral RNA, thus protecting the host organism.
- Gene Knockdown: Researchers utilize siRNA in laboratory settings to decrease the expression of specific genes, aiding in the study of gene function.
- Therapeutic Applications: There is potential for using siRNA in clinical settings to target genes involved in diseases, including cancer and genetic disorders.
"The power of RNA interference lies in its ability to specifically target and silence genes, offering a profound tool for both research and therapy."
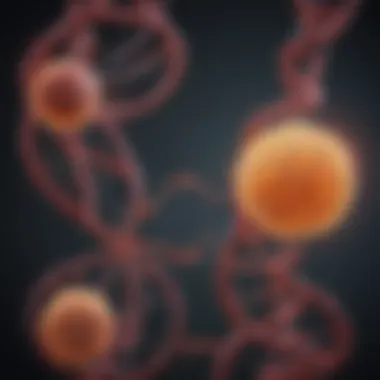
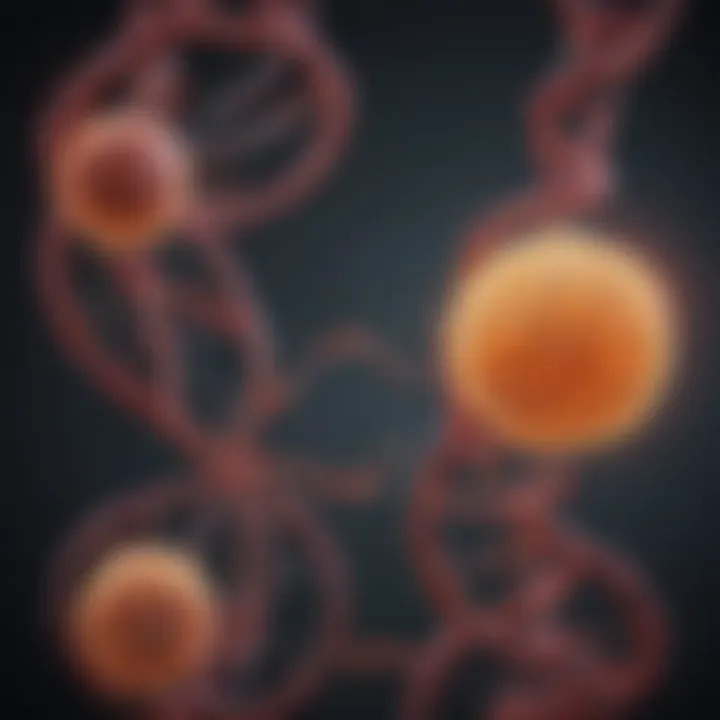
In summary, small RNA, particularly in the form of siRNA, is central to the mechanisms of gene silencing. Its importance in research and medicine continues to grow, further establishing the intricate relationship between RNA and gene regulation.
RNA in Regulation of Cellular Processes
Ribonucleic acid (RNA) plays essential roles in the regulation of cellular processes. It serves as a crucial intermediary in various mechanisms that control gene expression and cellular responses. Understanding how RNA contributes to these processes is vital for comprehending cellular functionality and the broader aspects of biology. This segment explores two significant areas: the influence on gene expression and the role in cellular response to stress.
Influence on Gene Expression
Gene expression is the process by which information from a gene is used to synthesize functional gene products, typically proteins. RNA is central to this process. Messenger RNA, or mRNA, carries genetic information from DNA to the ribosomes, where proteins are synthesized. However, other RNA types, such as microRNAs and long non-coding RNAs, also serve critical roles in regulating gene expression.
- MicroRNAs: These small, non-coding RNAs bind to mRNA molecules, leading to their degradation or repression. This prevents the translation of specific proteins, thus fine-tuning the expression levels necessary for cellular homeostasis.
- Long non-coding RNAs: These molecules interact with chromatin, influencing the accessibility of DNA to transcription factors. This modulation is critical in determining when and where genes are expressed.
"RNA modulates gene expression at multiple levels, providing a versatile mechanism for cellular regulation."
By influencing the stability and translation of mRNA, RNA helps maintain proper cellular function. Understanding these mechanisms provides insights into how cells adapt to environmental changes and maintain internal balance.
Role in Cellular Response to Stress
Cells encounter various stressors, which can be external, like temperature changes or toxins, or internal, such as DNA damage. The response to these stressors is essential for survival. RNA molecules are pivotal in modulating this response, particularly through changes in gene expression.
During stress, specific RNAs are upregulated or downregulated, demonstrating the plasticity of RNA in responding to challenges. For example:
- Stress Induced mRNAs: Some mRNA variants become more abundant when cells face stress, thus facilitating the production of stress-response proteins.
- Small RNAs: These molecules can silence genes that are no longer needed during stress responses, enabling cells to conserve resources.
In summary, RNA's role in the regulation of cellular processes is multifaceted. From influencing gene expression to reinforcing cells' ability to respond to stress, RNA's regulatory functions are crucial for maintaining cellular integrity and adapting to changing conditions. Understanding these roles enables researchers and professionals to explore therapeutic strategies targeting RNA-based functions.
RNA as a Therapeutic Target
The exploration of RNA as a therapeutic target has gained significant momentum in recent years. This development is largely attributed to the realization that various RNA species have critical roles in regulating gene expression and influencing cellular mechanisms. Targeting RNA offers new avenues for the treatment of diseases, particularly genetic disorders and cancers, where traditional approaches may be inadequate.
Therapeutic interventions that focus on RNA can potentially mitigate disease by modulating RNA interactions or correcting dysfunctional RNA molecules. The ability to specifically target RNA can lead to precise and efficient treatments, minimizing off-target effects commonly seen in traditional drug therapies. This specificity is crucial, given the complexity of the gene regulatory networks involved.
RNA-Based Drug Development
Developing RNA-based drugs involves several strategies that capitalize on RNA's biological functions. Among these, antisense oligonucleotides (ASOs) play a pivotal role. ASOs are short, synthetic strands of nucleic acids designed to bind to specific RNA sequences. By binding to target mRNA, they can inhibit its translation into protein, effectively silencing the expression of genes associated with disease.
Another noteworthy approach is RNA interference (RNAi), which utilizes small interfering RNA (siRNA) to degrade target mRNA transcripts. This method is instrumental in the context of diseases such as cancer, where overexpression of specific proteins drives pathogenesis. Recent advancements have paved the way for the use of RNAi in clinical settings, offering hope for previously untreatable conditions.
Development also includes the use of modified RNA molecules. These modifications can enhance the stability and effectiveness of RNA drugs while increasing their cellular uptake. Examples of such modifications include phosphorothioate linkages and 2'-O-methylation. The implications of these advancements extend to the optimization of delivery systems, ensuring that RNA therapeutics reach their intended targets efficiently.
Examples of RNA Therapeutics
The emergence of RNA therapeutics has led to concrete examples that underscore the potential of this approach. One prominent example is Nusinersen, which is an ASO used to treat spinal muscular atrophy (SMA). By targeting the SMN2 gene, Nusinersen modifies splicing to produce functional SMN protein, providing remarkable benefits to patients.
Additionally, Patisiran showcases RNAi technology's promise. This drug is used for treating hereditary transthyretin amyloidosis by silencing the production of transthyretin protein, thus addressing the root cause of the disease. Furthermore, Onpattro has emerged as a landmark achievement in utilizing siRNA for clinical treatment, providing a template for future RNA-based therapeutics.
The landscape of RNA therapeutics is rapidly evolving, with numerous candidates in various stages of clinical development.
These examples stress a transformative shift in therapeutic strategies and demonstrate the tangible benefits of targeting RNA. As research progresses, the likelihood of RNA therapeutics expanding into broader applications seems promising, making it an exciting frontier in modern medicine.
Finale
In this article, we comprehensively explored the various types of RNA and their fundamental functions. Understanding RNA is crucial, as it plays an essential role in cellular processes, influencing everything from protein synthesis to gene regulation. The types of RNA including messenger RNA, transfer RNA, ribosomal RNA, and non-coding RNAs are not just passive agents; they actively participate in complex biological mechanisms that sustain life.
Summary of RNA Functions
Each type of RNA serves distinct yet interconnected roles:
- Messenger RNA (mRNA) acts as the intermediary that conveys genetic information from DNA to ribosomes, where proteins are synthesized.
- Transfer RNA (tRNA) is vital in the translation process, transporting specific amino acids to ribosomes, ensuring they are added in the correct sequence during protein assembly.
- Ribosomal RNA (rRNA) forms the core of ribosome structure and function, facilitating the assembly of amino acids into proteins.
- Non-coding RNAs include a variety of molecules such as microRNAs and long non-coding RNAs, which regulate gene expression and contribute to cellular architecture.
The interplay among these RNA types underscores their collective importance in maintaining cellular integrity and function. Recognizing these roles helps in understanding more complex biological systems and evolving therapeutic strategies for diseases.
Future Directions in RNA Research
As RNA continues to unveil its secrets, several areas are ripe for exploration:
- RNA Therapeutics: The advent of RNA-based drug design, especially utilizing mRNA technology for vaccines, presents vast potential for combating various diseases.
- Gene Editing: Advancements in CRISPR technology and RNA interference open new pathways for precise gene modifications, aiming to rectify genetic disorders.
- RNA's Role in Cancer: Investigating the roles of non-coding RNAs in cancer biology can lead to novel diagnostic and therapeutic approaches.
"The future of RNA research lies not just in understanding its functions but in manipulating its pathways to foster advancements in medicine and biotechnology."
By focusing on these cutting-edge developments, researchers can leverage RNA's versatility, paving the way for innovative solutions in health and disease.