Exploring the Transformative Power of Nanotechnology
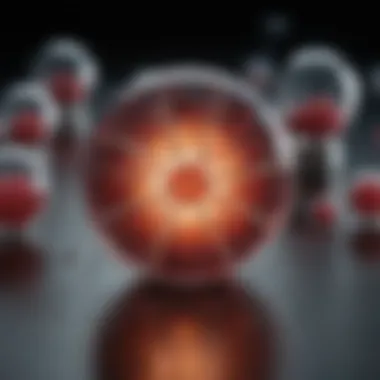
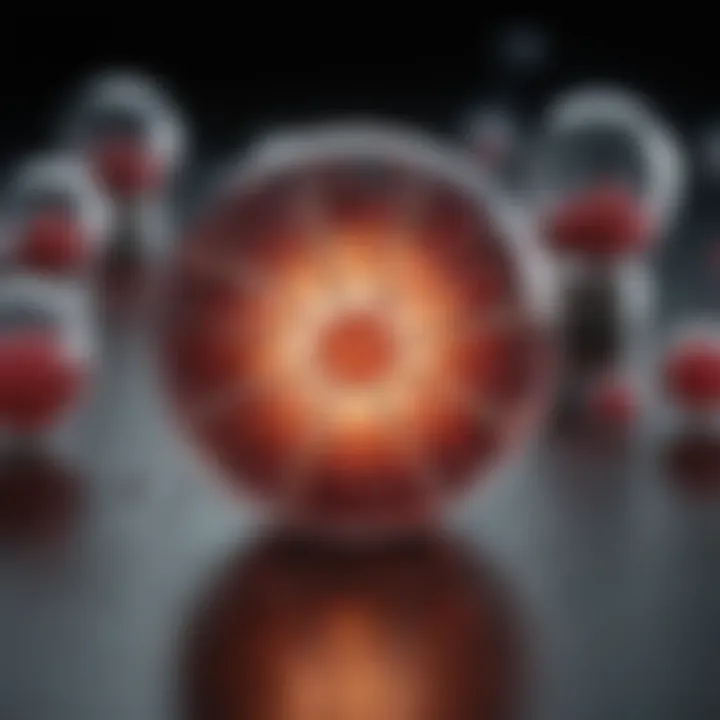
Intro
Nanotechnology has emerged as a pivotal field in contemporary science, merging the boundaries between multiple domains such as medicine, electronics, materials science, and environmental science. At its core, nanotechnology involves the manipulation of materials at the atomic or molecular level, often resulting in innovative solutions to longstanding challenges. This article delves into the various ways nanotechnology is reshaping our world, highlighting its significant advancements and assessing the benefits and challenges that accompany these developments.
In exploring the multifaceted applications of nanotechnology, this article aims to provide readers with a nuanced understanding of its relevance in modern society. Key discussions will revolve around how nanoscale innovations impact different sectors and the underlying principles of these technologies. As researchers and professionals from diverse backgrounds engage with these advancements, a critical assessment becomes necessary to balance the transformative potential of nanotechnology with the associated ethical and practical concerns.
This comprehensive examination of nanotechnology will enrich the understanding of its role in scientific and practical paradigms, catering to students, researchers, educators, and professionals alike.
Research Overview
Summary of Key Findings
Nanotechnology's practical applications span a wide array of fields. Notable findings include:
- Medical Advancements: Nanotechnology enhances drug delivery systems, enabling precise targeting of therapies while minimizing side effects. Theranostics, which merges therapeutics and diagnostics at the nanoscale, is also emerging as a significant breakthrough.
- Electronics Innovation: The electronic industry benefits from nanoscale materials that lead to better conductivity and efficiency in devices. The development of quantum dots and graphene for next-generation electronics exemplifies significant innovation.
- Materials Science: Nanotechnology contributes to the creation of lighter, stronger materials. These advancements are crucial in industries like aerospace and construction, where durability and weight are critical.
- Environmental Applications: Nanotechnology is proving effective in water purification, with nanoparticles aiding in the removal of contaminants. Additionally, it plays a role in renewable energy technologies, notably in solar cells with enhanced efficiency.
Research Objectives and Hypotheses
The primary objectives of this study include:
- To explore the transformative impact of nanotechnology on various industries.
- To delineate the benefits and challenges presented by these advances.
The following hypotheses guide this research:
- Nanotechnology significantly improves the efficacy and safety of medical treatments.
- Nanoscale materials lead to enhanced performance in electronic devices.
- The development of new materials at the nanoscale will contribute to sustainability in environmental technology.
Preamble to Nanotechnology
Nanotechnology is an area of study that focuses on the manipulation of matter at an atomic and molecular scale. This discipline delivers vast opportunities for innovation, impacting many areas such as medicine, electronics, materials science, and environmental science. As such, understanding the fundamentals of nanotechnology is essential for researchers, professionals, and anyone interested in the latest scientific advancements.
One of the most compelling aspects of nanotechnology is its ability to transform existing applications through the enhancement of material properties. By controlling matter on a nanoscale, scientists can develop new products that are lighter, stronger, and more efficient. Consider the advancements in medicine, such as drug delivery systems that utilize nanoparticles. This represents a significant leap in how healthcare can address complex diseases.
Moreover, the relevance of nanotechnology is underscored by its contribution to addressing global challenges, including environmental issues and energy consumption. As industries increasingly seek sustainable solutions, nanotechnology emerges as a front-runner in providing innovative paths forward.
Understanding key concepts in this field is crucial, especially as society increasingly relies on technology. These innovations will shape our future, affecting how industries function and how individuals interact with technology on a daily basis.
Definition and Historical Context
Nanotechnology refers to the science of manipulating materials at the nanometer scale, which is one billionth of a meter. The term gained prominence in the 1980s, although the concept dates back centuries with the use of engineered materials.
In the 1950s, physicist Richard Feynman delivered a seminal lecture titled "There's Plenty of Room at the Bottom," which envisioned the potential of manipulating matter at a molecular level. This idea laid the groundwork for modern nanotechnology, which has since evolved rapidly owing to advancements in imaging techniques and materials science.
Fundamental Principles of Nanotechnology
The fundamental principles of nanotechnology hinge on the unique physical and chemical properties exhibited by materials at the nanoscale. These properties differ significantly from those of bulk materials, often resulting in increased reactivity, strength, and conductivity.
One critical concept is the surface area-to-volume ratio. At nanoscale dimensions, particles have a much larger surface area relative to their volume, enhancing interactions with other substances. This property is pivotal in applications like catalysts and drug delivery.
Another important principle is quantum effects, which emerge at nanoscale and can lead to novel behaviors in materials. For instance, quantum dots, which are semiconducting nanoparticles, exhibit unique optical properties used in display technology.
"The manipulation of materials at the nanometer scale allows for innovations that were previously unimaginable, offering transformative solutions across diverse fields."
In summary, the introduction to nanotechnology not only elucidates essential concepts but also sets the stage for exploring its vast applications across various industries. By grasping the definition and historical context, as well as the fundamental principles, readers can appreciate the diverse impact of this field.
Nanotechnology in Medicine
Nanotechnology has found vital applications in the field of medicine, significantly enhancing the capacity for diagnosis and treatment. This technology focuses on manipulating materials at the nanoscale, leading to innovations that can provide more effective and targeted solutions in healthcare. The benefits of nanotechnology in medicine include improved drug delivery systems, advancements in diagnostic capabilities, and enhancements in therapeutic applications. Each of these areas plays a crucial role in transforming medical practices and patient care, making this a significant topic within the broader landscape of nanotechnology.
Drug Delivery Systems
Targeted Therapy
Targeted therapy is an innovative approach in medicine that utilizes nanotechnology to deliver drugs directly to specific cells or tissues. This method reduces side effects and enhances therapeutic efficacy. One key characteristic of targeted therapy is its ability to increase the concentration of drugs at the disease site while minimizing systemic exposure. This is particularly beneficial in conditions such as cancer, where traditional treatments can affect healthy tissues. The unique feature of targeted therapy allows for customizing medication based on individual patient needs and disease characteristics, which can be an advantage in achieving better outcomes. However, it may also pose challenges related to manufacturing complexity and regulatory approval processes.
Nanoparticles in Chemotherapy
Nanoparticles play a pivotal role in chemotherapy by providing a means to enhance drug solubility and stability. This approach allows for the formulation of chemotherapy drugs into nanoparticles, facilitating more effective delivery to tumor cells. A key characteristic of nanoparticles in chemotherapy is their localized action, which can lead to higher concentrations of the drug at the tumor site. This is a preferred choice due to its potential to minimize toxicity associated with conventional chemotherapy while maximizing effectiveness. However, the challenge lies in ensuring that nanoparticles can effectively evade the immune system and deliver the drug precisely to the targeted cells.
Smart Delivery Mechanisms
Smart delivery mechanisms represent a new frontier in drug distribution, making use of nanotechnology to release therapeutic agents in response to specific stimuli. These mechanisms can be programmed to respond to changes in pH, temperature, or other cellular signals, allowing for precise control over the release of medication. The key benefit of smart delivery mechanisms is their ability to optimize drug release profiles tailored to individual treatment requirements. This innovation improves patient compliance and treatment outcomes. Nonetheless, the development of smart delivery systems can be intricate, requiring extensive research and development.
Diagnostic Applications
Nanobiosensors
Nanobiosensors are devices that leverage nanotechnology to detect biological molecules or pathogens with high sensitivity and specificity. They are essential in facilitating rapid diagnostics, especially in disease outbreak scenarios. A key characteristic of nanobiosensors is their capability to detect markers at extremely low concentrations. This advantage makes them a beneficial choice for early disease detection and monitoring. The unique feature of nanobiosensors often involves their miniaturized size, enabling portable and easy-to-use diagnostic tools. However, there is a need for rigorous validation and standardization to ensure reliable results.
Imaging Techniques
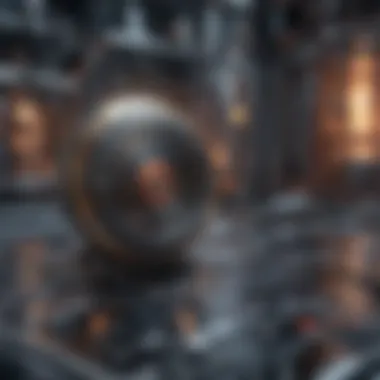
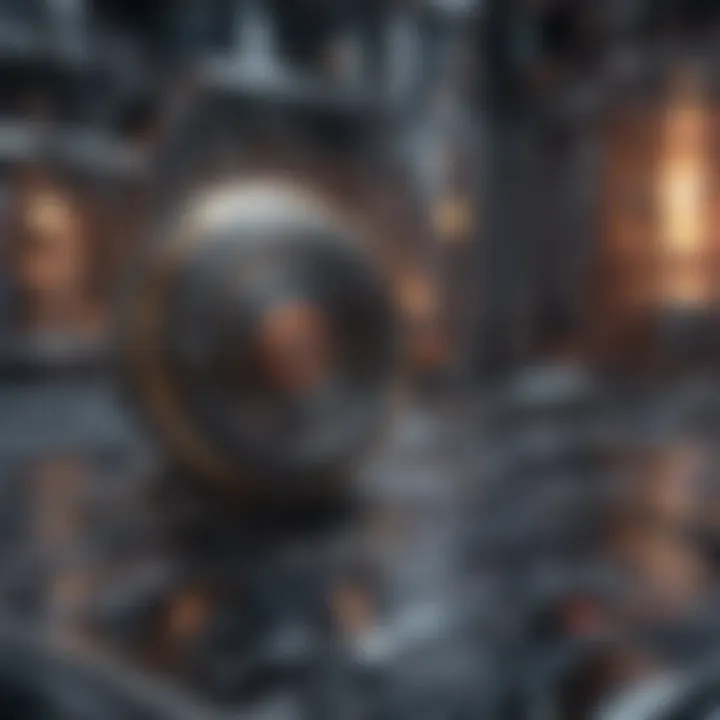
Imaging techniques enhanced by nanotechnology enable improved visualization of biological processes and structures at the nanoscale. This advancement contributes significantly to research and clinical diagnostics. A key characteristic of these techniques is their ability to offer higher resolution images compared to traditional imaging methods. This makes them a popular choice for detecting early signs of diseases. One distinctive aspect is the incorporation of nanoparticles as contrast agents, which enhances the imaging contrast significantly. However, concerns regarding the long-term bio-distribution of these nanoparticles are still being investigated.
Early Disease Detection
Early disease detection is critical for successful treatment outcomes, and nanotechnology plays a key role in this area. Techniques such as nano-enabled assays can identify disease markers before symptoms occur. A notable characteristic of early disease detection is its potential to shift healthcare from reactive to proactive approaches. This can greatly benefit patient outcomes and reduce healthcare costs. The unique aspect of these detection methods is their speed and accuracy, allowing for timely interventions. However, the accessibility of such technologies can be a barrier in some settings, necessitating increased efforts in education and resource allocation.
Therapeutic Applications
Nanoparticles for Gene Therapy
Nanoparticles in gene therapy offer a method for delivering genetic material into cells to alter disease processes at their source. This application has revolutionized how genetic diseases are approached. A key characteristic of nanoparticles for gene therapy is their ability to encapsulate and protect genetic material, facilitating its intracellular delivery. This is advantageous for targeting specific cells while decreasing off-target effects associated with traditional methods. The unique feature of nanoparticles enables tailored approaches to gene editing, although safety and ethical considerations must be navigated carefully.
Photothermal Therapy
Photothermal therapy utilizes nanoparticles that convert light into heat, effectively killing cancer cells. This therapy has emerged as a complementary approach to conventional methods. A key characteristic of photothermal therapy is its non-invasive nature, which minimizes damage to surrounding tissues. It is a beneficial choice due to its targeted approach, leading to less pain and quicker recovery for patients. The unique aspect involves the use of infrared light to excite the nanoparticles. However, there are challenges in optimizing light penetration and ensuring the equanimous distribution of nanoparticles within tumors.
Immunotherapy Enhancements
Immunotherapy enhancements using nanotechnology aim to strengthen the bodyβs immune response against cancer. The focus is on manipulating nanoparticles to deliver immune-modulating agents effectively. A key characteristic of immunotherapy enhancements is their capacity to promote longer-lasting immune responses compared to traditional treatments. This innovative approach holds promise as it can lead to more durable remissions for patients. The unique feature is the ability of nanoparticles to deliver compounds directly to immune cells, enhancing their activation. However, immunotherapy is still being studied for its long-term effects and potential toxicity.
Nanotechnology in Electronics
Nanotechnology plays a crucial role in the field of electronics by enabling significant advancements in performance, efficiency, and miniaturization. The ability to manipulate materials at the nanoscale allows engineers to develop electronics that are not only more compact but also exhibit superior functionality. This section will discuss key advancements in nanoscale transistors, quantum dots, and flexible electronics, highlighting their significance in modern technology.
Nanoscale Transistors
Performance Enhancements
The demand for faster and more efficient electronic devices has led to the development of nanoscale transistors. These components are integral for increasing the speed of data processing in electronic circuits. One of the key characteristics of performance enhancements in nanoscale transistors is their ability to operate at lower voltages. This results in less power consumption, which is particularly beneficial for mobile devices where battery efficiency is critical. The unique feature of these transistors lies in their capability to handle significantly higher densities of circuits on a chip, improving overall performance while minimizing energy usage. However, the miniaturization of transistors can sometimes lead to challenges such as increased heat generation and complexity in manufacturing.
Miniaturization Trends
Miniaturization trends have transformed the electronics landscape, allowing for the creation of smaller devices that possess greater capabilities. The trend toward smaller transistors results in chips that can house millions, if not billions, of individual circuits. This scaling provides notable benefits, including the reduction of material costs and an increase in the speed of devices. A unique feature of these trends is their alignment with consumer demands for portability and efficiency. Nonetheless, as transistors shrink, there also arises a set of engineering challenges, such as quantum tunneling effects, which can affect performance.
Quantum Dots
Applications in Displays
Quantum dots have emerged as a potent tool in display technology, especially in televisions and monitors. Their unique property of emitting specific colors based on their size allows for enhanced image quality and color accuracy. A critical characteristic of applications in displays is their ability to produce shades that are more vibrant when compared to traditional display technologies. This feature makes quantum dots a popular choice in LCD and OLED screens, offering better energy efficiency and lifespan. However, the integration process can be complex, and manufacturing costs can be higher, impacting large-scale adoption.
Photovoltaic Technologies
Another significant application of quantum dots is in photovoltaic technologies. These tiny semiconductor particles are effective in converting sunlight into electricity. Their main advantage lies in their versatility; they can be tuned to absorb a wider range of the solar spectrum. This characteristic enhances overall energy conversion efficiency, making them a promising option for renewable energy solutions. However, challenges remain regarding stability and production costs, which could hinder widespread implementation.
Flexible Electronics
Conductive Nanomaterials
Conductive nanomaterials have opened new avenues in the development of flexible electronics. These materials, which maintain high conductivity while being lightweight and bendable, are crucial for next-generation devices. A significant characteristic of conductive nanomaterials is their ability to maintain performance while allowing for the design of flexible circuits and displays. This flexibility is particularly beneficial in wearable technology and innovative medical devices. Yet, the challenge remains in ensuring durability and performance under mechanical stress.
Applications in Wearable Tech
The rise of wearable technology has significantly benefited from advancements in flexible electronics. These applications allow for the creation of devices that can conform to the human body, providing seamless interaction and functionality. A key feature of applications in wearable tech is their potential for real-time health monitoring, enabling users to track vital signs and performance metrics effortlessly. This functionality represents a significant step forward in personal healthcare. However, issues related to comfort, power supply, and data security are ongoing considerations that need addressing in future designs.
Nanotechnology in Materials Science
Nanotechnology plays a significant role in materials science, showcasing profound impacts on how materials are developed and utilized. The manipulation of materials at the nanoscale brings about unique properties that are not observed in their bulk counterparts. This enhanced functionality fosters innovations that are crucial for various applications across industries including automotive, aerospace, and consumer electronics. With the increasing demand for high-performance materials, nanotechnology serves as a pivotal force in addressing current and future challenges.
Enhanced Material Properties
Strength and Durability
Strength and durability are paramount when considering material applications. Nanotechnology enhances these characteristics through various mechanisms, such as improving the microstructure of materials. Nanomaterials can distribute stress more evenly, making them less prone to fracture. This characteristic is especially beneficial in applications where safety and longevity are critical. For example, in the aerospace sector, stronger and durable materials help reduce weight without compromising safety.
Another unique feature of enhanced strength is its contribution to developing lighter vehicles that also meet stringent safety standards. However, one must consider potential trade-offs. Increased strength sometimes correlates with higher costs in production or difficulty in processing, which can pose challenges in widespread adoption.
Lightweight Composites
Lightweight composites become essential in markets that prioritize efficiency, particularly in transportation and construction. The integration of nanotechnology in composites enables a significant reduction in weight while maintaining structural integrity. This property leads to energy savings, especially in vehicles, where every gram counts for fuel efficiency.
The key characteristic of lightweight composites is their high strength-to-weight ratio. These materials are popular choices for numerous applications because they reduce overall weight without compromising functionality. A notable feature is their ability to absorb energy, which helps in safety applications.
Despite these advantages, there are considerations. The manufacturing processes for lightweight composites can be more complicated and expensive compared to traditional materials. This factor can hinder their adoption in less economically developed sectors.
Nanocoatings
Self-Cleaning Surfaces
Self-cleaning surfaces often utilize nanotechnology to repel dirt and water through specific surface modifications. This aspect contributes significantly to maintenance reduction in various applications, from buildings to electronics. The ability to self-clean enhances the longevity of materials, reducing the frequency and necessity of cleaning processes.
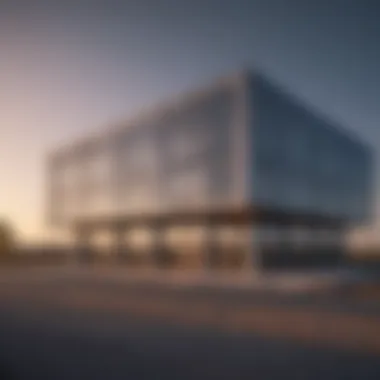
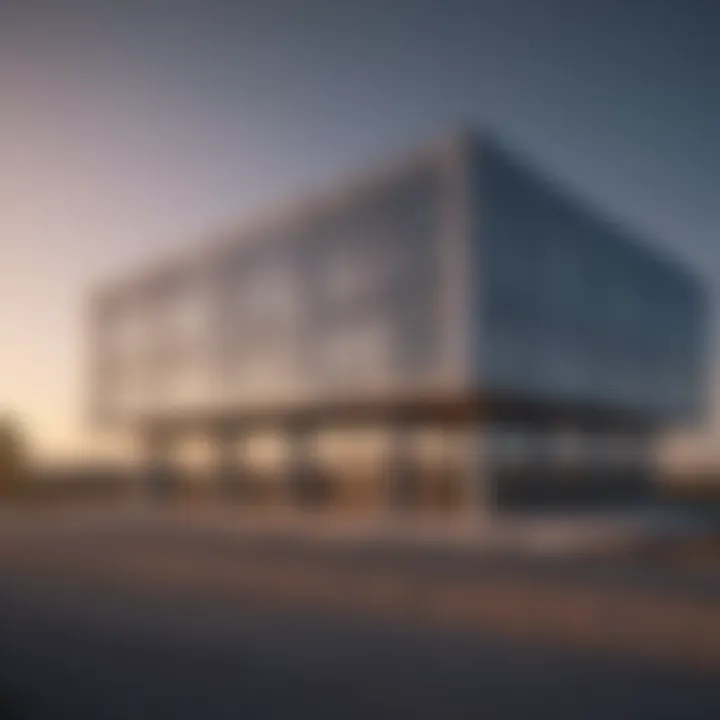
The primary characteristic of self-cleaning surfaces is their ability to maintain cleanliness without human intervention. They are particularly beneficial in environments where hygiene is critical, such as in healthcare settings. A unique feature of these surfaces is their hydrophobic properties, which effectively allow dirt and particles to be removed with rain or simple rinsing.
However, considerations include the potential for decreased performance over time due to wear and tear. Self-cleaning surfaces may require reapplication or treatment after prolonged exposure to harsh conditions, which can incur additional costs.
Anti-Corrosion Properties
Anti-corrosion properties of nanotechnology-enhanced coatings provide significant benefits in protecting materials against environmental factors. This is essential in industries such as construction and marine applications where exposure to moisture and chemicals is common. The nanocoatings create a barrier that prevents corrosive agents from reaching the base material, extending its lifespan substantially.
The strong characteristic of anti-corrosion coatings is their ability to maintain structural integrity over time. They are advantageous choices for maintaining the aesthetic and functional aspects of materials. A unique feature is the reduction of maintenance costs since materials require less frequent replacements.
Nevertheless, some considerations should be noted. The effectiveness of anti-corrosion coatings can vary based on the environment they are in, potentially necessitating tailored solutions for different applications.
Smart Materials
Thermochromic Materials
Thermochromic materials change color in response to temperature fluctuations. This characteristic is harnessed in various fields, from fashion to safety applications. Their ability to visually indicate temperature changes can enhance safety measures, particularly in environments where extreme changes occur.
The key aspect of thermochromic materials is their responsiveness to heat, providing clear visual cues that can enhance user interactions. These materials are beneficial for applications such as temperature-sensitive packaging. A unique attribute is that they can be integrated into existing products without significant redesign, making them appealing to manufacturers.
However, there are downsides, such as limited temperature ranges for effective coloration. Additionally, over time, exposure to certain conditions may affect their responsiveness, impacting reliability in critical applications.
Shape-Memory Alloys
Shape-memory alloys possess the remarkable ability to return to a predetermined shape when heated. This characteristic is influential in robotics, medical devices, and actuators. The adaptability of these materials facilitates design flexibility and operational efficiency.
The main feature of shape-memory alloys lies in their unique response to thermal stimulation, allowing them to perform mechanical tasks. This has been a popular choice for applications in aerospace, where lightweight yet strong materials are essential. Moreover, their versatility opens innovation pathways across multiple sectors.
Nonetheless, it is important to consider limitations in terms of fatigue resistance. Over time, repeated cycling may lead to a decrease in performance, warranting careful analysis in longevity and reliability for critical applications.
To summarize, nanotechnology in materials science greatly influences various industries through the development of advanced materials with enhanced characteristics that are crucial for the future. The integration of these technologies opens new frontiers for innovation and efficiency.
Nanotechnology in Environmental Science
Nanotechnology is playing an increasingly significant role in environmental science. It offers innovative solutions to major challenges we face today, such as pollution control and sustainable energy. By utilizing nanoscale materials and processes, scientists can develop techniques for mitigating environmental damage and enhancing eco-friendliness. This section discusses how nanotechnology contributes to pollution remediation, energy solutions, and soil health.
Pollution Remediation
Nanoscale Adsorbents
Nanoscale adsorbents are materials engineered at the nanoscale to capture pollutants from air or water. Their small size provides a larger surface area that enhances interaction with contaminants. This characteristic allows them to effectively trap heavy metals, organic compounds, and other pollutants. In environmental applications, nanoscale adsorbents are beneficial because they can be tailored to target specific contaminants, making them versatile tools in pollution cleanup efforts.
A unique feature of nanoscale adsorbents is their ability to regenerate. After capturing pollutants, they can often undergo processes to release the contaminants, allowing for reuse. However, there are disadvantages, including the potential for leaching nanoscale materials back into the environment if not properly handled.
Nanoparticles for Water Purification
Nanoparticles provide innovative methods for water purification by targeting different types of pollutants. These particles can disrupt bacterial membranes or assist in the breakdown of organic pollutants at lower concentrations than traditional methods. Their high reactivity is a key characteristic that makes them essential for advanced purification systems.
One exclusive feature of nanoparticles in this context is their ability to work in diverse water conditions, including turbid or chemically complex environments. This adaptability makes them a significant choice in many purification applications. However, their usage may raise concerns over reliability and the stability of nanoparticles under varying conditions, requiring ongoing research to address these challenges.
Sustainable Energy Solutions
Nano-enhanced Solar Cells
Nano-enhanced solar cells incorporate nanomaterials to improve efficiency and performance over traditional solar technology. They often facilitate better light absorption and increase the conversion efficiency of sunlight into electricity. A notable characteristic of these cells is their thinner structure compared to standard options, reducing material costs while enhancing flexibility and functionality.
One significant advantage is that nano-enhanced solar cells can be integrated in various applications, from building-integrated photovoltaics to portable devices, thus widening their use. However, initial costs and market acceptance still pose challenges within this sector.
Energy Storage Improvements
Nanotechnology is transforming energy storage solutions, particularly with batteries and supercapacitors. It allows for materials that can hold a greater charge and charge more quickly. The distinctive feature of these improvements is the enhancement of energy density without increasing weight.
This advantage is essential as society shifts towards renewable energy systems that require efficient storage mechanisms. There are disadvantages linked with the longevity and recycling of nanomaterial-enhanced storage systems, which necessitates thorough investigation to ensure sustainability.
Nanotechnology in Soil Science
Nanofertilizers
Nanofertilizers are designed to deliver nutrients more effectively than conventional fertilizers. Their small particle size enables them to penetrate soil and reach plant roots more efficiently. A key characteristic is that they can reduce the quantity of fertilizer needed, minimizing chemical runoff in agricultural settings.
This technology can lead to a more sustainable approach to agriculture by optimizing nutrient delivery, reducing environmental impact. However, potential risks include the unintentional effects nano-sized materials may have on soil health and local ecosystems, warranting a cautious advance in their application.
Nanosensors for Soil Health
Nanosensors are tools capable of providing real-time data on soil conditions, such as moisture levels and nutrient availability. This ability to gather precise information offers farmers the chance to optimize their irrigation and fertilization strategies. A crucial characteristic of nanosensors is their sensitivity, which allows for early detection of soil health issues before they escalate.
The integration of such technology can enhance agricultural productivity while promoting sustainability. Nonetheless, the reliance on these sensors may lead to over-dependence on technology, which poses a long-term concern for traditional practices of farming.
"Nanotechnology is shaping the future of environmental solutions by offering innovative advances that enhance efficiency and sustainability across multiple sectors."
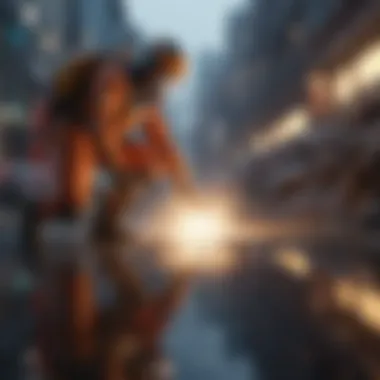
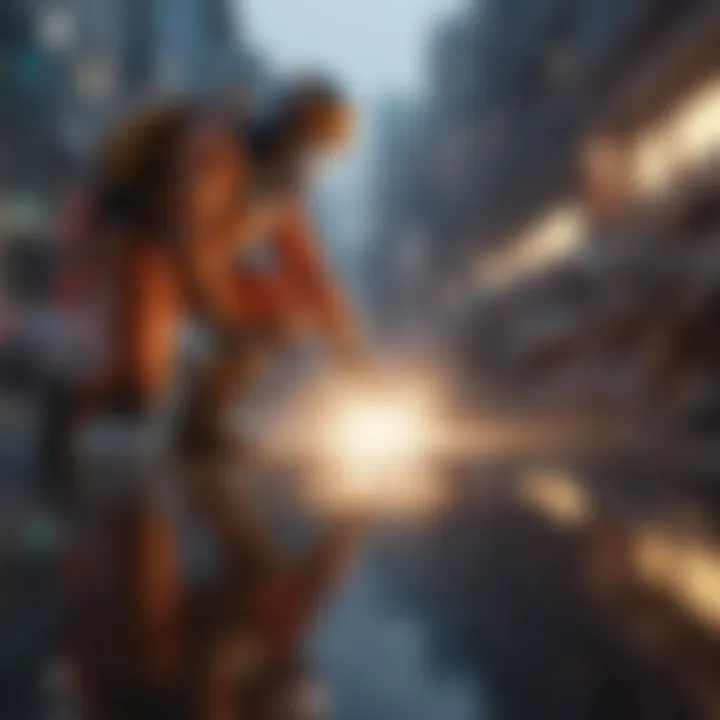
Ethical Considerations and Risks of Nanotechnology
Nanotechnology presents significant opportunities and advantages in various sectors; however, it also brings about considerable ethical considerations and potential risks. These concerns are essential to address, as they influence not only public perception but also the direction of future research and regulation. The implications of deploying nanotechnology can extend to health, environmental sustainability, and the ethical treatment of technology itself. Exploring these dimensions is critical for ensuring responsible advancement in this innovative field.
Health and Safety Concerns
Health and safety concerns around nanotechnology must be considered seriously. These worries mainly stem from the unknown effects that nanomaterials can have on human health. As materials are manipulated at a nanoscale, their behavior can differ significantly from their bulk counterparts. For instance, nanoparticles can penetrate biological barriers, potentially leading to unintended exposure.
Toxicological Assessments
Toxicological assessments are crucial in determining the safety of nanomaterials. This process involves evaluating the potential toxic effects these materials may have on human health and the environment. The key characteristic of toxicological assessments is the methodical examination of the interactions between nanomaterials and biological systems.
These assessments are a beneficial component of the research process, allowing scientists to understand risks before widespread application of nanotechnology. One unique feature of toxicological assessments is their reliance on specialized methods that address the unique properties of nanoparticles. However, there are challenges. For example, standard testing methods may not fully capture the risks associated with new nanomaterials, leaving gaps in knowledge that need further investigation.
Environmental Impacts
The environmental impacts of nanotechnology remain an area of concern. Nanomaterials can enter ecosystems and potentially disrupt existing environmental balances. A significant aspect of understanding these impacts is evaluating how nanoparticles interact with soil, air, and water systems.
The prominent characteristic of focusing on environmental impacts is identifying both beneficial and harmful outcomes. While nanotechnology has the potential to improve pollution remediation and energy efficiency, it could also contribute to novel forms of pollution. A unique feature of this discussion is the duality of benefits and challenges. Understanding these aspects is vital for developing strategies that prioritize sustainable use.
Regulatory Frameworks
Regulatory frameworks play a central role in managing the risks associated with nanotechnology. They provide necessary guidelines to ensure that nanomaterials are developed and applied safely. An effective regulatory framework promotes innovation while protecting public health and the environment.
Policy Development
Policy development is critical for creating a comprehensive approach to nanotechnology regulation. This process involves charting out the guidelines and legal structures that govern nanomaterials' development and use. A key characteristic of policy development in this area is the collaboration between scientists, lawmakers, and industry stakeholders to create informed regulations. This being a beneficial approach enhances regulatory effectiveness and public trust.
Unique to this aspect is the continuous evolution of policies as new scientific findings emerge. However, the dynamic nature of technology can outpace regulatory efforts, posing a challenge.
Global Standards
Global standards for nanotechnology provide a framework for consistent regulation across different countries. These standards are essential for ensuring that nanomaterials are managed effectively, irrespective of their geographical application.
The primary characteristic of global standards is their ability to facilitate international cooperation and trade. They aim to promote safety and efficacy while harmonizing regulations worldwide. However, developing universally accepted standards is complex due to variations in regulatory environments and scientific understanding among nations. Navigating these differences remains a challenge that must be addressed to ensure the safe integration of nanotechnology into various sectors.
Future Trends and Innovations in Nanotechnology
The field of nanotechnology is rapidly evolving, and its future trends indicate a significant potential for reshaping industry practices and emerging processes. As researchers develop more sophisticated tools and methods, there is increasing optimism about the impact of these advancements on various sectors. Understanding these trends is crucial for recognizing the possibilities that nanotechnology brings.
Emerging Technologies
Graphene and Beyond
Graphene is a single layer of carbon atoms arranged in a two-dimensional lattice. It has exceptional electrical conductivity, mechanical strength, and thermal properties. These attributes make it a focal point in nanotechnology research. In this article, graphene is highlighted for its role in numerous innovations, from advanced batteries to touching nanoelectronics. Its ability to enhance conductivity leads to better performance in electronic devices, presenting a compelling advantage in rapidly evolving tech environments. However, the production of high-quality graphene at scale remains a challenge that could impact its long-term feasibility.
Advancements in Nanomedicine
Nanomedicine is an expanding sector that utilizes nanomaterials for medical purposes. Key characteristics of advancements in nanomedicine include improved drug delivery systems and innovative therapeutic approaches. For instance, targeted treatments using nanoparticles can minimize side effects and increase the effectiveness of drugs. This specificity is beneficial for patients, making treatments less disruptive. Nonetheless, there are ongoing concerns about long-term effects and biocompatibility of nanoparticles in human bodies, which must be addressed through rigorous research.
Cross-disciplinary Applications
Integration with Biotechnology
The convergence of nanotechnology with biotechnology leads to exciting developments. This integration enables advancements in areas like genetic engineering and bioimaging. The key characteristic of this synergy is the enhanced precision in manipulating biological systems at the nanoscale. By using nanotechnology, researchers can develop better diagnostic tools and targeted therapies. However, ethical concerns regarding genetic modifications may present challenges that require careful consideration and regulation.
Nanotechnology in Telecommunication
Nanotechnology's role in telecommunication is becoming increasingly relevant. For example, nanoscale materials can enhance signal transmission and antenna design. The unique features of these materials allow for more robust and energy-efficient communication systems. As data consumption continues to soar, these innovations are critical for meeting growing demands. However, issues surrounding data security and privacy in a nanoscale framework must be resolved to ensure public trust and readiness for adopting these advancements.
"Nanotechnology is at a stage where it promises to transform industries but also needs a framework to address the challenges that lie ahead."
In summary, the future trends and innovations within nanotechnology hold significant promise and potential challenges. From graphene to nanomedicine, the applications are numerous and varied.
As more is understood about these technologies, it is crucial that advancements are paired with ethical considerations and safety assessments.
Finale
The conclusion of this article serves to encapsulate the myriad facets of nanotechnology and its practical applications. This review is essential not only for consolidating existing knowledge but also for establishing a foundation for future progress in this field. As we have explored, nanotechnology plays a pivotal role in diverse sectors such as medicine, electronics, materials science, and environmental science. Each area reveals unique benefits while simultaneously presenting challenges that necessitate careful consideration.
Summary of Findings
Throughout this exploration, several key points emerge prominently:
- Transformative Power: Nanotechnology is revolutionizing traditional practices, introducing innovations in drug delivery, diagnostic methods, and advanced materials.
- Interdisciplinary Nature: This field spans multiple disciplines, creating opportunities for cross-pollination between sectors like biotechnology and environmental science.
- Health and Safety Considerations: While the benefits are substantial, it is crucial to remain vigilant regarding potential health risks and environmental impacts. Regulatory frameworks may need to evolve alongside technological advancements.
- Economic Implications: The integration of nanotechnology into industries could significantly enhance efficiency and sustainability, potentially leading to economic growth.
The combination of these factors illustrates the urgent need to continue research and dialogue in nanotechnology.
Implications for Future Research
Looking ahead, future research in nanotechnology should focus on several critical areas:
- Health Risks and Toxicology: Investigating the long-term effects of nanomaterials on human health and the environment must remain a priority. Understanding how different nanoparticles interact with biological systems is essential for safe implementation.
- Sustainability and Eco-friendliness: Research should also emphasize the development of sustainable nanomaterials that minimize environmental impact, particularly in pollution remediation and energy solutions.
- Collaborative Efforts: Encouraging partnerships between academic institutions and industries may foster innovations that address real-world challenges. Collaboration can help bridge the gap between theoretical potential and practical utility.
- Legislative and Ethical Frameworks: As advancements occur, legal and ethical considerations should also evolve, ensuring that policies keep pace with technology while prioritizing safety and public welfare.
In summary, the exploration of nanotechnology is not just an academic pursuit; it encompasses vital societal implications that warrant thorough investigation and discussion. Only through sustained effort and interdisciplinary collaboration can the full potential of nanotechnology be realized.