Exploring Substitution Problems in Scientific Research
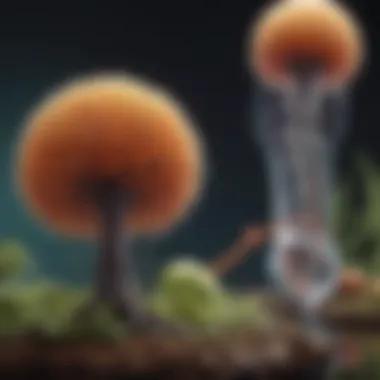
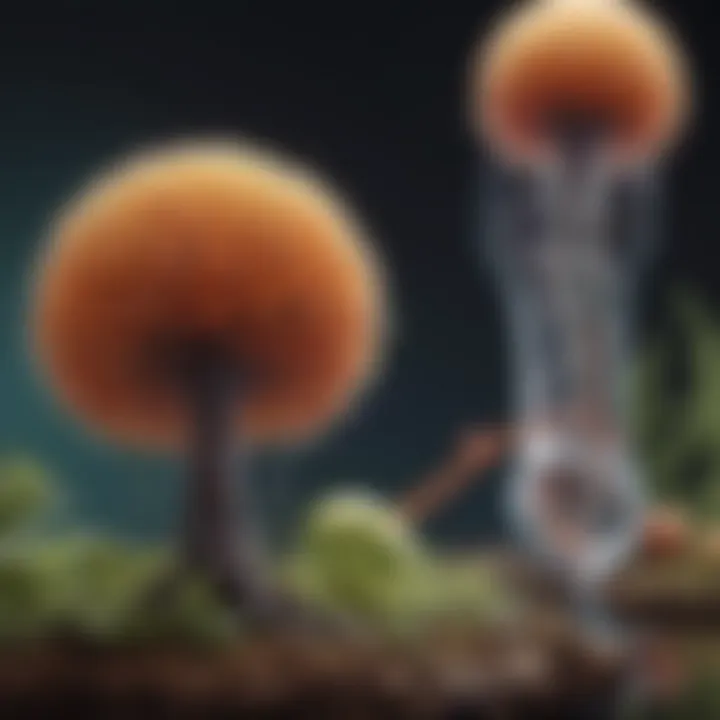
Intro
Substitution problems are prevalent across various scientific domains, manifesting in unique ways that challenge researchers and educators alike. Understanding these problems is crucial because they often influence experimental design, theoretical frameworks, and practical applications. This article will delve into substitution issues within three primary scientific fields: biology, chemistry, and physics. By investigating these challenges, scholars can better appreciate their significance and develop effective strategies for their resolution.
Research Overview
Summary of Key Findings
The exploration of substitution problems reveals several consistent themes. Firstly, the nature of substitution varies widely across disciplines; for instance, in biology, it may refer to genetic mutations, whereas in chemistry, it could involve the replacement of elements within compounds. Secondly, the consequences of ignoring these problems can lead to flawed results and misinterpretations. Notably, this article aims to illustrate not just the problems themselves but also potential solutions that have emerged through recent research.
Research Objectives and Hypotheses
This article strives to achieve the following objectives:
- Examine the types of substitution problems found in biological, chemical, and physical research.
- Analyze the implications these problems have on scientific outcomes.
- Propose methodologies and approaches that can effectively mitigate these challenges.
We hypothesize that a systematic understanding of substitution issues can enhance the accuracy and reliability of scientific experiments. A comprehensive overview will help students and professionals identify these problems early in their research endeavors.
Methodology
Study Design and Approach
To craft a thorough narrative, an analytical approach is taken, utilizing a qualitative study design. This involves a review of existing literature, case studies, and relevant scientific texts. Methods from each discipline will be compared, providing a multi-faceted view of substitution problems.
Data Collection Techniques
Various data collection techniques will be employed:
- Literature Review: Gathering scholarly articles and books to provide context.
- Case Studies: Analyzing specific instances of substitution problems to draw practical lessons.
- Expert Interviews: Consulting researchers in the fields of biology, chemistry, and physics for insights into current challenges and their solutions.
The insights derived from these methods will enrich the discussion on substitution problems, making it relevant for students and professionals working in scientific fields.
Defining Substitution Problems
In the context of scientific research, defining substitution problems is crucial. These challenges arise when one element needs to be replaced with another, and the implications of this substitution can ripple through various layers of complexity. Understanding these problems provides valuable insights into how scientists navigate research questions and hypotheses. It lays a foundation for effectively analyzing data and draws connections between theoretical frameworks and practical applications.
Overview of Substitution Problems
Substitution problems can manifest in different forms across various scientific fields. At its core, a substitution problem involves identifying an appropriate alternative that meets specific criteria, such as functionality or efficacy. In biology, this might involve replacing a gene to observe changes in phenotype. In chemistry, it often refers to replacing one atom in a compound with another to explore changes in reactivity. The primary objective is to analyze the consequences of the substitution in a controlled manner.
Key factors contributing to the complexity of these problems include:
- Disciplinary Variability: Different scientific areas have distinct norms and frameworks, leading to unique interpretations of substitution problems.
- Interconnected Systems: Substitution can affect numerous components within a system, making predicting outcomes challenging.
- Data Dependency: The availability and accuracy of data can significantly influence the success of addressing these problems.
By dissecting the idea of substitution, researchers can harness critical thinking to navigate challenges effectively. Thus, comprehensively understanding substitution problems is not merely academic; it serves practical functions in hypothesis testing, modeling, and experimentation.
Types of Substitution Problems
The types of substitution problems can be categorized based on their applications in various scientific fields. Understanding these types provides clarity for researchers and helps in developing targeted methodologies.
- Biological Substitution Problems:
- Chemical Substitution Problems:
- Physical Substitution Problems:
- Examining genetic variations where one allele is substituted for another, impacting traits.
- Exploring ecological substitutions, such as species replacement within a habitat contributing to ecosystem dynamics.
- Investigating methods for substituting reactants in a chemical reaction to optimize desired products.
- Understanding how substituents on a molecular structure can influence chemical behavior and properties.
- Substituting components within a physical system, such as materials in thermodynamic processes, to evaluate efficiency.
- In quantum mechanics, substitutive changes to variables can affect the outcomes of experiments.
Identifying and categorizing these substitution problems enhances research clarity while promoting interdisciplinary dialogue. Each type offers specific insights, ultimately contributing to a broader understanding of how substitution-effective strategies can be implemented across scientific disciplines.
Historical Context
Understanding the historical context surrounding substitution problems is crucial for grasping their evolution and significance across scientific disciplines. These problems have not only shaped research methodologies but have also influenced theoretical frameworks in various fields such as biology, chemistry, and physics. By examining the historical trajectory, researchers and students can gain insights into the complexities and nuances associated with substitution issues.
In the realm of scientific inquiry, historical context provides a backdrop against which contemporary challenges can be assessed. It allows for a critical evaluation of past methodologies and their outcomes, shedding light on lessons learned that can inform current practices. Furthermore, this context illustrates the progression of scientific thought, highlighting how foundational ideas have paved the way for advanced concepts and technologies today.
Evolution of the Concept
The concept of substitution problems has undergone significant transformation since its inception. Initially, these issues arose from practical needs within scientific research. Early scientists encountered various dilemmas when attempting to interchange or replace variables in their experiments. Over time, this led to the formalization of substation concepts through mathematical and statistical frameworks.
Researchers like Karl Pearson and Ronald A. Fisher contributed greatly to developing statistical methods that aid in addressing these problems. Their work established a solid foundation for future explorations in substitution issues, particularly in terms of quantifying relationships and predicting outcomes. Today, substitution is considered a vital element in hypothesis testing and data analysis, reflecting a more systematic understanding of how variables interact.
Key Historical Figures
Several key figures have made substantial contributions to the understanding of substitution problems, influencing how these issues are tackled across various scientific domains.
- Karl Pearson: Often regarded as a pioneer of statistics, Pearson introduced methods for correlation and regression analysis. His work laid the groundwork for many modern statistical techniques used in substitution problems.
- Ronald A. Fisher: As one of the foremost statisticians, Fisherβs research in experimental design and statistical inference significantly impacted how substitution issues are approached. His methodologies allowed for more sophisticated analyses of experimental data.
- J. Willard Gibbs: In chemistry and thermodynamics, Gibbs explored the concept of substitutions in chemical reactions, influencing how reaction mechanisms are understood. His work highlights the importance of chemical equilibrium in formulating substitution theories.
These figures, among others, shaped the dialogue surrounding substitution problems, steering research towards more rigorous and refined methodologies. Understanding their contributions provides a clearer picture of how the principles of substitution have evolved and how they continue to inform scientific inquiry.
Theoretical Framework
Understanding the theoretical framework surrounding substitution problems is crucial for leveraging insights and methodologies applicable across scientific research. This framework not only provides a structured approach to examining these problems but also outlines the foundational theories that inform practical applications. By establishing clear mathematical and statistical underpinnings, researchers can develop robust models that facilitate the analysis and interpretation of complex substitution scenarios.
Mathematical Models
Mathematical models serve as essential tools in addressing substitution problems. They allow for the abstraction of real-world phenomena into quantifiable terms, facilitating deeper analysis. These models can represent various scenarios wherein substitution occurs, ranging from the replacement of one variable with another to more complex systems involving multiple variables.
For example, in population genetics, mathematical models can express how alleles substitute over generations due to evolutionary pressures. Such models can be represented through equations that reflect genetic frequency changes, helping researchers to predict future allele distributions.
A common approach in constructing these models involves using differential equations to describe the rate of change in populations as substitutions occur. By analyzing these equations, researchers can extract significant insights into the dynamics of biological systems.
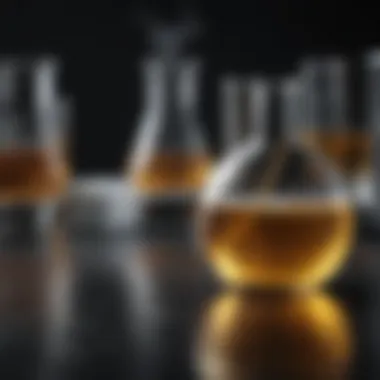
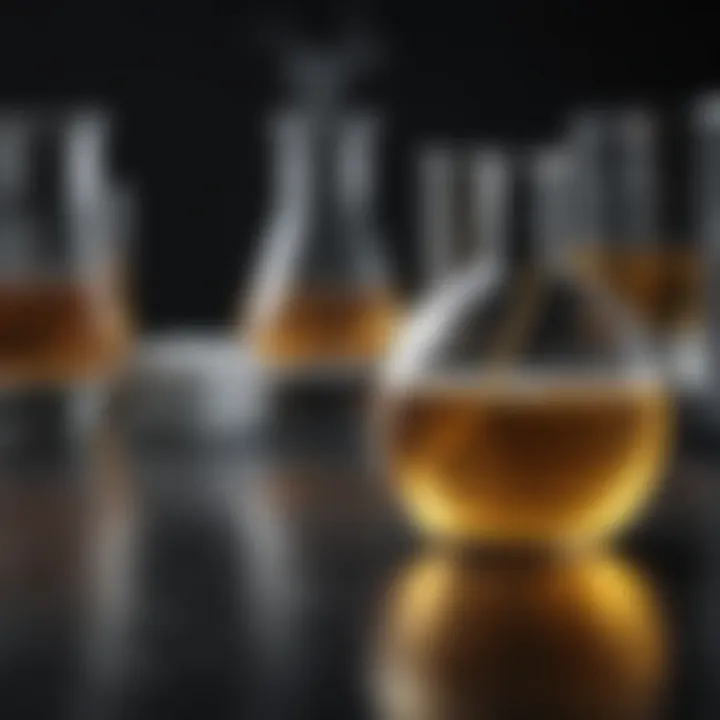
"Mathematical modeling is an indispensable component of investigating substitution challenges, providing clarity and predictions that may not be readily apparent from empirical observations."
Statistical Approaches
Statistical approaches are equally vital in managing substitution problems, offering techniques to assess and interpret data meaningfully. By applying statistical methods, researchers can evaluate the significance of substitutions and their implications within experimental or observational studies.
Among various statistical techniques, regression analysis is particularly prominent. It allows researchers to understand relationships between variables, which can be crucial when substitutions impact outcomes. For instance, in chemical research, regression models can help analyze how substituting one chemical compound for another affects reaction yields.
Moreover, hypothesis testing plays a significant role in enabling researchers to determine whether observed substitutions are statistically significant. This can help in confirming theories related to substitutions in fields like ecology, where researchers might ask if changes in species compositions significantly affect ecosystem functionality.
Applications in Biology
Substitution problems are integral to biological research, influencing our understanding of genetics, species interactions, and ecological systems. This section explores how substitution challenges affect biological inquiries, highlighting the need for precise analytical approaches in these scenarios. The significance of substitution in biology cannot be overstated as it leads to advancements not only in scientific theory but also in practical applications such as conservation efforts and medical research.
Genetic Variation and Substitution
Genetic variation serves as a cornerstone for evolutionary processes. Substitution events, which may involve the replacement of one nucleotide with another in a DNA sequence, are pivotal in determining an organism's traits as well as its adaptability to environmental changes. The study of such substitutions helps researchers identify advantageous or disadvantageous traits that may evolve through natural selection.
- Key Considerations:
- Mutation Rates: Understanding the frequency of mutations can help predict how quickly populations adapt to changing environments. The rate of substitution can significantly vary among species.
- Functional Impact: Not all substitutions are equal; some may lead to significant phenotypic changes, while others are silent and do not affect the organism.
- Applications in Medicine: Insight into genetic substitutions is critical in personalized medicine, where understanding an individual's genetic makeup allows for better treatment plans.
By investigating genetic substitution, scientists can draw connections between genetic change and broader biological phenomena, enriching our comprehension of life processes.
Ecosystem Dynamics
The dynamics of ecosystems are influenced greatly by substitution at both the genetic and species level. Substitution problems can arise when species interactions are modified, either through natural phenomena or human activities. These interactions frame the stability and functionality of ecosystems.
- Key Benefits of Understanding Substitution in Ecosystems:
- Biodiversity Assessment: Understanding which species can substitute for others in ecological roles can provide insights into biodiversity metrics and ecosystem resilience.
- Impact of Invasive Species: Studying the substitution of native species by invasives highlights how ecosystems can change when new players enter the scene.
- Habitat Conservation: Recognizing how species may substitute one another in changing environments can inform conservation strategies to protect vulnerable ecosystems.
The implications of studying substitution in ecosystems stretch far, providing strategic insights for biodiversity preservation and management practices.
Applications in Chemistry
The field of chemistry serves as a critical platform for understanding substitution problems. These problems embody various challenges and opportunities across chemical reactions and material science. The interactions between different elements, compounds, and conditions can lead to innovative solutions and the advancement of chemical processes.
Understanding substitution problems in chemistry allows researchers to systematically approach chemical reactions. This understanding facilitates the prediction of outcomes in various reactions, which is pivotal for both theoretical exploration and practical application.
Chemical Reactions and Substitutions
Chemical substitutions significantly influence the pathways of reactions. In a substitution reaction, an atom or group of atoms in a molecule is replaced by another atom or group. This process can alter the properties of the resulting compounds, making it valuable in synthesis.
- Types of Substitution Reactions
- Nucleophilic Substitution: A common mechanism where nucleophiles attack electrophiles, leading to the displacement of a leaving group.
- Electrophilic Substitution: Often seen in aromatic compounds, where an electrophile replaces a hydrogen atom.
- Radical Substitution: Involves radicals and can occur under conditions such as heat or light.
These substitutions can provide various outcomes based on the conditions and reagents used. Researchers must be meticulous in selecting conditions to achieve desired results.
"Understanding the specific types of substitution reactions allows chemists to tailor their approaches, leading to more efficient synthesis and innovation in chemical design."
Material Science Implications
In the context of material science, substitution problems are crucial in developing new materials with specific properties. Substitution can lead to changes in electronic, thermal, or mechanical properties that are of great interest in research and industry.
For instance, the substitution of different elements in alloys can enhance strength or durability. This has implications in constructing buildings, vehicles, and other structures that require resilience against harsh conditions.
Furthermore, in polymers, the substitution of monomers dictates the behavior of the final product, influencing factors like flexibility, strength, and thermal stability. Understanding how to manipulate these substitutions allows scientists to design materials with precise properties for various applications.
Notably, both how substitution reactions occur and their outcomes can be analyzed using computational methods, which enhances our understanding of material behaviors on a molecular level.
The applications of substitution problems in chemistry extend beyond fundamental chemistry alone, contributing to innovations in technology and materials. Through understanding these processes, chemists can drive advancements and tackle challenges in diverse areas.
Applications in Physics
Exploring substitution problems in physics reveals fundamental insights into the nature of the physical world. These problems not only present challenges but also offer opportunities for innovation and refinement in scientific methodologies. Understanding the role of substitution can enhance theoretical predictions, facilitate experimental design, and promote technological advancements. By examining specific applications in physics, we can gain a deeper appreciation of how these challenges affect fundamental research and its practical applications.
Substitution in Quantum Mechanics
Substitution in quantum mechanics often involves replacing variables or parameters in equations to solve problems related to particle behavior or energy states. One crucial aspect is the use of wave functions, which describe the probabilities of the presence of particles in various states. By altering conditions within the equations, physicists can predict outcomes tied to measurements. This substitution is vital in quantum computing as it helps model and simulate complex systems.
For instance, the substitution of position variables with momentum variables aids in understanding how particles interact on quantum scales. These substitutions support concepts like uncertainty principles and wave-particle duality, which are foundational to modern physics. Additionally, practical applications in quantum mechanics extend to fields like quantum cryptography, offering secure communication methods.
"Understanding substitutions in quantum mechanics is essential for advancing technologies like quantum computing and cryptography."
Thermodynamic Systems
In the realm of thermodynamics, substitution problems often relate to the transformation of one form of energy into another, or the exchange of heat and work within a system. These substitutes are explored through the laws of thermodynamics, which govern the relationships between different states of energy materials. One significant consideration is the use of enthalpy and entropy in calculations. By substituting values within these thermodynamic expressions, scientists can predict system behavior during changes of state, such as phase transitions.
The efficiency of energy systems, particularly in engines and refrigeration cycles, relies heavily on the principles of substitution. For example, substituting different working fluids can impact the performance of a heat engine or a refrigerator, making it crucial for engineers to understand these variables. Advances in material science are also linked with these principles, as new materials can introduce novel thermodynamic behaviors.
In summary, the study of substitution problems in both quantum mechanics and thermodynamic systems illustrates the importance of flexible thinking in physics. By reframing challenges through substitution, we can unlock new methods and technologies that drive research forward.
Challenges in Addressing Substitution Problems
Understanding the challenges in addressing substitution problems is crucial for effectively navigating this complex area of scientific research. Substitution problems can arise across various disciplines, and recognizing the difficulties can aid in developing strategies to overcome them. This section focuses on two primary challenges: data limitations and methodological constraints. Each of these elements plays a significant role in the advancement and resolution of substitution issues, making it imperative for researchers to consider these factors in their investigations.
Data Limitations
Data limitations present a significant barrier to effectively analyzing substitution problems. Research in many scientific fields often relies on accurate and comprehensive datasets. When data is sparse, outdated, or inaccurately collected, it can lead to misleading results and conclusions. In biology, for instance, genetic variation studies may suffer from inadequate sample sizes. This can hinder the ability to detect even subtle but important substitution patterns. Furthermore, errors in collected data can also lead to flawed statistical analyses, limiting the applicability of findings.
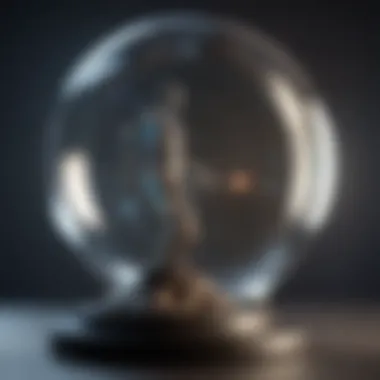
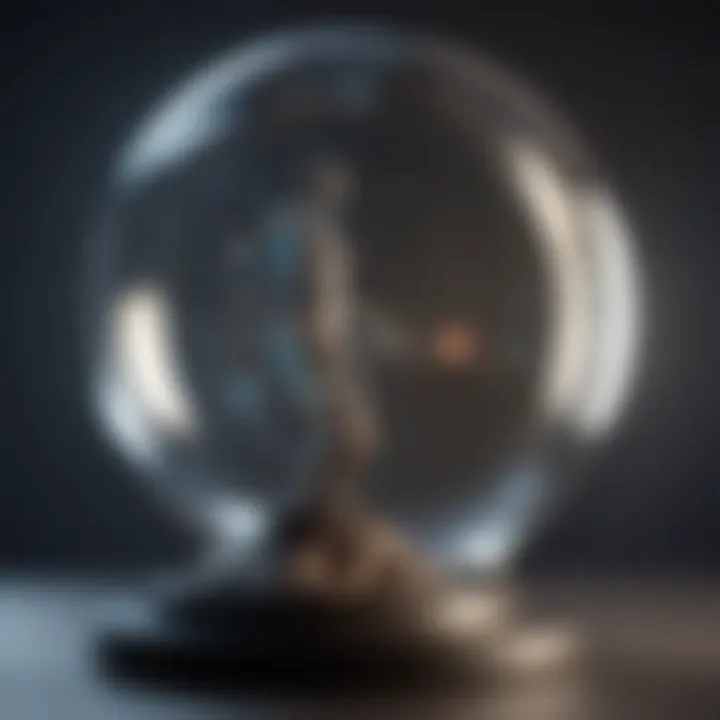
*
- Incomplete datasets
- Sample bias
- Misclassification of variables
These issues can result in gaps in knowledge that ultimately stifle innovation. Researchers must carefully evaluate the quality of the data they are using. They should proactively seek to refine their data collection methods and consider employing advanced statistical techniques to address potential shortcomings.
Methodological Constraints
Methodological constraints are another important challenge in substitution problem analysis. The approaches researchers choose to adopt can greatly influence the outcomes of their studies. For instance, traditional experimental designs may not adequately account for the complex interactions in biological ecosystems or chemical reactions. When methodological frameworks are rigid or ill-suited for the context of the study, important variables can be overlooked.
*
- Limited experimental designs
- Over-reliance on specific statistical methods
- Neglecting interdisciplinary insights
Researchers can benefit from adopting more flexible, interdisciplinary approaches to tackle substitution problems. By merging techniques and insights from different fields, scientists can create a more holistic understanding of the challenges they face. This encourages innovative problem-solving that is essential for progressing in this arena.
Effective problem-solving in substitution issues requires a comprehensive understanding of both data and methodologies. Addressing these challenges can lead to more robust research findings and greater collaboration across scientific disciplines.
Innovative Solutions
Innovative solutions play a crucial role in addressing substitution problems across various scientific domains. These solutions are essential not only for resolving existing challenges but also for paving the way for further advancements in research and application. As science continues to evolve, the ability to adapt and apply new methodologies becomes paramount. Innovative approaches can facilitate a better understanding of the intricate mechanisms behind substitution processes, improve accuracy, and ultimately lead to more effective applications in real-world situations.
By integrating innovative solutions, researchers can tackle substitution problems with fresh perspectives and cutting-edge techniques. Such solutions often lead to enhanced problem-solving abilities and increased collaboration among different disciplines. This interconnectivity fosters a sharing of ideas and methods that can inspire breakthroughs. As the complexity of scientific inquiries increases, innovative solutions can harness advanced technologies and methodologies, resulting in more adept handling of substitution issues.
Emerging Technologies
Emerging technologies are pivotal when exploring substitution problems. Advancements in computational power and data analytics have transformed traditional methodologies. For instance, the rise of big data allows researchers to analyze vast datasets that can reveal trends and patterns related to substitution in biological or chemical systems. Likewise, artificial intelligence and machine learning offer powerful tools for modeling substitution scenarios, predicting outcomes, and optimizing experimental conditions.
These technologies bring several benefits, such as:
- Increased Efficiency: They streamline processes, enabling faster analysis and interpretation of complex data.
- Higher Accuracy: Advanced modeling can reduce errors in predictions regarding substitution behavior across different scenarios.
- Greater Accessibility: Tools like cloud computing make sophisticated technologies available to a broader audience, democratizing research capabilities.
Emerging technologies can also facilitate interdisciplinary collaborations, as they provide common grounds for researchers with diverse expertise to unite towards solving substitution problems. With the collective knowledge base, they build robust frameworks that support innovative research initiatives.
Interdisciplinary Approaches
Interdisciplinary approaches are foundational for addressing substitution problems effectively. When different fields collaborate, they bring unique insights, techniques, and terminologies that can enhance understanding and foster innovation. For example, integrating principles from biology, physics, and chemistry can yield novel solutions to problems that may not be solvable from a single disciplinary perspective.
There are various aspects to consider when implementing interdisciplinary approaches:
- Communication: Clear dialogue between disciplines ensures that all parties understand the contexts of the substitution issues. This reduces misunderstandings and promotes accurate knowledge transfer.
- Complementary Expertise: Combining expertise from various fields can lead to more comprehensive models that better represent complex substitution scenarios.
- Holistic Viewpoint: Considering multiple scientific traditions can unveil fresh insights into substitution problems, leading to breakthroughs in understanding or application.
The Role of Computational Methods
In current scientific research, computational methods play a pivotal role in addressing substitution problems. These methods facilitate the handling of complex data sets and computational models, thus enhancing analytical capabilities in various disciplines. Utilizing computer-assisted tools enables researchers to visualize and simulate scenarios that would otherwise remain theoretical.
The evolution of computational methods has transformed research approaches, offering more precise predictions and efficient problem-solving strategies. With these advancements, scientists can conduct more exhaustive analyses, ultimately leading to better decision-making in experimental contexts.
Modeling and Simulation
Modeling and simulation are essential facets of computational methods. Through modeling, researchers can create mathematical representations of real-world systems to study their behavior. This allows them to hypothesize potential outcomes based on specific variables. For scientific problems that involve substitutions, models can facilitate understanding the relationships between different components.
Simulations, often run on computer systems, allow researchers to test hypotheses by mimicking real-life processes. They can examine how changes in one element influence the entire system. For example, in biology, researchers can simulate genetic variations to see how substitutions might affect phenotypic traits. This is particularly useful, as real-world experiments can be costly and time-consuming.
Benefits of modeling and simulation include:
- Enhanced understanding of complex systems.
- Ability to predict outcomes under varying scenarios.
- Reduced time and costs compared to traditional experimentation.
- Increased opportunities for collaboration across disciplines.
These tools are not without their challenges. Model accuracy depends on the assumptions made and the data quality. Consequently, researchers must remain vigilant, ensuring only the most reliable data is utilized in their simulations.
Machine Learning Applications
Machine learning is increasingly becoming integral to computational methods in addressing substitution problems. This approach uses algorithms to analyze large data sets and uncover patterns that may not be immediately apparent. By training these algorithms on established data, researchers can predict trends and identify potential substitutions based on historical information.
The utility of machine learning is evident in various applications, including:
- Drug discovery: Identifying potential candidate compounds that could substitute certain elements in drug formulations.
- Predictive maintenance: Recognizing when to replace components in physical systems before failure occurs.
- Environmental monitoring: Detecting shifts in ecosystems due to substitution of certain species.
Machine learning applications are powerful, yet they require careful implementation. Ensuring that training data is representative and that models are validated is crucial for accuracy. Furthermore, the interpretability of machine learning outcomes must be prioritized to ensure that findings are meaningful and actionable.
In summary, computational methods significantly enhance the ability to tackle substitution problems within scientific research. Modeling and simulation provide structured frameworks for hypothesis testing, while machine learning contributes predictive analytics that drives innovation and improvement across various domains.
Case Studies
Case studies serve as a crucial tool in understanding substitution problems across various scientific disciplines. They provide empirical evidence and context for theoretical concepts. Analyzing real-world scenarios highlights the complexities and nuances involved in these problems. Additionally, case studies can demonstrate the applicability of methods used to address these challenges. This empirical approach aids in validating theoretical frameworks and methods previously discussed in the article. Furthermore, they foster a better comprehension of how variations in substitution influence outcomes in practical settings.
Biological Systems Analysis
In the realm of biology, case studies focusing on biological systems are paramount. They showcase how substitution problems affect genetic variations and organism adaptation. By studying specific cases, researchers can observe the impact of genetic substitution on evolutionary processes. For instance, examining the Darwin's finches gives insights into how slight changes in genetic makeup can affect survival and reproductive success.
These case studies help in understanding the dynamics of ecosystem responses to environmental changes. Real-life examples illustrate the ways organisms adapt through mutation and natural selection, emphasizing the importance of substitution at the molecular level. Furthermore, documenting these cases can lead to significant advancements in conservation strategies.
Chemical Substitution in Industry
Chemical substitution in industrial applications presents another critical area for case studies. These studies illustrate the importance of substituting hazardous chemicals with safer alternatives. For example, the development of bio-based solvents offers a practical application of substitution principles.
In industries such as manufacturing and pharmaceuticals, the effects of substitution affect not only safety but also process efficiency and environmental footprints. Case studies documenting successful substitutions help validate approaches to sustainability in the chemical industry. Moreover, they provide a model for best practices, highlighting challenges overcome and innovations achieved. By analyzing such examples, professionals gain insights that inform future strategies and policies in chemical safety.
Future Directions
The landscape of substitution problems in scientific research is dynamic and continuously evolving. As scientific disciplines progress, the approaches to addressing substitution challenges also need to adapt. This section examines how future directions can shape research methodologies and educational paradigms.
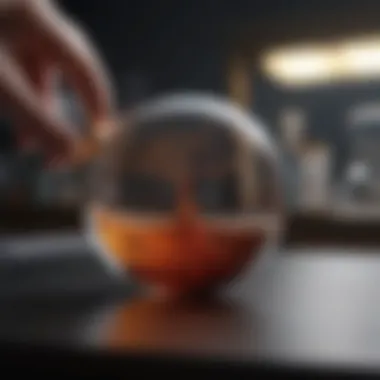
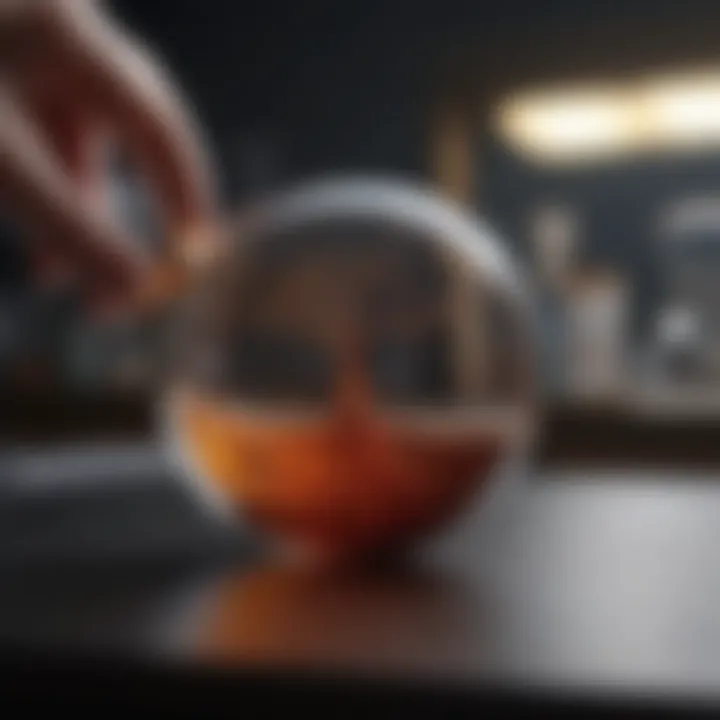
Emerging Trends in Research
Emerging trends indicate a shift towards more integrated scientific approaches when tackling substitution problems. Interdisciplinary strategies incorporating insights from various fields can lead to innovative solutions.
- Collaborative Research: Scientists are increasingly working across fields, leveraging expertise from biology, chemistry, and physics to address complex substitution issues. This collaborative spirit nurtures creativity and generates more holistic solutions.
- Advanced Computational Techniques: The rise of computational power enables researchers to model substitution dynamics with higher precision. Simulations and virtual experiments can facilitate deeper understanding before real-world applications take place.
- Focus on Sustainability: With growing environmental concerns, there is a swing towards sustainable practices. Researchers are exploring substitution methods that minimize ecological impacts, especially in chemical substitutions.
βEmerging trends in research will reshape the way we handle substitution problems, leaning towards sustainability and collaboration.β
Predicted Advances
Predictions for the future point towards several advances that could fundamentally change the approach towards substitution problems:
- Blockchain in Research Management: Utilizing blockchain technology can enhance transparency and efficiency in collaborative research efforts, particularly for data sharing between different research teams.
- Artificial Intelligence Integration: The use of AI can predict substitution outcomes based on massive datasets. This predictive capability can streamline research processes and refine results significantly.
- Personalized Approaches in Biology: As research develops, there may be a greater focus on personalized medicine. Specific substitution problems may cater to individual genetic profiles, revolutionizing treatment plans in healthcare.
Anticipating these advances is crucial for researchers. Engaging with forthcoming technologies and methodologies will better prepare scientists for the challenges ahead. Understanding these future directions lays the groundwork for effective adaptations in research and educational practices.
Implications for Education
Understanding substitution problems is essential in the realm of education, particularly within scientific disciplines. Integrating these concepts into academic curricula allows students to gain practical knowledge and critical thinking skills that are valuable in research and industry settings. This section discusses the importance of incorporating substitution problems in educational frameworks, alongside the benefits and considerations that arise from this approach.
Integrating Substitution Problems into Curricula
Incorporating substitution problems into science curricula provides a robust foundation for students. It encourages them to engage with complex concepts that they may encounter in future studies and research. By learning about how substitution problems function across various disciplines, students can:
- Develop analytical skills necessary for tackling real-world issues.
- Gain insight into how these problems are resolved in fields like chemistry, biology, and physics.
- Understand the interdisciplinary nature of scientific research, promoting collaboration among different fields.
The integration can take several forms. Educators might use case studies, practical experiments, or simulations to illustrate substitution mechanisms. For example, discussing genetic variation in biology can lead understandings of ecological adaptations. In chemistry, exploring chemical reactions that involve substitutions offers students hands-on opportunities to experiment and analyze results directly.
Preparing Students for Future Research
Preparing students for future research is another critical element of integrating substitution problems into education. Familiarity with these concepts allows students to navigate the complexities they may face in professional settings. Additionally, a sound understanding of substitution problems helps students:
- Cultivate problem-solving abilities crucial for innovative research.
- Encourage a mindset that embraces challenges, fostering resilience in their academic journey.
- Enable them to contribute meaningfully to discussions and projects centered around scientific inquiry.
Furthermore, equipping students with these skills goes beyond theoretical knowledge. It prepares them for direct involvement in research opportunities, where they can apply what they've learned in real-world contexts. This can lead to advancements in various scientific fields, ensuring that the next generation of researchers is well-prepared to tackle emerging challenges.
"Education is the most powerful weapon which you can use to change the world." β Nelson Mandela
In summary, the implications of substitution problems in education are profound. By integrating these topics into curricula and preparing students for future research, we ensure that they are well-equipped to address the challenges of tomorrow's scientific landscape.
Collaboration Opportunities
In the realm of scientific research, collaboration is not merely advantageous; it is essential. The complexity of substitution problems requires diverse expertise and perspectives. Engaging in collaborative efforts enables researchers to share knowledge, resources, and methodologies. This comprehensive approach can lead to innovative solutions that an individual researcher may not achieve alone.
Collaborations can take numerous forms, including interdisciplinary partnerships that unite various scientific disciplines. This multidimensional approach not only fosters creativity but also enhances the rigor of investigations. By drawing upon the distinct strengths of different fields, teams can address substitution problems from various angles. For example, a biology researcher might collaborate with a chemist to better understand the chemical processes involved in genetic variation, thereby creating a more integrated perspective on the challenges at hand.
Benefits of Collaboration:
- Resource Sharing: Researchers can access each otherβs databases, tools, and laboratory facilities, which accelerates the pace of discovery.
- Diverse Perspectives: Collaborative teams bring together different viewpoints and expertise, enriching the analytical process.
- Increased Funding Opportunities: Joint projects can appeal to funding bodies that prioritize collaborative initiatives.
- Enhanced Learning Opportunities: Individuals in these teams often learn from one another's skills or knowledge bases, fostering personal and professional development.
Collaboration must be approached thoughtfully. Factors such as communication dynamics, project management, and conflict resolution can significantly influence the success of collaborative efforts. Establishing clear objectives and protocols at the start of any partnership is crucial for ensuring all team members remain aligned throughout their endeavors.
Interdisciplinary Collaborations
Interdisciplinary collaborations represent a unique opportunity to tackle substitution problems by blending insights and techniques from various scientific domains. These partnerships can yield results that reflect a deeper understanding of complex issues. Each discipline offers distinct methodologies, theoretical frameworks, and analytic techniques, creating a rich tapestry from which new ideas can emerge.
For instance, when biologists and computational scientists work together, they can use statistical models to analyze genetic substitution patterns more effectively. The biologist's expertise in cellular mechanisms complements the computational scientist's knowledge in data modeling. This synthesis allows for more precise predictions regarding evolutionary outcomes in different environments.
Considerations for Interdisciplinary Collaborations:
- Shared Vocabulary: Establishing a common language is necessary to facilitate communication among team members from different fields.
- Common Goals: Clearly defined research objectives help prevent misunderstandings and keep the group focused.
- Flexibility: Members must be adaptable and open to new ideas and methods, which can enhance the collaboration.
By fostering these collaborations, researchers can address the multifaceted aspects of substitution problems more successfully and create impactful solutions that contribute to the scientific community.
Research Partnerships
Research partnerships, especially those formed between academia and industry, can significantly amplify the impact of scientific investigation. These partnerships can offer a practical context for theoretical insights, particularly concerning substitution problems. This interplay between theory and practice is essential for translating research findings into tangible applications.
For example, a partnership between a university and a chemical company could involve exploring new materials that utilize substitution methods within manufacturing processes. Collaborating in this manner not only generates innovative solutions but also allows researchers access to industry-standard tools and methodologies.
Strategies for Effective Research Partnerships:
- Identify Complementary Strengths: Seek partners whose expertise aligns with your research needs.
- Establish Mutual Benefits: Ensure that all parties gain from the partnership to maintain motivation and engagement.
- Regular Communication: Frequent updates and discussions can help navigate challenges and maintain momentum.
Through well-structured research partnerships, scientists can significantly enhance their ability to address substitution problems across various disciplines, ultimately benefiting both academic knowledge and industry practice.
The End
The conclusion serves as a crucial synthesis of the preceding discussions regarding substitution problems in scientific research. It encapsulates the key themes explored throughout the article and emphasizes their significance across various disciplines like biology, chemistry, and physics. The value of understanding these problems lies not only in their theoretical explanations but also in their practical applications.
Addressing substitution problems allows researchers to gain insights that influence future studies and innovations. Consider for instance the role of substitution in genetic variation, where understanding molecular replacements can lead to breakthroughs in genetic engineering. Similarly, in material science, the implications of chemical substitutions inform the creation of new materials with desired properties.
Moreover, the importance of interdisciplinary collaboration cannot be overstated. This collaboration enhances the ability to approach substitution challenges from multiple perspectives, enabling more comprehensive solutions.
In summary, the conclusion reaffirms that an analytical approach to substitution problems fosters deeper understanding, encourages innovative thinking, and contributes to advancing scientific knowledge.
Summary of Key Points
- Understanding Substitution Problems: Unraveling these issues is vital for progress in research.
- Interdisciplinary Applications: Insights from biology, chemistry, and physics reveal interconnectedness among scientific fields.
- Future Research Directions: Emphasis on the need for continued investigation into substitution problems will drive future innovations.
Final Thoughts
In the realm of scientific inquiry, substitution problems represent both challenges and opportunities. They are essential in drawing connections between complex theories and real-world applications. The informed analysis of these issues can lead to advancements that reshape our understanding of the world.
As we progress, it is important to maintain an open-minded approach. Future research can benefit from embracing diverse methodologies and perspectives. Engaging in thoughtful discussions and sharing findings enriches the scientific community as a whole.
The analysis throughout this article demonstrates that embracing substitution problems is fundamental not just for academic pursuits but also for practical advancements. This insight paves the way for future explorations that can alter scientific domains in transformative ways.