Spectrophotometric Analysis: Principles and Applications
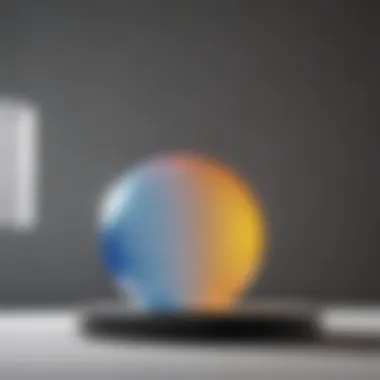
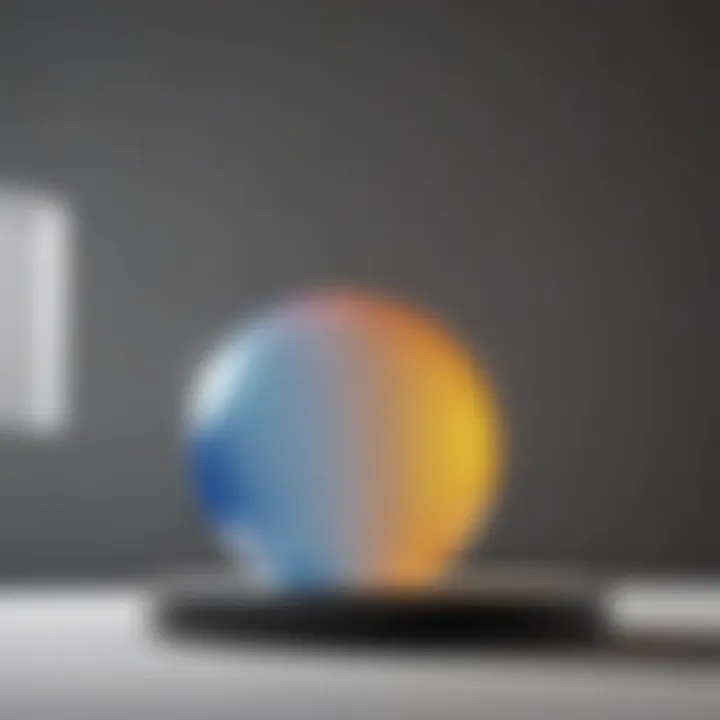
Intro
Spectrophotometric analysis is a cornerstone in the realm of scientific measurement. It involves the assessment of how light interacts with substances to provide great insights into their properties. This technique has diverse applications across chemistry, biology, and environmental science. Understanding these principles not only enhances experimental design but also improves the accuracy of analytical results.
The essence of spectrophotometry lies in its ability to quantify the concentration of solutes based on their light absorption characteristics. Each substance has a unique way of absorbing light, creating a distinct spectral signature. This feature allows researchers and professionals to differentiate between compounds, analyze mixtures, and conduct quality control more effectively.
Research Overview
Summary of Key Findings
Recent studies underscore the advancements in spectrophotometric techniques. There is a move toward integrating high-performance instruments with better sensitivity and throughput. Additionally, the emergence of new software applications facilitates more sophisticated data analysis. The combination of these factors vastly improves the reliability of spectrophotometric results.
- Enhanced detector technology has improved sensitivity.
- Automated systems enable higher throughput for large sample batches.
- Innovations in data analysis have increased the accuracy of spectral interpretation.
Research Objectives and Hypotheses
This article aims to elucidate the fundamental principles of spectrophotometric analysis. The objective is to explain the working mechanisms of various spectrophotometers and to highlight their relevance in different fields. Key hypotheses include:
- The hypothesis that newer spectrophotometric technologies outperform traditional methods in terms of speed and accuracy.
- The proposition that increased automation leads to a reduction in human error across analytical processes.
Methodology
Study Design and Approach
This research encompasses a systematic review of existing literature on spectrophotometric techniques. Primary sources were utilized, emphasizing notable advances in technology and their implications for various fields. Case studies from applications in pharmaceutical analysis and environmental testing provide concrete examples.
Data Collection Techniques
Qualitative and quantitative data were gathered from multiple peer-reviewed sources, ensuring comprehensive coverage of the topic. Each study is evaluated for its methodology and findings, thus providing a balanced view of current trends and future directions in spectrophotometric analysis.
"Spectrophotometric analysis is not just a measurement tool; it is an integral part of modern scientific inquiry, providing essential data across disciplines."
Preamble to Spectrophotometric Analysis
Spectrophotometric analysis has become a fundamental tool across various scientific fields. Its importance lies primarily in its ability to measure the absorbance and transmission of light through samples. This technique provides quantitative and qualitative data, crucial for researchers and industries alike. In a world where precision in data is paramount, spectrophotometric methods offer reliable results that can inform decisions in numerous domains, such as chemistry, biology, and environmental science.
Definition and Scope
Spectrophotometry can be defined simply as the measurement of light intensity as a function of wavelength. This process involves the interaction between light and matter, where specific wavelengths are absorbed or transmitted by a sample. The scope of spectrophotometric analysis extends to countless applications, including determining the concentration of substances in various solutions and investigating the physical properties of materials. By understanding how light interacts with different substances, scientists can derive significant insights that contribute to advancements in research and applied science.
Historical Development
The development of spectrophotometric techniques dates back to the early experiments in optics. One significant milestone was in the 19th century when scientists began using prisms and diffraction gratings to analyze light spectra. The establishment of the Beer-Lambert Law further solidified the foundation of this discipline, providing a quantitative relationship between absorbance and concentration. Over the years, various innovations have occurred, from the construction of more sophisticated spectrophotometers to the integration of digital technology. These historical advancements have shaped current practices, and an understanding of these developments is essential for any researcher or student delving into spectrophotometry.
Fundamental Principles of Spectrophotometry
Spectrophotometry is a fundamental technique that relies on the comprehension of lightβs interaction with matter. Understanding these principles is crucial for accurate analysis in various scientific fields. The science of light absorption and transmission lays the groundwork for both qualitative and quantitative analyses. Without a solid foundation in these principles, the subsequent applications become less effective and void of clarity.
Understanding Light and Electromagnetic Spectrum
Light consists of electromagnetic radiation, which spans a wide spectrum of wavelengths. This spectrum includes visible light, ultraviolet (UV), and infrared (IR). Each segment has distinct properties and behaviors when interacting with substances. In spectrophotometry, it is essential to focus on specific wavelengths that molecules absorb. This targeted absorption highlights the unique characteristics of different materials, providing insights into their composition. By analyzing the response of these materials to various wavelengths, researchers can infer both qualitative and quantitative aspects of their samples. Notably, different molecules absorb light at unique wavelengths, creating a barcode-like pattern that allows for specific identification.
Beer-Lambert Law
The Beer-Lambert Law serves as a pivotal foundation for spectrophotometry, articulating the relationship between light absorbance and concentration of a substance. This law is critical in determining how much light a substance absorbs based on its concentration and the path length of the light.
Beer-Lambert Equation
The Beer-Lambert Equation is mathematically represented as: Where:
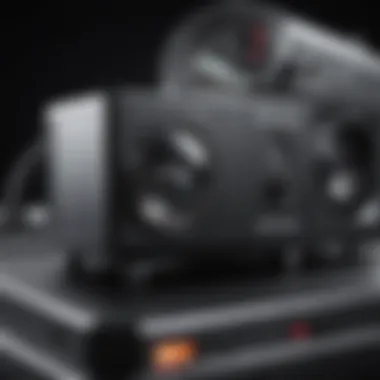
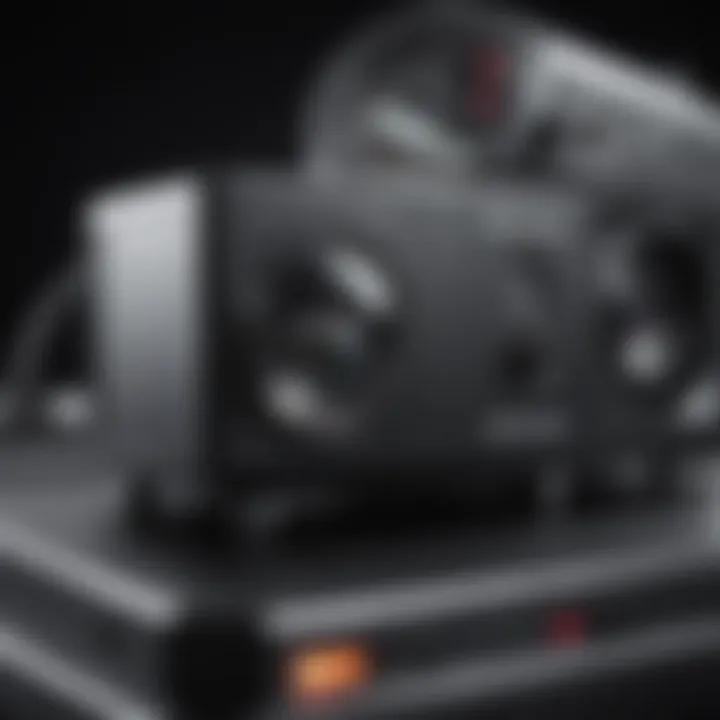
- A = absorbance
- Ξ΅ = molar absorptivity
- c = concentration of the substance
- l = path length of light through the sample
This equation is widely recognized for its simplicity and ease of application in laboratory settings. It applies to various analytical scenarios, making it a beneficial choice in this article. The key characteristic is its linearity; absorbance is directly proportional to concentration, allowing for straightforward calculations. However, it is crucial to consider the limitations that come with this model, as it does not hold under all conditions.
Limitations of the Law
Despite its strengths, the Beer-Lambert Law has its limitations, which can impact analysis accuracy. One key characteristic is its application range; the law remains effective up to a specific concentration. Beyond this range, deviations occur due to various factors like scattering or overlapping spectral bands. These limitations are significant in high-concentration scenarios, where accurate measurements may fail. Therefore, understanding these drawbacks is essential for effective implementation in spectrophotometric analysis.
Types of Absorption Spectra
Absorption spectra can be categorized into three main types: continuous, line, and band spectra. Continuous spectra arise from a broad range of wavelengths being absorbed by a substance, resulting in a smooth curve. Line spectra consist of discrete lines representing specific wavelengths absorbed by individual elements, while band spectra occur in larger molecules, where groups of wavelengths are absorbed. Each of these spectra provides critical information for identifying the composition of a sample, enabling robust analytical results across various domains.
"The precision of spectrophotometric measurements essential for various applications heavily relies on understanding its foundational principles."
Understanding and applying these principles not only enhances the accuracy of results but also develops a deeper comprehension of material interactions with light, paving the way for advanced applications across numerous scientific fields.
Components of a Spectrophotometer
Understanding the components of a spectrophotometer is crucial to appreciate its function and applications in various scientific fields. Each element plays a significant role in the overall efficiency and accuracy of spectrophotometric analysis. A robust understanding of these components will enrich the readerβs knowledge and can raise their competency in practical analysis.
Light Source
The light source is fundamental to a spectrophotometer. It illuminates the sample with a beam of light, enabling the measurement of absorbance and transmittance. Common light sources include tungsten lamps for visible light and deuterium lamps for ultraviolet light.
The choice of light source affects the wavelength range and intensity of the light reaching the sample. A stable and uniform light source contributes to consistent and reproducible results. Variability in light intensity can lead to inaccurate data, making careful selection essential for precise analysis.
Monochromator
A monochromator serves as a filtering device that isolates specific wavelengths of light. Its purpose is to select a narrow range of wavelengths to pass onto the sample. This process is imperative because different substances absorb light at distinct wavelengths.
Common types of monochromators include prism monochromators and diffraction gratings. Each type offers unique advantages depending on the application. The ability to fine-tune the selected wavelength enhances the sensitivity and selectivity of the analysis.
Sample Holder
The sample holder is where the specimen is positioned for analysis. It must securely hold the sample while allowing the light to pass through with minimal interference. Factors such as path length and material of the holder can influence the outcomes of measurements.
Sample holders vary based on the nature of the sample being analyzedβbe it a liquid in a cuvette or a solid sample placed within appropriate containers. Proper alignment is critical, as any misalignment can introduce errors in the measurement process.
Detector
The detector converts the transmitted light into a measurable signal. Common types of detectors include photodiodes, photomultiplier tubes, and charge-coupled devices (CCDs). Each detector has its own operating principles and is chosen based on requirements such as sensitivity, speed, and wavelength range.
The detector's ability to translate light into an electrical signal often dictates the limits of detection. Hence, selecting an optimal detector designed for the specific application is important for achieving accurate results.
Data Processing Unit
This component acts as the heart of the spectrophotometric instrument. It processes the signals received from the detector and converts them into numerical data that can be analyzed. Modern data processing units often feature integrated software that allows for real-time analysis and data representation.
Several activities such as calibration, data storage, and analysis of the results occur in this unit. This makes the data processing unit central to ensuring that the output is reliable and meaningful for any scientific inquiry.
By understanding the components of a spectrophotometer, users can better appreciate how adjustments in one part can affect the overall performance and results of the analysis. In such a precise field, even minor changes can have significant impacts on the conclusions drawn from spectrophotometric measurements.
Methodology of Spectrophotometric Analysis
Understanding the methodology of spectrophotometric analysis is crucial for anyone engaged in this scientific discipline. The methodology not only outlines the steps involved in carrying out the analysis but also emphasizes the care and precision required to achieve reliable results. The approach is systematic and can be broadly categorized into several key aspects: preparation of samples, calibration of instruments, conducting the analysis, and interpreting data. Each of these elements plays a significant role in enhancing the accuracy and reliability of the measurements acquired.
Preparation of Samples
Sample preparation is the first step in spectrophotometric analysis. This stage is critical as it directly impacts the validity of the results. Samples must be treated, diluted, or otherwise manipulated to ensure that they fall within the optimal range for analysis. The purity of the sample is essential. Contaminants can skew results, making it difficult to obtain accurate concentrations or absorbance values. It is common to use solvents such as distilled water or specific reagents to prepare samples, depending on the analysis required. Careful control over variables such as temperature and pH is also important, as these factors can influence the behavior of the sample under light exposure.
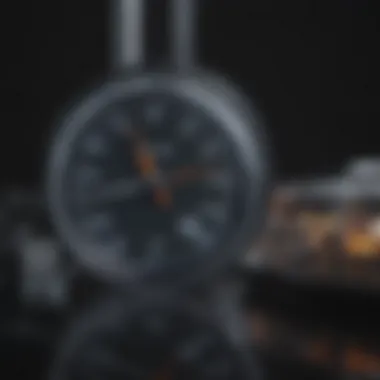
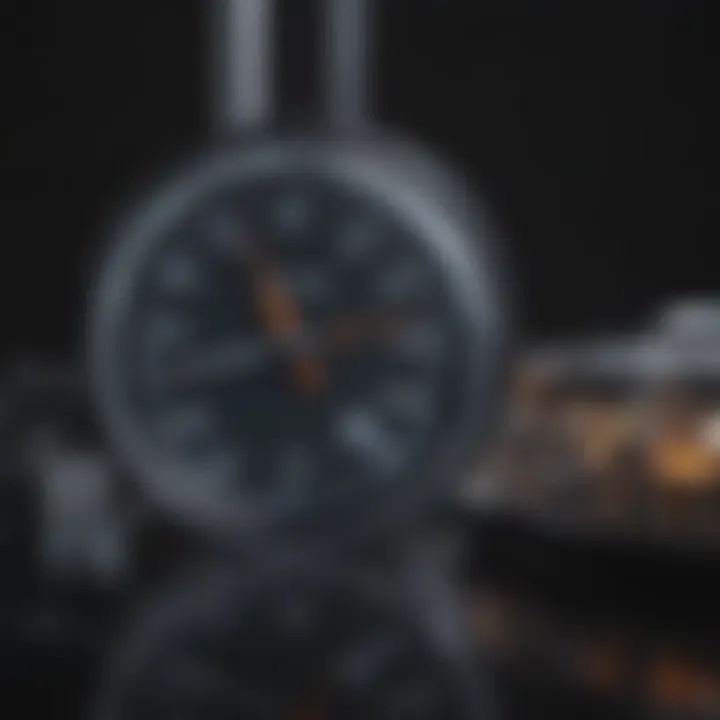
Calibration of Instruments
Calibration of instruments is the next important step in the methodology. The spectrophotometer must be properly calibrated to ensure accurate measurements. This involves setting a baseline using a blank solution, which typically contains all components of the sample except the analyte of interest. Applying the correct calibration methods allows for the identification of systematic errors and enhances measurement reliability. Regular calibration against standardized solutions is also recommended to maintain the precision of the instrument. This step is fundamental, as errors in calibration can lead to significant inaccuracies in all subsequent analyses.
Conducting the Analysis
Conducting the analysis itself follows a structured approach. Once the samples are prepared and the instruments calibrated, the actual measurement begins. The spectrophotometer sends a light beam through the sample to determine how much light is absorbed at specific wavelengths. It is vital to choose the right wavelength since different compounds absorb light differently. Running multiple trials can help to ensure accuracy and reproducibility. Throughout this process, proper technique must be employed to minimize external interferences that could distort the results.
Data Interpretation
Interpreting the data is perhaps one of the most critical aspects of spectrophotometric analysis. The data obtained from the instrument need to be analyzed accurately to derive meaningful conclusions.
Understanding Absorbance and Transmittance
Absorbance and transmittance are two fundamental concepts in spectrophotometric analysis. Absorbance refers to the amount of light absorbed by the analyte, while transmittance is the amount of light that passes through the sample. Understanding these terms is essential because they are used in the Beer-Lambert Law for calculating concentrations. The key characteristic of absorbance is its direct proportionality to concentration, making it a beneficial choice for quantifying chemical substances. However, one limitation includes the potential for non-linearity at high concentrations, which can complicate the analysis.
"Absorbance is crucial for determining the concentration of unknown samples using known standards."
Graphical Representation
Graphical representation of data plays an important role in visualizing the results of spectrophotometric analyses. Typically, a plot of absorbance versus concentration is generated. This visual interpretation can elucidate relationships that may not be obvious from numeric data alone. Graphical representations facilitate better understanding and clearer communication of the results. However, it can be less effective if data points do not form a clear relationship or show anomalies, necessitating caution in interpretation. The graphical analysis also allows for easier identification of trends or patterns in large datasets, enhancing the overall discourse in research and application.
Applications of Spectrophotometric Analysis
The applications of spectrophotometric analysis are extensive, providing vital insights in various scientific fields. This technique allows the study of light interactions with matter, enabling researchers to quantify and characterize substances within complex mixtures. The accuracy and versatility of spectrophotometry make it an essential tool in laboratories worldwide. This section explores its crucial applications in chemistry, biology, and environmental science, emphasizing key aspects, benefits, and considerations.
In Chemistry
Quantitative Analysis
Quantitative analysis in chemistry utilizes spectrophotometry to determine the concentration of substances in a solution. This application stands out due to its ability to deliver precise measurements effectively. By applying the Beer-Lambert Law, it allows chemists to derive concentrations using absorbance data. A significant feature is its sensitivity, which makes it a beneficial choice for detecting low levels of analytes. The primary advantage is fast results with minimal sample preparation. However, it can be less effective when the sample has high turbidity or color interference, limiting its effectiveness.
Qualitative Analysis
Qualitative analysis employs spectrophotometric techniques to identify substances based on their absorption spectra. This application is fundamental in pharmaceutical development and material science, as it assists in confirming the presence of specific compounds. Its key characteristic is the unique absorption fingerprint exhibited by different molecules. This feature facilitates easy identification during analysis. While qualitative analysis is beneficial due to its straightforward approach, it may not always provide conclusive evidence of compound identity without corroborating techniques.
In Biology
Enzyme Activity Measurement
Spectrophotometry plays an essential role in measuring enzyme activities, which is a critical aspect of biotechnology and biochemistry. It helps quantify the rate of enzyme-catalyzed reactions by monitoring changes in absorbance over time. The methodβs key characteristic is its ability to provide real-time data, which is crucial for understanding enzyme kinetics. It is highly beneficial for research, as it can be applied in various experiments without extensive modifications. Nevertheless, variations in enzyme concentration or substrate can affect accuracy, thus care must be taken when designing experiments.
Protein Concentration Determination
Determining protein concentration is pivotal in many biological experiments. Spectrophotometric methods, such as the Bradford or Biuret assays, are often used. The notable aspect of this application is its simplicity and speed. Researchers can analyze multiple samples quickly, allowing high-throughput studies. The main advantage lies in its effectiveness in quantifying protein despite the presence of contaminants. However, these methods can encounter challenges if the sample contains interfering substances, leading to skewed results.
In Environmental Science
Pollutant Detection
Spectrophotometry is a vital tool for pollutant detection, providing clear data on the presence of contaminants in various matrices such as air and water. It allows for rapid screening, which is essential in environmental monitoring and regulatory compliance. The key characteristic here is the ability to quantify pollution levels accurately. This advantage aids policymakers in making informed decisions about environmental health. However, challenges include the need for calibration against specific pollutants and the potential for false positives from other substances.
Water Quality Assessment
Utilizing spectrophotometry in water quality assessment helps determine the health of aquatic ecosystems. Parameters such as turbidity, chemical oxygen demand, and nutrient concentrations are often measured. The method's strength is its non-destructive nature, enabling sampling without altering the water's composition. This application is critical for ensuring safe drinking water and monitoring environmental changes. However, it requires thorough understanding of the equipment used, as improper calibration can lead to misleading results.
"Spectrophotometry provides a robust framework for analyzing compounds across diverse scientific disciplines, ensuring accuracy and efficiency in various applications."
Among the diverse applications outlined, spectrophotometric analysis stands as a cornerstone of modern scientific practices, enabling continuous advancement in research and development across disciplines.
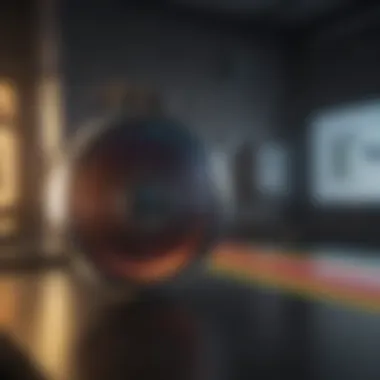
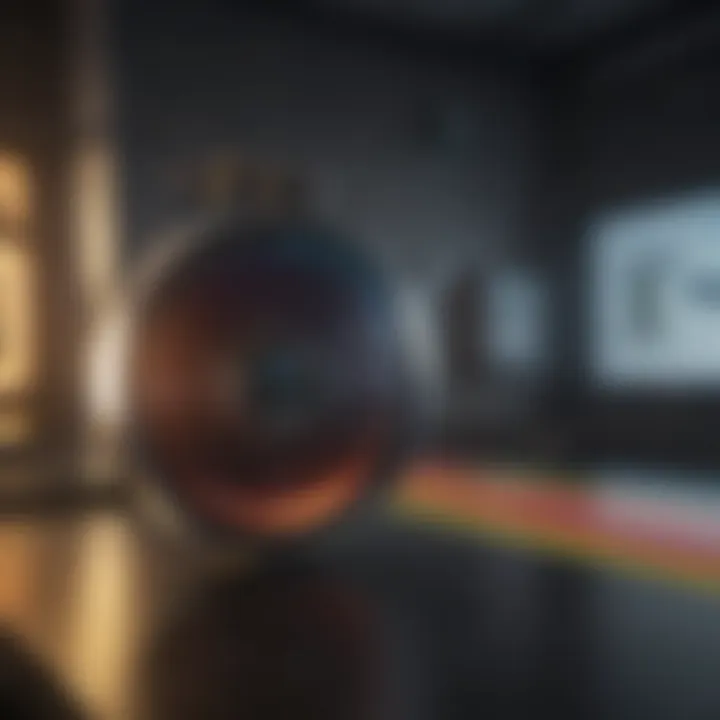
Technological Advancements in Spectrophotometry
Technological advancements in spectrophotometry are essential for the evolution of analytical techniques. Innovations in this field improve the accuracy, reliability, and efficiency of spectrophotometric analysis. These developments cater to the growing demands of researchers and industries while addressing the intrinsic challenges faced in traditional methods. The importance of focusing on such advancements stems not only from their impact on research quality but also their implications for practical applications across various scientific disciplines.
Miniaturization of Spectrophotometers
Miniaturization refers to the trend of reducing the size of spectrophotometric instruments while maintaining performance and accuracy. This has led to portable devices that are ideal for in-field analysis, enabling quicker results without the need for bulky laboratory setups. Smaller devices can easily be applied in remote locations, which is valuable for environmental monitoring and on-site testing.
Benefits of miniaturization include:
- Increased Accessibility: It allows more researchers, including those in under-resourced areas, to access advanced analytical techniques.
- Cost-Effectiveness: Miniaturized instruments are often less expensive to manufacture and maintain.
- User-Friendly Designs: Smaller devices typically have simpler operations, broadening their usability among non-specialists.
Integration with Software and Automation
The integration of software with spectrophotometric instruments has revolutionized the way data is collected and analyzed. Advanced software can process vast amounts of data nearly instantaneously, reducing human error and increasing the throughput of results. Additionally, automation facilitates repetitive tasks like calibration and sample positioning, significantly saving time.
This integration brings several benefits, including:
- Real-Time Data Analysis: Users can receive immediate feedback on samples, allowing for quicker decision-making.
- Enhanced Data Visualization: Sophisticated software tools provide graphical representations and analytical models for better interpretation of results.
- Data Management: Improved data handling capabilities support better storage, retrieval, and sharing of analytical outcomes.
Emerging Technologies in Spectral Analysis
Emerging technologies in spectral analysis include novel techniques and applications that further enhance the capabilities of spectrophotometric analysis. Examples like hyperspectral imaging systems are gaining traction. These systems can capture and analyze hundreds of bands of light across a wide spectrum simultaneously.
Some notable advancements include:
- Quantum Dots: These semiconductor particles have unique optical properties and improve sensitivity in detection.
- Surface-Enhanced Raman Scattering (SERS): This technology enhances the Raman scattering signals and allows for identification of low-concentration substances.
- Artificial Intelligence (AI): With AI in spectral analysis, patterns in data can be identified more effectively, facilitating predictive analytics.
The convergence of innovative technologies is shaping the future of spectrophotometric analysis, pushing the boundaries of what is currently achievable in scientific research.
Future Trends in Spectrophotometric Analysis
The field of spectrophotometric analysis is evolving. Innovation in technology continuously shapes how we approach research and diagnostics. This section explores the emerging trends that could influence the future of spectrophotometry. These trends play a critical role in increasing efficiency, precision, and accessibility in various scientific domains.
Integration with Emerging Scientific Fields
Integration of spectrophotometric techniques with fields such as biochemistry and materials science is becoming vital. For example, in biochemistry, advancements in spectrophotometric analysis enhance our understanding of complex biochemical pathways. Techniques like surface plasmon resonance are closely related to spectrophotometry and increase the sensitivity of detection methods.
Researchers use these combined tools to analyze biomolecular interactions. By merging spectrophotometric methods with genomic and proteomic analyses, scientists can develop higher precision in quantifying changes at the molecular level. This integration also provides new insights into drug development and personalized medicine, bolstering therapeutic strategies.
Environmental and Sustainability Considerations
Environmental concerns are increasingly shaping the landscape of spectrophotometric research. Developing procedures that minimize waste and optimize resource usage is imperative. Spectrophotometric methods are now being designed to reduce chemical usage, supporting more sustainable practices in both research and industry.
A prominent area is the assessment of pollutants in water. Advanced spectrophotometric techniques help detect trace amounts of contaminants, offering rapid and reliable data crucial for environmental monitoring. This aligns with the global aim of fostering sustainable practices, ensuring the protection of ecosystems.
Moreover, applying spectrophotometric analysis in renewable energy research is gaining traction. This includes studying photovoltaic materials and optimizing their efficiency. Integrating sustainability goals with spectrophotometric innovations opens new pathways for researchers.
"The advancement of spectrophotometry holds unprecedented potential to bridge gaps between various scientific disciplines and address pressing global challenges."
In summary, the future of spectrophotometric analysis is promising. Integrating with emerging fields and emphasizing sustainability can enhance both research capabilities and environmental stewardship. These developments will not only improve analytical precision but also ensure that scientific progress aligns with ecological considerations.
Epilogue
In wrapping up the discourse on spectrophotometric analysis, it is crucial to emphasize the multifaceted benefits and implications of this technique. Spectrophotometry stands as a fundamental analytical tool across diverse scientific domains, facilitating both qualitative and quantitative measurements. Its ability to provide accurate and reproducible results underscores its significance in research and industry. This article has detailed various principles, methodologies, and applications, making it a valuable resource for those involved in scientific inquiry.
Recapitulation of Key Points
The exploration began with a definition and scope, establishing spectrophotometric analysis as pivotal for understanding light interactions with matter. Core principles like the Beer-Lambert Law elucidate how absorbance relates to concentration, a vital point for accurate measurements. Key components of spectrophotometers were outlined, illustrating how each part contributes to overall functionality. The methodology section clarified the processes necessary for effective analysis, emphasizing sample preparation and data interpretation. In addition, real-world applications in chemistry, biology, and environmental science highlighted the versatility of this technique, illustrating its relevance in practical scenarios. Moreover, advancements in technology point towards more efficient and capable instruments that can integrate seamlessly into modern scientific workflows.
Implications for Future Research
Spectrophotometric analysis undoubtedly paves the way for further exploration in many fields. Future research can focus on enhancing the sensitivity of spectrophotometers, making it possible to detect lower concentrations of analytes. Research might also delve into miniaturization and the integration of artificial intelligence in spectrophotometric methods, potentially leading to advanced data analysis capabilities. Furthermore, the exploration of sustainable practices within spectrophotometry invites consideration of environmentally-friendly materials and methods.
As the landscape of science continues to evolve, so too will the techniques utilized to navigate it. The ongoing commitment to refining spectrophotometric methods and exploring novel applications can yield new insights and foster innovation, indicating a promising future in both academic and industrial settings.