Comprehensive Overview of Redox Flow Batteries
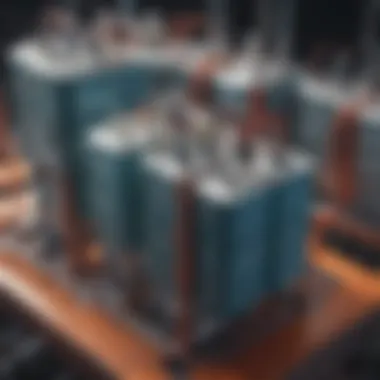
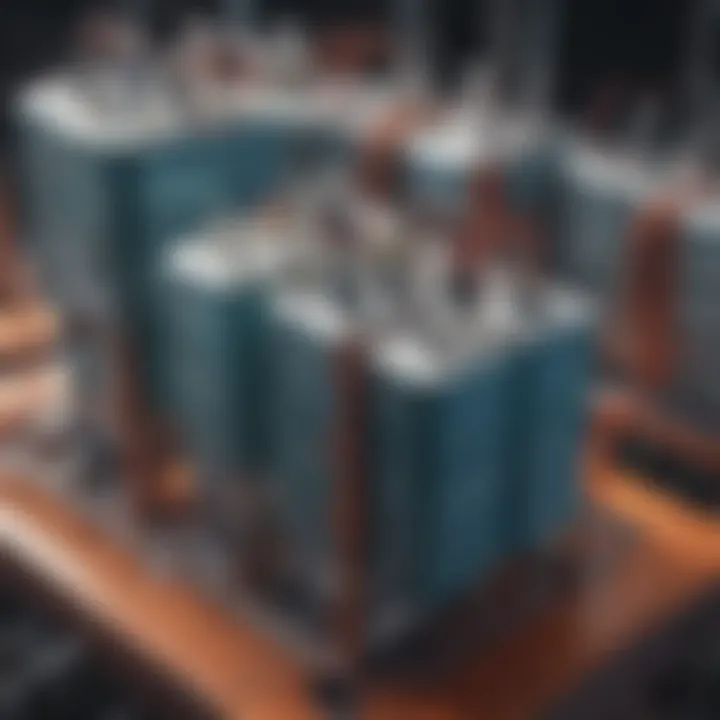
Intro
Redox flow batteries (RFBs) are attracting significant attention in the field of energy storage. These systems offer unique operational advantages compared to traditional battery technologies. As the world shifts towards renewable energy sources, understanding RFBs becomes crucial. Their ability to store energy at scale and provide long-duration discharge capabilities is vital for stabilizing intermittent energy supplies.
This section provides a foundation for further discussion about the principles, components, and applications of redox flow batteries. By breaking down the complexities of these systems, we can appreciate their role in the future of energy storage.
In the sections that follow, we will delve into the fundamental mechanics of RFBs, evaluate their pros and cons, and assess their potential in various applications such as renewable energy integration and electric vehicles. This exploration will shed light on how RFBs can contribute to advancing energy solutions globally.
Prelims to Redox Flow Batteries
Redox flow batteries represent an innovative approach to energy storage, addressing some critical needs of our modern power demands. Their ability to store and release energy from various sources makes them particularly significant in today's energy landscape. Their relevance lies in the increasing reliance on renewable energy systems, which require efficient and reliable storage solutions.
In understanding redox flow batteries, one must appreciate how they differ from traditional energy storage technologies. They utilize liquid electrolytes, allowing for scalable energy storage capacities. This adaptability can address the challenges posed by fluctuating energy supply from sources like solar and wind.
Additionally, the longevity and cycle stability of redox flow batteries provide further advantages; they can endure thousands of charge-discharge cycles with minimal degradation. As industries seek more sustainable energy solutions, grasping the specifics of redox flow batteries and their potential applications is essential.
Definition and Importance
A redox flow battery is an electrochemical system that stores energy in chemical form and releases it through redox reactions. These batteries consist of two electrolyte solutions, which flow through a cell stack. During operation, this cycle allows for energy conversion between chemical and electrical energy. The fundamental appeal of redox flow batteries lies in their scalability; one can adjust the system size to meet energy needs without changing its internal chemistry.
The significance of redox flow batteries extends beyond mere energy storage. They foster increased integration of renewable energy sources into the grid. By allowing for peak shaving and load leveling, these systems can help manage energy supply more effectively.
Historical Context
The concept of redox flow batteries is not new. The chemistry behind them has its roots dating back to the late 19th century, but practical applications did not emerge until the late 20th century. Early developments were limited due to cost and material limitations. However, advances in materials science and engineering have catalyzed renewed interest in recent decades.
Research efforts from the 1970s onwards focused on improving the efficiency and viability of these systems. Notably, the emergence of the vanadium redox flow battery in the 1980s galvanized interest due to its minimal cross-contamination and stable performance. Following this breakthrough, various designs and chemistries have emerged, with each iteration refining the technology's efficiency and practicality.
Ongoing research continues to push the boundaries of redox flow batteries, making them a compelling option for sustainable energy solutions. Their evolution from niche technology to mainstream consideration signifies their potential impact on future energy systems.
Fundamental Principles of Redox Flow Batteries
Understanding the fundamental principles of redox flow batteries is essential for grasping their role within the broader context of energy storage technologies. These principles describe the electrochemical processes that enable redox flow batteries to charge, store, and discharge energy efficiently. Without a grasp of these basic concepts, it is challenging to appreciate the advantages and limitations that characterize this technology.
Basic Electrochemistry
Redox flow batteries operate based on the principles of reduction and oxidation, colloquially called redox reactions. At the core of their operation are two distinct electrolyte solutions, which contain active compounds that undergo oxidation and reduction reactions during charge and discharge cycles. This setup allows energy to be stored in the chemical bonds of the active materials, leading to energy availability upon demand.
During the charging process, electrical energy drives the oxidation of the active species in one half-cell and the reduction of the other active species in the second half-cell. When the battery is discharged, the reverse occurs, producing electrical energy. This process highlights the significance of the half-cell reactions, and it sets the redox flow battery apart from traditional battery systems.
The overall chemical equations can be quite complex but understanding the basic abstractions is sufficient. Additionally, one can adjust the capacity and power output of the battery system by varying the concentrations of the electrolytes, which can lead to more tailored solutions for specific applications.
Energy Storage Mechanism
The energy storage mechanism in redox flow batteries is inherently linked to their unique design. In conventional batteries, energy is stored within solid electrodes. However, in a redox flow battery, energy is stored in liquid electrolytes that flow through the electrochemical cells. This design lends itself to significant advantages.
- Scalability: By increasing the size of the electrolyte storage tanks, one can easily scale the energy capacity. This means that redox flow batteries can be tailored to meet various energy demands without the constraints of fixed-size components found in traditional batteries.
- Independent Power and Energy Storage: The configuration of redox flow batteries allows the power output to be decoupled from energy storage. Power ratings depend on the surface area of the electrodes and stack design, whereas energy capacity is determined by the volume of electrolytes. This flexibility is rare in energy storage solutions.
- Long-lasting Capacity: Since the active materials in the liquid state can be easily replenished or replaced, the longevity of redox flow batteries is notable. They can undergo thousands of charge-discharge cycles without significant degradation.
In summary, the fundamental principles of redox flow batteries reveal the ingenuity of this technology. Understanding the basic electrochemistry and energy storage mechanisms offers valuable insights into their advantages over conventional energy storage solutions.
Redox flow batteries represent a unique approach to energy storage, maximizing efficiency while minimizing environmental impact.
These insights not only illuminate the workings of redox flow batteries but also underscore their potential in addressing modern energy challenges.
Components of Redox Flow Batteries
The prosperity of redox flow batteries depends heavily on their components. Each element plays a vital role in determining the performance, efficiency, and longevity of the energy storage system. Understanding these components is essential for anyone interested in the intricacies of redox flow technology.
Electrolytes
Electrolytes serve as the medium for charge transport in redox flow batteries. They facilitate the movement of ions between the two half-cells during the charging and discharging processes. The choice of electrolyte directly impacts the energy efficiency and operational stability of the battery. Common electrolytes include vanadium ions, iron-chromium systems, and organic compounds.
The chemical stability of these electrolytes must align with the operating conditions, as degradation can lead to reduced performance. Moreover, the solubility of charging species is crucial in maximizing energy density. For instance, vanadium electrolytes offer advantages like reduced cross-contamination between half-cells, which can enhance the lifespan of the system. However, their cost and availability are also factors that need consideration.
Electrodes
Electrodes are another critical component in redox flow batteries. They are the sites where the redox reactions occur. The materials selected for the electrodes can drastically affect the total charge and discharge capacities. Common electrode materials include carbon-based compounds, graphite, and metallic substrates.
A major consideration is the electrocatalytic activity of the material. This property influences how effectively the electrode facilitates the redox reactions. Furthermore, the structural integrity of electrodes during repeated cycling is vital. If electrodes degrade quickly, it jeopardizes the overall efficiency and lifespan of the battery.
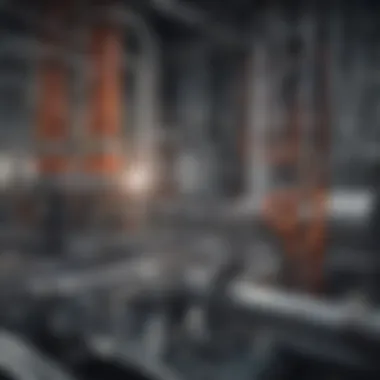
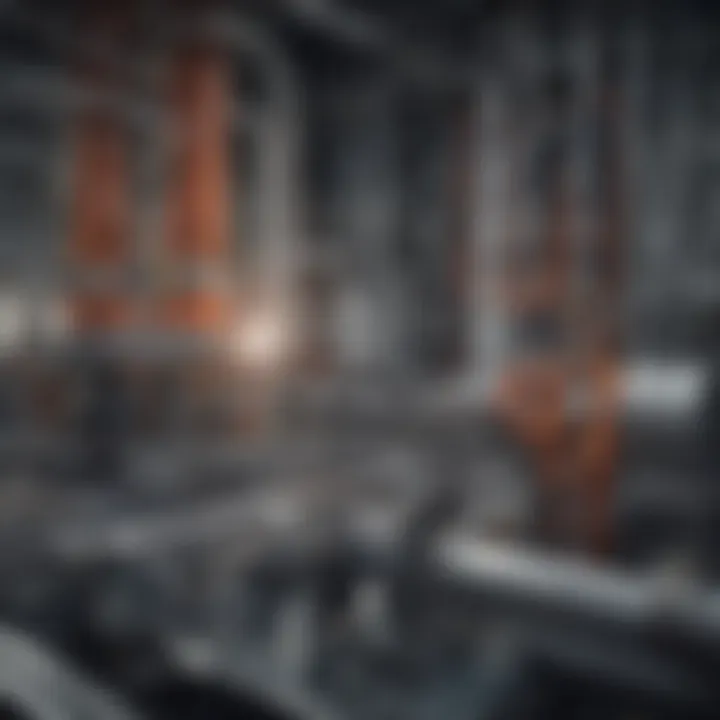
Some modern batteries are now utilizing composite structures to improve performance. By combining conductivity with a high surface area, these materials can enhance charge transfer rates. This development is promising for future advancements in electrode technology for redox flow batteries.
Membranes
Membranes in redox flow batteries act as selective barriers, allowing only specific ions to pass through while separating the two electrolyte solutions. They are essential for maintaining the electrochemical stability of the system. The choice of membrane affects not only the energy efficiency but also the overall cost and complexity of the battery system.
Common materials for membranes include Nafion and other polymeric materials. These membranes must possess good ionic conductivity, chemical stability, and mechanical strength. The efficiency of ion transport through the membrane can determine the overall energy loss during operation. Some researchers are exploring the use of new materials, such as anion-exchange membranes, to improve performance.
"The membrane's function is not just a separator; it is critical for optimizing the flow battery's efficiency and effectiveness in energy storage."
Low resistance in the membrane can translate into improved battery efficiency, making it a focal point for ongoing research. Enhancing membrane technology remains a key challenge but offers significant potential for the future of redox flow batteries.
Types of Redox Flow Batteries
Understanding the different types of redox flow batteries is crucial for comprehending their unique characteristics and the roles they play in energy storage solutions. Each type features specific chemistries, operational efficiencies, and applications, establishing its significance in the realm of renewable energy. By discerning these variations, stakeholders in energy production, distribution, and consumption can make informed decisions regarding their deployment.
Vanadium Redox Flow Battery
The vanadium redox flow battery (VRFB) stands out as one of the most developed types of redox flow batteries. Its core principle relies on the use of vanadium ions in both the positive and negative electrolytes. This reduces the risk of cross-contamination between positive and negative sides of the cell. The abilities of vanadium to exist in different oxidation states is key to its effectiveness.
One notable advantage of VRFBs is their long cycle life, which can exceed 20 years, depending on the operational conditions. They also exhibit a relatively high energy density compared to other flow battery types. Additionally, the scalability of VRFB systems makes them suitable for a variety of applications, from small-scale installations to large-scale energy storage for grid applications.
However, the cost of vanadium is a consideration that cannot be overlooked. Market fluctuations can significantly affect pricing, which impacts the initial investment for setting up these systems. Despite this, the long-term benefits of VRFBs may outweigh the upfront costs, especially in settings where durability and longevity are prioritized.
Iron-Chromium Flow Battery
Iron-chromium flow batteries combine iron and chromium for their electrochemical processes, benefiting from the abundant availability of these elements. This type of battery has been of interest for its relatively low cost and great potential for large-scale applications. The basic operation involves iron ions oxidizing at the anode and chromium ions at the cathode.
Iron-chromium systems can provide reasonable energy density, making them competitive in the market. One of the distinct advantages is their resilience in harsh operating conditions, which can be useful in diverse environments. These features make iron-chromium flow batteries attractive for applications that require robust systems with a lower capital investment.
However, performance can sometimes be compromised by issues such as voltage losses and lower round-trip efficiency compared to vanadium systems. Therefore, understanding the specific demands of a project is essential to determine if an iron-chromium flow battery will meet those needs effectively.
All-Vanadium and Hybrid Systems
All-vanadium and hybrid redox flow battery systems are designed to leverage the strengths of vanadium while incorporating other materials to enhance performance. All-vanadium setups use vanadium for both electrolytes, ensuring long-term stability and minimized cross-contamination.
Hybrid systems may involve a combination of two different redox processes, which could increase energy density while retaining some benefits of flow technology. Both configurations are still in discussion for various applications, especially those aligned with renewables like solar or wind, where energy storage is becoming increasingly critical.
The potential for innovative materials to enhance efficiency is promising. Advancements could lead to significant improvements in both capacity and discharge rates.
Thus, the ongoing exploration of these systems signifies a critical juncture in redox flow battery development, emphasizing the need for research and innovation in materials and designs.
"The exploration of new materials in all-vanadium and hybrid systems is essential for advancing redox flow battery technology and maximizing its real-world applications."
Operational Mechanisms
The operational mechanisms of redox flow batteries are central to their function and performance. Understanding these mechanisms not only clarifies how energy is stored and released but also highlights the efficiency and flexibility of this technology. This section will delve into the specifics of charging and discharging processes, underscoring their significance in the larger framework of energy storage solutions.
Charging Process
The charging process of redox flow batteries is a key phase where electrical energy is converted to chemical energy. During this phase, ions are transferred through the electrolyte, altering their oxidation states. When an external power source is connected to the battery, it pushes electrons to one electrode while pulling them from another, creating a potential difference. As a result, the electrolyte solution undergoes a redox reaction. This reaction facilitates ion engagement with the electrodes, which transforms electrical energy into stored chemical energy within the liquid electrolytes.
Understanding this process is critical for evaluating the efficiency of redox flow batteries. Various factors influence the charging efficiency, including the concentration of the electrolytes, the surface area of the electrodes, and the technology used in the membrane separation. This phase is not simply about energy input; a systematic approach can enhance overall energy retention and quicken recharge times.
Discharging Process
In the discharging process, the stored chemical energy is converted back into electrical energy for use. When the load is connected to the battery terminals, the redox reactions reverse. The ions move from one half-cell to another through the membrane, releasing electrons in the process. These electrons flow through the external circuit, providing electric power.
The efficiency of discharging significantly impacts the overall performance of a redox flow battery. Several parameters affect this efficiency, such as the flow rate of the electrolytes, the quality of the electrodes, and the design of the system. Proper optimization during this phase can lead to better performance, extended battery life, and lower operational costs.
The coordination between charging and discharging mechanisms in redox flow batteries dictates their usability and adaptability in various energy systems.
By understanding the operational mechanisms, including what happens during the charging and discharging processes, one can appreciate the advantages redox flow batteries present in energy management systems. This knowledge is essential for advancing research toward more effective and sustainable energy storage solutions.
Advantages of Redox Flow Batteries
The advantages of redox flow batteries (RFBs) are significant and impact both current and future energy storage technologies. Unlike conventional batteries, RFBs offer unique benefits that enhance their application in various fields. For students and professionals alike, understanding these advantages is essential in assessing RFBs' place in renewable energy solutions.
Scalability
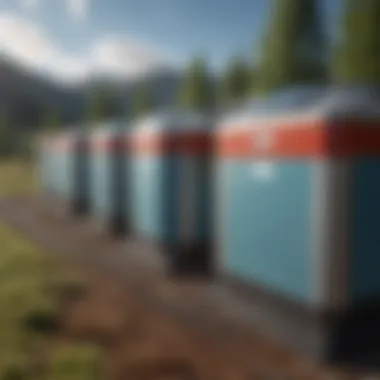
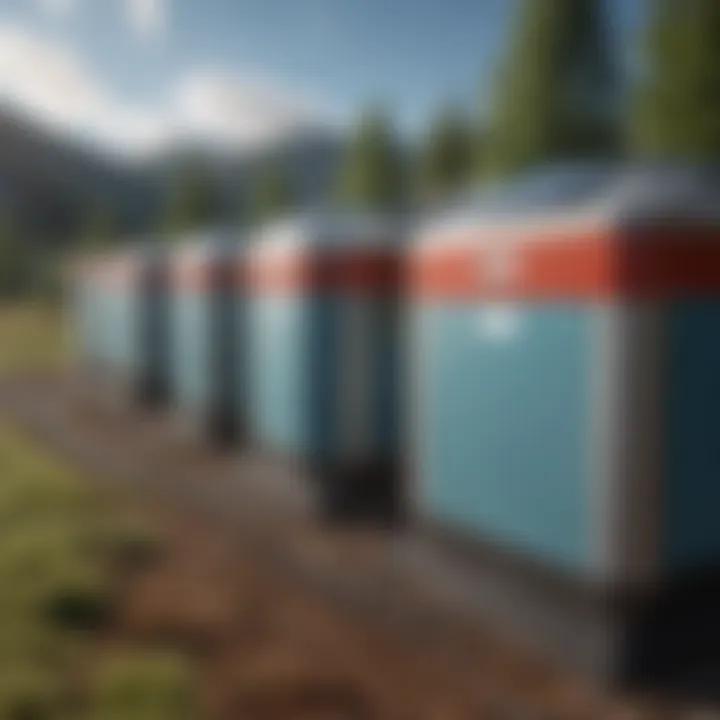
Scalability is one of the most notable features of redox flow batteries. The system design allows them to be scaled up easily, accommodating larger energy storage needs without changing the core technology.
- Modular Design:
Redox flow batteries have a modular design. Each module can be added as needed, helping to meet increasing demands effectively. - Storage Capacity:
The energy storage capacity can be expanded by increasing the sizes of the tanks that hold the electrolytes. Thus, capacity can be adjusted according to requirements.
This flexibility makes RFBs particularly attractive for grid energy storage applications where load demands can fluctuate. As renewable energy sources like solar and wind become increasingly prevalent, scalable solutions prove paramount in managing energy surges and deficits.
Long Cycle Life
Another advantage of redox flow batteries is their long cycle life. Unlike traditional batteries that degrade with repeated charge-discharge cycles, RFBs do not suffer from similar wear and tear.
- Reduced Degradation:
The electrochemical reactions in RFBs occur in liquid electrolytes. This separation reduces the risk of electrode degradation. - Extended Lifespan:
Moreover, RFBs can operate for tens of thousands of cycles. This durability equals a longer lifespan compared to lithium-ion or lead-acid batteries.
Long cycle life contributes to lower replacement costs and better overall efficiency, making RFBs a more sustainable choice for energy storage.
Safety and Stability
Safety and stability are critical concerns in energy storage systems. RFBs demonstrate exceptional performance in these areas, which adds to their appeal.
- Non-flammable Electrolytes:
Many redox flow batteries utilize non-flammable electrolytes, reducing the risk of fires or explosions. This property ensures safer operation under various conditions. - Thermal Stability:
RFBs typically feature better thermal management than traditional batteries, allowing them to operate in a wider range of environments. This stability is critical for large-scale applications.
The robust safety profile of redox flow batteries makes them suitable for both industrial and residential uses, where safety cannot be compromised.
In summary, redox flow batteries provide notable advantages that include scalability, long cycle life, and enhanced safety. These features make RFBs a compelling option for various applications in energy storage.
Limitations and Challenges
Understanding the limitations and challenges associated with redox flow batteries (RFBs) is crucial. While RFBs offer significant advantages, they are not without drawbacks. Assessing these issues can help researchers and industry professionals develop better solutions and optimize performance. This section dives into three key areas: cost considerations, energy density limitations, and material availability and environmental impact.
Cost Considerations
The initial cost of redox flow batteries can be a hurdle to their widespread adoption. Compared to lithium-ion batteries, RFBs typically require a higher capital investment. This can deter companies looking to implement these systems for energy storage. Factors contributing to high costs include the manufacturing of specialized components like membranes and the need for large tanks to store electrolytes.
Producing vanadium, a primary component in many RFB systems, incurs additional costs. Price fluctuations in raw materials can affect overall project feasibility. To make RFB technology more attractive, ongoing research aims to identify cheaper alternatives for key materials, as well as improvements in production processes. Ultimately, reducing costs will be essential for rfb technology to compete effectively in the energy storage landscape.
Energy Density Limitations
Energy density is a critical parameter in evaluating the performance of any energy storage system. Redox flow batteries tend to have lower energy density compared to traditional battery technologies like lithium-ion batteries. This means RFBs can store less energy in a given volume or mass.
Lower energy density affects the practicality of RFBs in certain applications. For instance, in electric vehicles or small-scale devices, RFBs may not provide the necessary power-to-weight ratio. Researchers are actively working on developing new chemistries and optimizing designs to improve energy density without compromising other valuable characteristics, such as cycle life and safety.
Material Availability and Environmental Impact
The availability of materials used in redox flow batteries presents both a challenge and an opportunity. While some materials like vanadium are abundant, high-quality sources can be limited. This reliance on specific materials raises concerns about supply chain vulnerabilities. Moreover, as demand for RFB technology grows, the environmental impact of material extraction must be considered.
Extracting raw materials not only burden ecosystems, but can also have social implications. It's crucial to address these issues by sourcing materials sustainably. Furthermore, alternative battery chemistries that utilize more abundant or less harmful materials should be explored. By focusing on innovation and responsible sourcing, the overall impact of RFBs can be optimized, aligning better with global sustainability goals.
Applications of Redox Flow Batteries
Redox flow batteries (RFBs) present a unique proposition in energy storage systems. Their ability to effectively manage energy resources makes them vital for different sectors. The applications of RFBs are expansive. They range from enhancing grid stability to integrating renewable sources and catering to specific industrial needs.
Grid Energy Storage
Grid energy storage is one of the most crucial applications of redox flow batteries. These batteries facilitate the smooth operation of electrical grids by addressing the mismatch between energy supply and demand. RFBs can store excess electricity generated during off-peak hours and release it during peak hours, thus stabilizing the grid. This capability is significant as it helps prevent blackouts and allows for the integration of variable renewable energy sources such as wind and solar.
Furthermore, RFBs can operate at a larger scale compared to conventional batteries. Their modular design means that they can be expanded easily as demand increases. Notably, the long cycle life of RFBs minimizes the need for frequent replacements. In the context of grid energy storage, this leads to lower overall operational costs, making them an attractive option for utilities.
Renewable Energy Integration
The integration of renewable energy is another important area for redox flow batteries. As society transitions to greener energy sources, managing the variability in generation becomes paramount. RFBs can absorb energy from renewable sources during periods of high production and discharge when production wanes.
This characteristic of redox flow batteries makes them compatible with solar and wind installations. They ensure that the energy captured is not wasted. By acting as a buffer, RFBs enhance the overall reliability of renewable energy systems. As a result, renewable energy can contribute more effectively to the energy mix, supporting both sustainability goals and energy security.
Commercial and Industrial Use Cases
In commercial and industrial applications, redox flow batteries offer tailored solutions for energy management. Industries that require a reliable power supply can leverage RFBs for backup power, allowing them to maintain operations during outages. Also, they can reduce reliance on diesel generators, promoting eco-friendly practices in industrial environments.
Additionally, commercial facilities can utilize RFBs to manage their energy consumption more efficiently. By storing energy during off-peak rates, businesses can lower their energy costs and reduce their carbon footprint. Some industries are exploring the use of RFBs in electric vehicle charging stations, allowing for efficient energy distribution and utilization.
The versatility of redox flow batteries in different applications, from grid storage to industrial use, underscores their potential in advancing energy solutions.
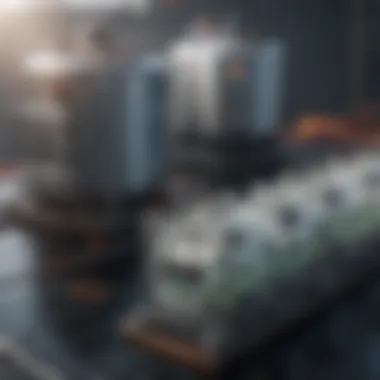
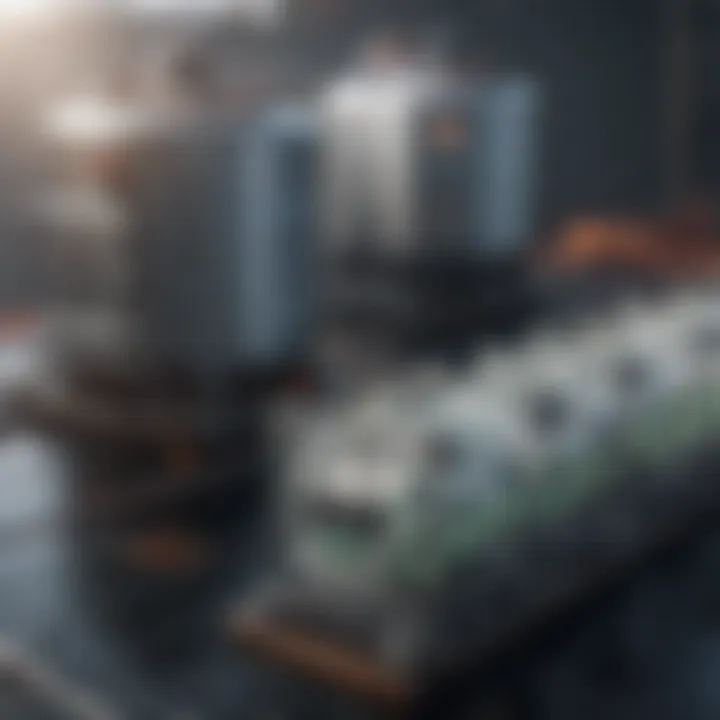
Comparative Analysis with Other Energy Storage Technologies
The comparative analysis of redox flow batteries with other energy storage technologies is essential for understanding their unique position within the energy landscape. As the demand for efficient, sustainable energy storage solutions continues to grow, evaluating the relative merits of each technology can guide stakeholders in choosing the optimal system for their needs. This analysis will particularly focus on two prominent technologies: lithium-ion batteries and lead-acid batteries. By examining these technologies, one can appreciate the advantages and limitations that redox flow batteries offer.
Lithium-Ion Batteries
Lithium-ion batteries are widely used in consumer electronics, electric vehicles, and energy storage systems. Their success stems from several factors.
- High Energy Density: They have a superior energy density compared to redox flow batteries, allowing them to store more energy in a smaller footprint.
- Efficiency: Lithium-ion batteries generally exhibit a high round-trip efficiency, typically around 90-95%.
- Established Technology: Due to decades of research and development, lithium-ion technology is well-understood and continuously improving.
However, there are notable drawbacks:
- Lifecycle Limitations: Lithium-ion batteries have a finite number of charge-discharge cycles, often requiring replacement after a few years.
- Cost Factor: Battery production and raw material costs, such as lithium, can be volatile, impacting overall economics.
- Safety Concerns: There are risks of thermal runaway, leading to fires and explosions in certain situations.
In contrast, redox flow batteries offer notable benefits:
- Long Cycle Life: They can endure thousands of cycles without significant degradation, making them ideal for applications requiring longevity.
- Scalability: The energy capacity can be easily adjusted by increasing the volume of the electrolyte, a flexibility not found in lithium-ion systems.
Lead-Acid Batteries
Lead-acid batteries have been around for over a century and are known for their robustness. These batteries are often used in automotive applications, UPS systems, and renewable energy storage.
- Cost-effectiveness: They remain one of the most affordable energy storage solutions, with lower initial capital costs.
- Mature Technology: Their technology is mature, and the recycling infrastructure for lead-acid batteries is well-established.
- Reliable Performance: They perform reliably in various temperatures and applications.
Nevertheless, lead-acid batteries possess limitations:
- Low Energy Density: They have lower energy density compared to redox flow and lithium-ion batteries, meaning more space is needed for the same storage capacity.
- Shorter Lifespan: Their lifecycle is also shorter, requiring more frequent replacement.
- Environmental Concerns: While recyclable, lead pollution can arise from improper disposal.
Through this analysis, it is clear that redox flow batteries stand out due to their unique characteristics. They do not match the energy density of lithium-ion or the cost-effectiveness of lead-acid batteries but provide extensive cycle life and flexibility that make them suitable for specific applications, particularly in larger-scale energy storage scenarios. Understanding these comparisons aids researchers and decision-makers in navigating the complexities of energy storage technologies.
Future Directions in Redox Flow Battery Research
The future of redox flow batteries (RFBs) is vital for advancing energy storage solutions. As the demand for energy efficiency grows, research into RFB technology becomes increasingly urgent. Current trends highlight several key areas where improvements can significantly enhance the performance and applicability of RFB systems.
Innovative Materials and Chemistries
Research into innovative materials has become central to the development of next-generation redox flow batteries. Improvements in electrolyte compositions and electrode materials are crucial for increasing energy density and efficiency. For example, the use of organic compounds as electrolytes has demonstrated potential. These materials can offer lower costs and environmental benefits compared to conventional metal-based systems.
Additionally, hybrid approaches that combine different redox couples could maximize the benefits of existing chemistries while reducing limitations. Such innovations may lead to higher efficiency in both energy storage and conversion processes.
"Investing in new materials can reshape the energy landscape, ensuring better performance of flow batteries in various applications."
Enhancements in Efficiency and Performance
Another essential direction for research is improving the efficiency of redox flow batteries. Reducing internal resistance is a significant focus area. Innovations in membrane technology may lower resistive losses, enhancing overall performance. Moreover, optimizing flow cell design is critical for effective ion transport, which directly influences the energy conversion efficiency.
Some studies explore the impact of temperature on performance, revealing that suitable adjustments can lead to significant improvements. Conducting research in real-world applications also highlights the effects of operational conditions. By understanding and mitigating these factors, researchers can achieve better performance metrics and reliability in commercial use.
Regulatory and Policy Impacts
The regulatory landscape significantly impacts research and development directions for redox flow batteries. As governments and organizations set sustainability goals, policies that support clean energy technologies can drive innovation. Standards for energy storage systems affect the marketβs growth and adoption rate, and understanding these guidelines is crucial for researchers and manufacturers.
Additionally, incentives for renewable energy storage solutions can accelerate the adoption of RFBs. Policymakers can foster a favorable environment for research funding and technology commercialization focused on RFB advancements. Collaboration between academia, industry, and regulatory bodies is essential to ensure that innovations align with policy objectives and sustainability goals.
Finale
The conclusion of this article underlines the significance of redox flow batteries (RFBs) within the broader context of energy storage and management. The vitality of RFB technology lies in its unique operational principles and structural design, making it a noteworthy contender in the renewable energy landscape.
Summation of Key Points
In this article, we have explored various dimensions of redox flow batteries, including their
- fundamental principles such as electrochemistry and energy storage mechanism,
- essential components like electrolytes, electrodes, and membranes,
- various types including vanadium and iron-chromium flow batteries,
- operational mechanisms during charging and discharging,
- the advantages of scalability, long cycle life, and inherent safety,
- the challenges related to costs, energy density, and material availability,
- the vital applications in grid energy storage, renewable energy integration, and commercial uses,
- a comparative analysis with lithium-ion and lead-acid batteries, and
- future directions that focus on innovative materials, efficiency enhancements, and policy impacts.
This synthesis of information highlights the multifaceted nature of redox flow batteries and provides insight into their potential role in addressing energy storage challenges.
Call for Future Research
The future of redox flow batteries is promising but requires further exploration. Key areas for research include
- development of new materials that enhance battery performance,
- investigation into cost-reduction strategies that could make RFBs more economically viable, and
- studies on environmental impacts related to material sourcing and disposal.
By prioritizing these avenues, the energy sector can better understand and harness the full potential of redox flow batteries. Advancing this technology could lead to breakthroughs in energy efficiency and sustainability, benefiting both consumers and the environment.
The continuous evolution of RFB technology represents a crucial step forward in renewable energy storage, emphasizing the importance of ongoing research and innovation.