Understanding the Driving Range of Electric Cars
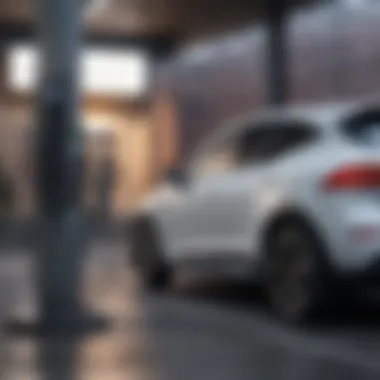
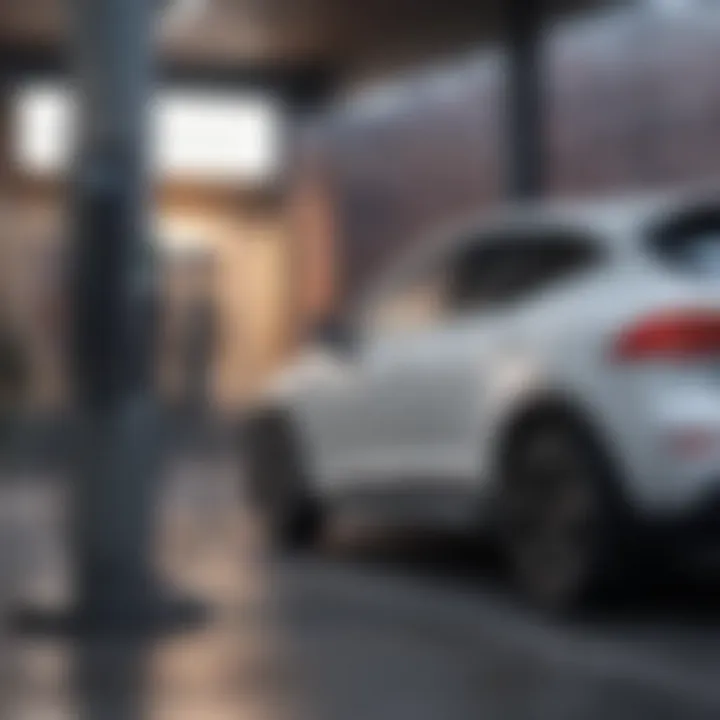
Intro
The driving range of electric cars plays a crucial role in shaping consumer decisions and the broader acceptance of this technology. It symbolizes mobility and convenience, directly tying into how individuals evaluate electric vehicles. A strong understanding of driving range parameters is essential for both potential buyers and industry stakeholders.
Electric cars utilize batteries to store energy. The capacity of these batteries, measured in kilowatt-hours (kWh), determines how far a car can travel on a single charge. However, driving range extends beyond just battery capacity. Factors such as energy consumption, climate conditions, and driving behavior also significantly influence how far vehicles can operate before needing a recharge.
This article provides insights into the intricacies of electric car range, focusing on ongoing advancements and the implications for consumers and enterprises alike.
Electric Vehicle Fundamentals
Understanding electric vehicles (EVs) requires a foundational grasp of their very essence. Electric vehicles are reshaping the automotive landscape, addressing both environmental concerns and the need for sustainable energy sources. Their importance in the context of this article is multifaceted. Recognizing how electric vehicles operate allows a deeper analysis of driving range and the considerations that accompany it.
A critical aspect of EVs is their reliance on electricity rather than fossil fuels. This shift not only reduces greenhouse gas emissions but also presents an opportunity to utilize renewable sources of energy. By understanding electric vehicle fundamentals, we can appreciate the intricacies of driving range, which directly ties to battery capacity, energy efficiency, and consumer behavior.
Definition of Electric Vehicles
Electric vehicles are defined as vehicles that are powered entirely or partially by electricity. This broad category encompasses a range of designs and technologies, all producing zero or low emissions during operation. The significance of this definition lies in its implication for understanding vehicle performance, especially in terms of range, which reflects how far an EV can travel on a single charge.
Types of Electric Vehicles
Electric vehicles come in various formats, each with its unique characteristics.
Battery Electric Vehicles
Battery Electric Vehicles (BEVs) operate solely on electric power stored in batteries. Their primary benefit is the absence of a combustion engine. This enhances their energy efficiency and reduces operational costs since electricity generally costs less than gasoline.
A key characteristic of BEVs is their capability for regenerative braking, which allows them to recover energy during stops. However, their driving range can be limited based on battery capacity, requiring an expansive charging infrastructure for consumer confidence.
Plug-in Hybrid Electric Vehicles
Plug-in Hybrid Electric Vehicles (PHEVs) combine an internal combustion engine with an electric motor. This duality allows for greater flexibility regarding driving range. Consumers can drive longer distances using gasoline when battery power is depleted, which makes PHEVs an attractive choice for those who may not have access to charging stations.
The main advantage is the balance between electric driving and the security of a gasoline engine. However, the reliance on gasoline can sometimes deter full commitment to the electric paradigm, creating a transitional mindset rather than a complete shift.
Fuel Cell Electric Vehicles
Fuel Cell Electric Vehicles (FCEVs) generate electricity through a chemical reaction between hydrogen and oxygen, primarily emitting water vapor as a byproduct. This technology has significant potential because it offers quick refueling times comparable to gasoline vehicles.
The major benefit of FCEVs is their long driving range and the ability to get refueled rapidly. Nonetheless, the limitations come from the scarcity of hydrogen fueling stations and the high costs associated with hydrogen production.
This diversity in electric vehicle types highlights the evolving landscape of electric mobility.
Understanding these fundamentals is crucial as they provide the basis for analyzing how various factors subject to technology influence the driving range and, ultimately, consumer choice.
Significance of Driving Range
Understanding the significance of driving range in electric vehicles is vital for several reasons. The driving range essentially dictates how far a vehicle can travel on a single charge. This metric is often a primary concern for potential electric vehicle buyers. A longer driving range can alleviate range anxiety, which is the fear of running out of battery power before reaching a charging station. Hence, driving range plays a crucial role in consumer confidence and overall acceptance of electric vehicles in the market.
Additionally, the driving range is not just about the numbers; it represents the intersection of technology and practicality. As electric vehicles become more advanced, the ability to extend driving range through improved battery technology and energy efficiency becomes increasingly important. Manufacturers that can provide a greater range tend to have a competitive edge, influencing consumer choices profoundly.
Moreover, the implications of driving range extend to infrastructure planning. As electric vehicle adoption rises, city planners and policymakers must consider charging station placements based on the achievable driving range of new vehicles. This consideration facilitates smoother integration of electric cars into urban environments, thereby fostering a thriving ecosystem for electric mobility.
Ultimately, the significance of driving range in electric vehicles encompasses consumer perceptions, technological advancements, and the broader context of urban planning and infrastructure. This multifaceted nature underscores the need to address driving range comprehensively in discussions surrounding electric vehicles.
Driving Range Explained
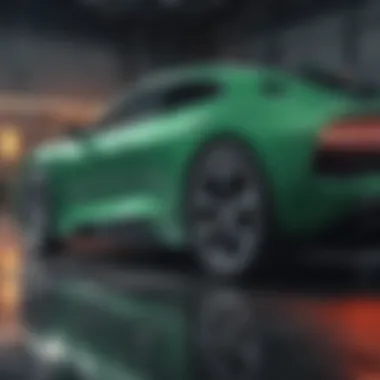
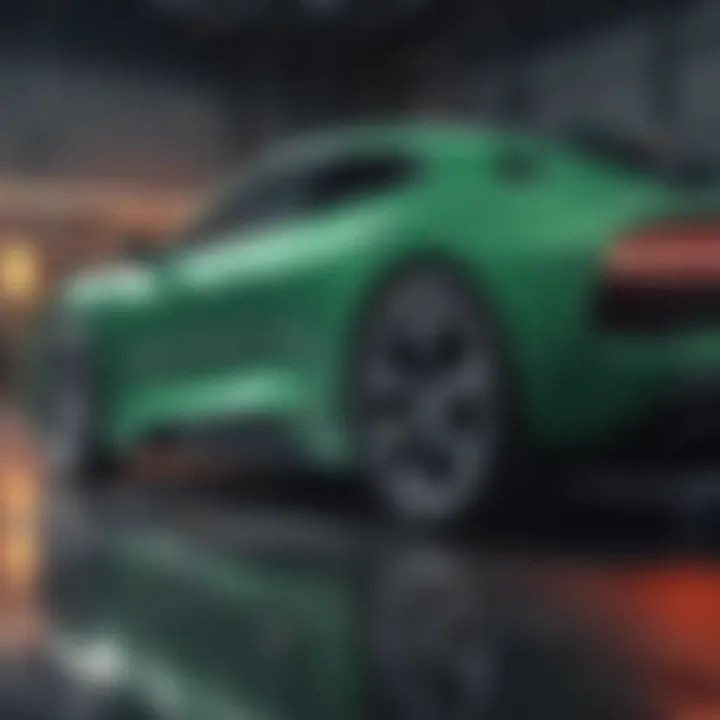
The driving range of electric vehicles refers to the maximum distance a vehicle can cover on a full battery charge. It is measured under standardized test conditions, which helps in offering a baseline for consumers to compare different electric models. Driving range may vary due to several factors, including battery capacity, driving style, and environmental conditions.
In general, battery capacity is often seen as the primary determinant of driving range. Larger capacities can potentially allow for longer distances. However, the efficiency of the vehicle's energy use also plays a significant role. Higher efficiency ratings can result in greater distance traveled even with moderate battery capacities.
It is crucial to recognize that while manufacturers provide estimated ranges, real-world performance can differ significantly. Conditions like driving speed, terrain, and use of climate controls can all impact how far the vehicle actually travels on a charge. Therefore, understanding the driving range requires looking beyond simple metrics to appreciate the variable conditions affecting it.
Consumer Perceptions and Concerns
Consumer perceptions heavily influence the driving range's significance in electric vehicle adoption. Many potential buyers still harbor skepticism towards electric vehicles due to lingering concerns about range limitations. This skepticism can manifest in hesitations regarding purchasing, especially for individuals accustomed to gasoline vehicles that offer longer ranges.
Surveys and studies indicate that range anxiety is a prevalent concern. Many consumers worry about whether they will find charging stations conveniently located along their routes, especially on long trips. These concerns contribute to the idea that electric vehicles may not be practical for some driving lifestyles.
Additionally, the understanding of driving range may vary across demographics, impacting buying trends. Younger consumers might be more willing to accept limited ranges, prioritizing sustainability, while older consumers might focus more on convenience and the dependence on a reliable driving range. The communications strategy used by manufacturers is also critical, as effective marketing can clarify misconceptions and highlight improvements in technology.
In summary, the significance of driving range extends beyond a mere number. It becomes a focal point of consumer concerns and perceptions, dictating purchasing decisions in an evolving automotive landscape.
Factors Influencing Driving Range
The driving range of electric vehicles is subject to various factors that can significantly affect the distance a car can travel on a single charge. Understanding these elements is vital, as they not only inform consumers about what to expect from their electric cars but also influence manufacturers in their design choices. Several key factors come into play when discussing driving range: battery capacity, energy efficiency, driving behavior, and external conditions. Each of these aspects contributes uniquely to the overall performance of electric vehicles.
Battery Capacity
Battery capacity is perhaps the most talked-about factor in driving range. Simply put, it refers to the amount of energy the battery can store, typically measured in kilowatt-hours (kWh). A higher capacity generally translates to a longer driving range. For example, vehicles like the Tesla Model S offer large battery capacities which allow for impressive ranges.
The larger the battery, the more power it can store, allowing the car to travel farther. However, it is essential to consider that larger batteries also add weight to the vehicle, potentially reducing range efficiency.
Energy Efficiency
Energy efficiency encompasses various components that determine how much energy the vehicle uses for propulsion. It is a crucial element in maximizing the potential driving range.
Motor Efficiency
Motor efficiency signifies how well an electric motor converts stored electrical energy into motion. A high-efficiency motor utilizes more of the energy for driving rather than losing it as heat. This characteristic is essential, especially in conditions where there are stop-and-go traffic scenarios.
Higher motor efficiency can be a beneficial trait for electric cars, encouraging design features that optimize energy use. However, developing efficient motors can be costly and may present challenges in production.
Vehicle Aerodynamics
Vehicle aerodynamics plays a critical role in reducing air resistance, allowing electric vehicles to glide through the air with less effort. A streamlined shape can enhance efficiency, which is crucial for extending the driving range.
Aerodynamic designs reduce drag and improve fuel economy. Cars such as the Tesla Model 3 exhibit adept engineering in this area, demonstrating how useful aerodynamics can be.
Tire Rolling Resistance
Tire rolling resistance relates to the energy required to keep the tires moving. Low-resistance tires lessen the energy consumed, contributing positively to the driving range. Selecting proper tires can have a noticeable impact on how far a vehicle can travel on one charge.
However, there is a trade-off; lower rolling resistance tires might compromise traction under specific driving conditions, which could present safety concerns.
Driving Behavior
The way drivers operate an electric vehicle can greatly influence its driving range. Aggressive acceleration and high-speed driving typically consume more energy, thus reducing the range. Conversely, smooth and steady driving habits can extend the vehicle's range.
Adopting eco-driving techniques can be beneficial for maximizing distance traveled. Techniques such as gradual acceleration, coasting, and gentle braking can increase range efficiency.
External Conditions
External conditions are another element affecting driving range. Numerous environmental factors can influence how far a car can go.
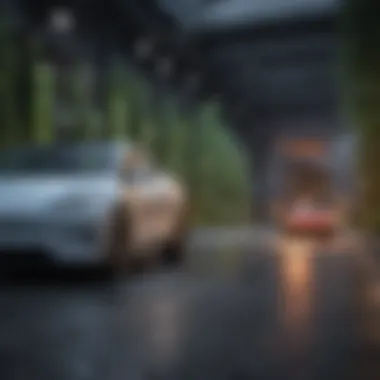
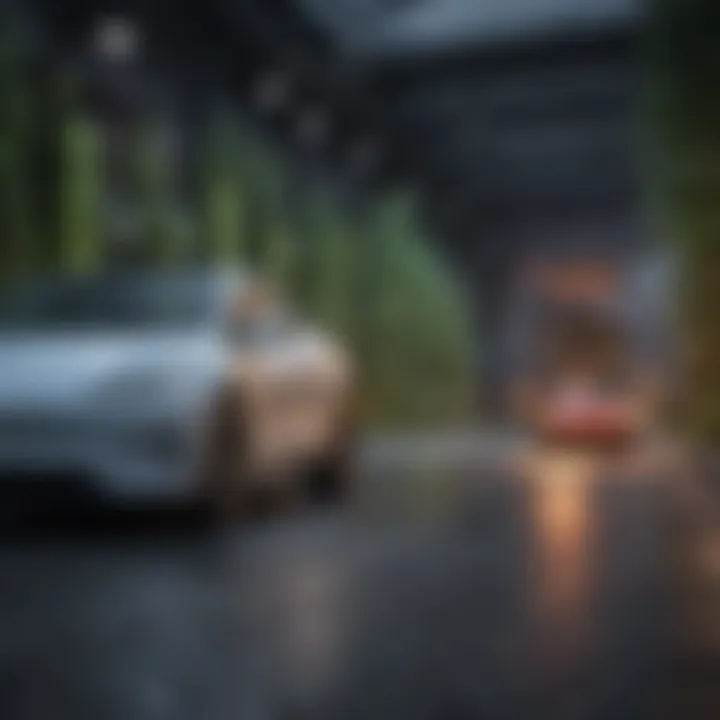
Temperature Effects
Temperature effects can alter battery performance and energy consumption. Cold weather can reduce battery efficiency, necessitating more energy for the same range. Conversely, moderate temperatures allow batteries to perform optimally. The impact of temperature on battery capacity can be significant, leading to variability in the expected range.
Terrain Impact
The terrain on which an electric vehicle operates also affects its performance. Driving uphill or on rough surfaces requires more energy. This increased demand on the battery reduces the overall driving range.
Flatter and well-maintained roads generally provide better range efficiency, whereas hilly or unpaved roads pose challenges.
Load and Weight
The weight of the vehicle, which can include passenger load and cargo, significantly impacts driving range. Heavier vehicles exert more demand on the battery, consequently reducing range. Therefore, understanding the load can help in planning trips and maintaining expectations.
Technological Enhancements
Technological enhancements play a crucial role in shaping the driving range of electric vehicles. As the market grows, innovations become essential for making electric cars more efficient and desirable. Understanding these advancements is key to assessing the future of electric mobility.
Advancements in Battery Technology
Solid-State Batteries
Solid-state batteries represent a significant leap in battery technology. Their structure replaces the liquid electrolyte used in traditional batteries with a solid electrolyte. This design enhances energy density, allowing for longer driving ranges. Another essential characteristic of solid-state batteries is their improved safety profile, as they are less prone to catching fire compared to liquid counterparts. These attributes make them a popular choice for manufacturers looking to improve range and reduce risk.
The unique feature of solid-state batteries is their potential for faster charging. Users could find themselves spending less time at charging stations while achieving more miles per charge. However, the development process is still in its early stages. Current production techniques can be costly and complex, posing challenges for widespread adoption. Nonetheless, their benefits position solid-state batteries as a promising candidate for the future of electric vehicle technology.
Improved Lithium-Ion Batteries
Improved lithium-ion batteries continue to dominate the electric vehicle landscape. Enhancements in materials and manufacturing processes have led to better performance. Key characteristics include higher energy density, which translates into longer driving ranges. These advancements make them a practical option for a variety of electric vehicles.
This type of battery stands out due to its relatively lower cost compared to emerging technologies. Additionally, advancements like silicon anodes are enabling greater energy capacity without dramatically increasing size or weight.
However, while improved lithium-ion batteries are more efficient, they still face some limitations. For instance, thermal management remains a concern, as they can overheat if not properly managed. Despite this, their widespread availability ensures they remain a critical component in the development of electric vehicle range.
Regenerative Braking Systems
Regenerative braking systems are another technological enhancement that impacts driving range. This system captures the energy typically lost during braking and converts it into usable electricity. This improves overall energy efficiency and extends the driving range significantly.
The primary advantage of regenerative braking is its ability to recover energy and reduce wear on brake components. This feature directly contributes to lower maintenance costs, providing additional incentives for electric vehicle users.
Overall, these systems are essential in the discussion of driving range, emphasizing the need for innovative solutions in overcoming the limitations of battery capacity. As electrification of transportation accelerates, understanding these technological enhancements is vital for making informed choices about future electric vehicles.
Evaluating Driving Range
Evaluating driving range is vital in understanding the performance and practicality of electric vehicles. The driving range determines how far an electric car can travel on a single charge, influencing consumer decisions and overall acceptance of electric cars in the market. If potential buyers perceive range as inadequate, it may deter them from choosing electric vehicles. Thus, evaluating driving range helps illuminate the strengths and weaknesses in electric car technology and offers insights into how these vehicles fit into daily life.
One key element in evaluating driving range is the adoption of standardized testing procedures. This provides a consistent framework for measuring and comparing the range of different electric car models. By using standardized methods, consumers can make informed decisions knowing that they are comparing apples to apples. Moreover, understanding real-world performance in relation to manufacturer claims can reveal gaps in expectations versus reality.
In this section, we will explore standardized testing procedures, focusing on EPA and WLTP testing. We will also analyze the differences between real-world range and what manufacturers claim, shedding light on how accurately these metrics inform consumers.
Standardized Testing Procedures
Standardized testing procedures are essential for providing clarity and consistency in the evaluation of electric vehicles. These procedures allow for reliable comparison between different models, helping consumers understand what to expect in terms of driving range. They also highlight advancements in electric vehicle technology.
EPA Testing
The Environmental Protection Agency (EPA) testing is a significant benchmark in the evaluation of electric vehicle ranges in the United States. EPA testing focuses on simulating real-world driving conditions to estimate the range under various circumstances.
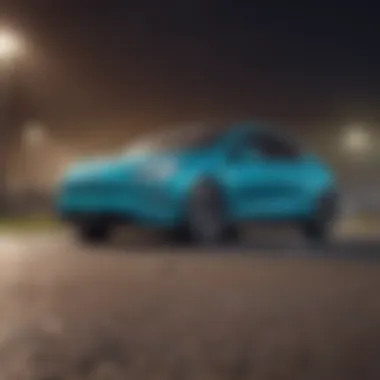
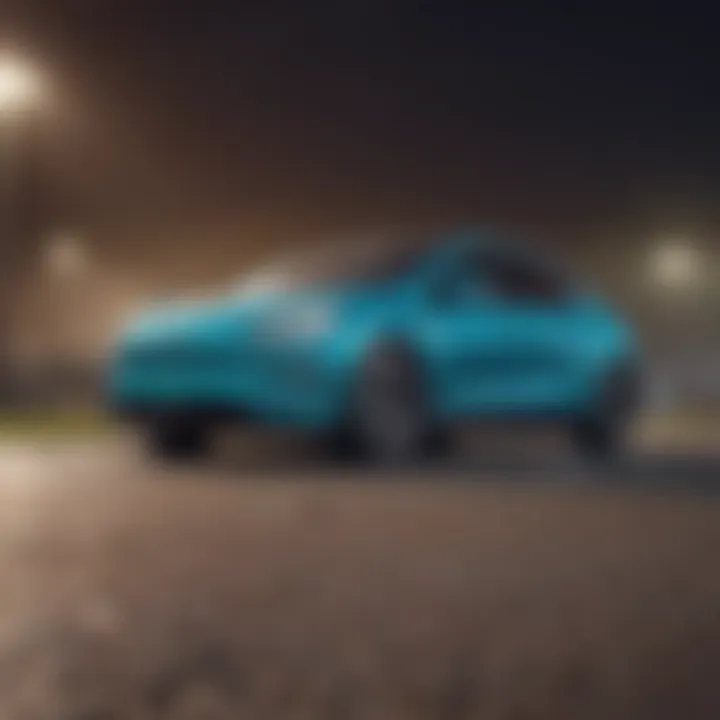
One key characteristic of EPA testing is its ability to provide two ratings: one for highway driving and one for city driving. This dual rating helps consumers gauge how range might vary in daily use. The unique feature of EPA testing is its combination of testing methods, including laboratory tests and software simulations, which add reliability to the estimates.
However, there are some disadvantages to consider. Some critics argue that EPA testing may not fully mimic every real-world scenario. For instance, it often does not account for extreme weather conditions or other variables that can impact driving range.
WLTP Testing
The Worldwide Harmonized Light Vehicles Test Procedure (WLTP) offers another standardized approach, widely recognized outside the United States. WLTP testing adopts a more dynamic driving cycle compared to the older NEDC protocol. This approach enhances the accuracy of range estimates by including a variety of speed profiles and conditions.
A key characteristic of WLTP testing is its ability to reflect real driving patterns more closely. This makes it a beneficial choice for understanding range, especially in Europe. The unique feature of WLTP is that it incorporates factors like air conditioning usage and additional weight from passengers or luggage, providing a more comprehensive evaluation of range.
Nevertheless, WLTP testing is not without its limitations. Some find that it may still not capture all real-world scenarios, particularly with hybrid vehicles where manufacturer-specific algorithms can influence efficiency.
Real-World Range vs. Manufacturer Claims
The contrast between real-world range and manufacturer claims is an area of crucial interest for consumers. Manufacturers often promote range figures that are achieved under optimal conditions, which can create unrealistic expectations.
Real-world conditions affect driving range significantly. Factors such as temperature, driving speed, and the use of accessories (like climate control) can all lead to deviations from manufacturer claims.
This section will delve deeper into case studies of various electric vehicles and how their real-world performance stacks up against advertised specifications. Understanding these discrepancies not only empowers consumers but also shapes future developments in electric vehicle technology.
Impact of Driving Range on Infrastructure
The driving range of electric cars significantly influences urban and rural infrastructure development. As the popularity of electric vehicles grows, it becomes essential to optimize the placement and accessibility of charging stations. This optimization ensures that drivers can easily recharge their vehicles, minimizing range anxiety. Without adequate infrastructure, even the most advanced electric cars might face adoption barriers.
Charging Station Placement
Proper placement of charging stations is vital for maximizing the utility of electric vehicles. Stations should be located strategically at high-traffic areas such as shopping centers, parking lots, and along highways, allowing for easier access. The following points highlight the considerations:
- Proximity to Main Roads: Stations closer to major routes help to alleviate concerns for longer trips.
- High Population Density Areas: Urban settings with a high number of potential electric vehicle users require more infrastructure.
- Partnerships with Businesses: Collaborating with retail and hospitality sectors to place charging stations can bolster customer satisfaction.
The effectiveness of charging station placement, however, is contingent upon thorough analyses of consumer patterns and preferences.
Fast Charging Technologies
Fast charging technologies are integral to reducing downtime for electric vehicle owners. Innovations like DC fast charging can supply significant amounts of electricity in short periods. The merits include:
- Reduced Charging Time: Fast chargers can restore up to 80% battery capacity in about 30 minutes, making long journeys more feasible.
- Increased Range Feasibility: A robust fast charging network enhances the practicality of electric cars for longer trips, boosting consumer confidence.
- Scalability: As technology advances, scaling the deployment of fast chargers becomes easier, accommodating an expanding electric vehicle market.
"The integration of fast charging networks is not just about speed, it is about establishing a reliable ecosystem for electric mobility."
This technological advancement influences how infrastructure is developed and integrated within urban planning, ultimately affecting user experience.
Future Trends in Electric Vehicles
The future of electric vehicles (EVs) is shaped not only by current advancements but also by anticipated trends that will redefine the landscape of transportation. Understanding these trends is crucial because they illuminate the direction in which EV technology is headed, inform consumer choices, and highlight the importance of investing in related infrastructure. As the electric vehicle market continues to evolve, significant elements such as emerging technologies and market predictions warrant close examination.
Emerging Technologies
Innovations play a pivotal role in the progression of electric vehicles. Key technologies that are on the horizon include:
- Solid-State Batteries: These batteries promise greater energy density, longer lifespan, and improved safety compared to traditional lithium-ion batteries. The move towards solid-state technology could significantly extend the driving range of electric vehicles, addressing a major consumer concern.
- Vehicle-to-Grid (V2G) Systems: This technology enables electric cars to not only draw power from the grid but also return excess energy when necessary. It could enhance grid stability and optimize energy use, turning EVs into assets rather than just energy consumers.
- Autonomous Driving Features: Integration of autonomous technology could lead to more efficient driving patterns, which would bolster energy utilization and thereby increase the effective driving range. These systems maximize the efficiency of power usage during operation.
Exploring these technologies reveals their potential to not only improve range but also create a more interconnected and efficient transportation system.
Market Predictions
Market forecasts indicate a promising future for electric vehicles. According to various studies, factors influencing this growth include:
- Increased Consumer Adoption: As battery technology continues to improve and prices decrease, more consumers are expected to choose electric vehicles over traditional combustion engine cars.
- Expanding Charging Infrastructure: Investments in charging networks are crucial for alleviating range anxiety among potential EV buyers. Predictions show that the number of fast-charging stations is set to rise, improving accessibility and convenience.
- Government Incentives and Policies: Many nations are setting ambitious electric vehicle sales targets. Support from governments through incentives and subsidies is likely to drive consumer interest and business investments.
"The transition to electric vehicles is part of a broader movement towards sustainable transportation, creating a ripple effect across various industries."
These elements paint a picture of a burgeoning electric vehicle market that will soon become more mainstream. A combination of advanced technology, consumer readiness, and supportive policies will affirm electric vehicles as critical components of future urban mobility. Understanding these trends equips stakeholders, from students to professionals, with crucial insights into the evolving world of electric mobility.