The Molecular Paradigm: An In-Depth Exploration
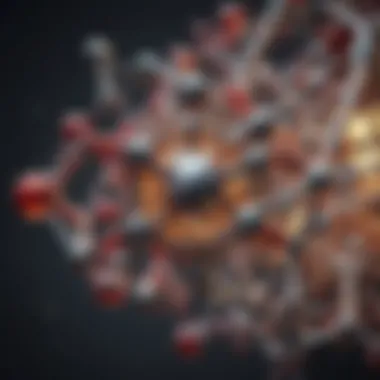
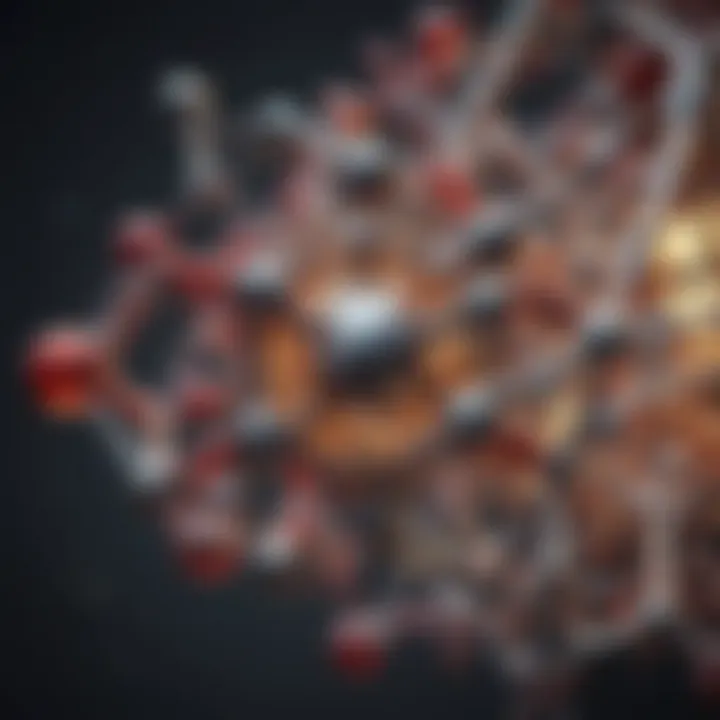
Intro
The study of molecules is an essential component of many scientific fields, offering insights into the fundamental mechanisms that govern chemical and biological processes. This article seeks to unveil the complexities of molecular structures and their significance. It outlines how molecules interact, and how their properties can be manipulated for various applications.
The exploration of molecules leads us to a deeper understanding of everything from genetics to materials science. Consequently, recognizing the molecular paradigm broadens the perspective on how life systems operate and how synthetic materials are developed.
Research Overview
Summary of Key Findings
Molecular analysis serves as a foundation for advancements in diverse fields such as pharmacology, biotechnology, and nanotechnology. Key findings from existing literature indicate that:
- Molecular structure directly influences biological function and chemical behavior.
- Interactions between molecules often dictate the pathways of metabolic processes and chemical reactions.
- Innovative tools, such as spectroscopy and microscopy, enhance our ability to study molecular phenomena effectively.
Research Objectives and Hypotheses
This article aims to thoroughly explore the principles underpinning molecular science. Specific objectives include:
- To define the fundamental concepts related to molecular structures.
- To analyze the various methods used for molecular examination.
- To project future trends in molecular research and its applications.
The hypotheses center on the assertion that advancements in molecular analysis will lead to significant breakthroughs in medicine and materials science.
Methodology
Study Design and Approach
A comprehensive review of existing literature and recent studies forms the core of this exploration. The design is qualitative, focusing on synthesizing knowledge from multiple disciplines. By juxtaposing findings from chemistry and biology, a nuanced understanding is formed that benefits both students and professionals.
Data Collection Techniques
Data is collected through systematic literature reviews from reputable sources, including peer-reviewed journals and scientific databases. This approach ensures that the information presented is credible and up-to-date. In addition, primary research articles will be referenced to provide firsthand insights into ongoing molecular studies.
"Understanding molecular interactions is key to harnessing their potential in technology and medicine."
Armed with this methodology, the article will illuminate various aspects of molecular science, making it accessible and informative for a high-IQ readership.
Foreword to Molecules
Understanding molecules is a cornerstone of many scientific disciplines. In this article, we will explore their roles, characteristics, and the implications they have across various fields. Molecules are not just the building blocks of matter; they are fundamental to everything from chemical reactions to biological processes.
Molecules can range from simple structures like carbon dioxide to complex proteins essential in cellular functions. By defining what a molecule is and exploring its historical development, we can better appreciate its significance.
Defining a Molecule
A molecule is defined as a group of two or more atoms bonded together. These atoms can be of the same or different elements. The simplest example of a molecule is a diatomic molecule like Oβ, which consists of two oxygen atoms. Molecules can be classified into two main categories: polar and nonpolar. The distinction largely depends on the distribution of electrical charge across the molecule.
Some common characteristics include:
- Bonding: Molecules are formed by covalent bonds, where atoms share electrons, or ionic bonds, where electrons are transferred.
- Stability: Molecules tend to reach a stable state, which means they are less reactive under normal conditions.
Historical Context
The concept of molecules dates back to ancient times but began to take shape in the 19th century. Early Greek philosophers theorized about indivisible particles, which later evolved into the atomic theory. In the early 1800s, John Dalton introduced a more systematic approach to atomic theory, which laid the groundwork for modern chemistry. Over the years, scientists like Dmitri Mendeleev and Avogadro expanded our understanding of molecular structures and behaviors.
Understanding this evolution helps us see how scientific knowledge is built over time and how interconnected the various scientific disciplines are.
Broader Implications in Science
The implications of molecules extend far beyond just chemistry. They play a pivotal role in numerous fields, including:
- Biology: Molecules such as DNA and proteins are essential for life.
- Physics: Understanding molecular interactions is vital for fields like thermodynamics and quantum mechanics.
- Environmental Science: Molecules impact ecosystems and climate through processes like photosynthesis and pollution.
"The study of molecules provides insights into not only how life functions but also how we can influence and improve our world through scientific understanding."
The study of molecular science offers crucial advantages. It opens avenues in drug development, nanotechnology, and materials science among others. As we explore various types and functions of molecules in the subsequent sections, we will gain a deeper understanding of their pervasive presence in our world.
Types of Molecules
The study of types of molecules is an essential aspect of molecular science. Understanding these categories helps in comprehending their diverse functions and interactions in various fields such as chemistry, biology, and medicine. Molecules can be classified based on their composition and characteristics, which can lead to significant implications in research and applications. This section will cover organic molecules, inorganic molecules, and biomolecules in detail.
Organic Molecules
Definition and Characteristics
Organic molecules are primarily composed of carbon atoms, often in combination with hydrogen, oxygen, nitrogen, and other elements. A key characteristic of organic molecules is their ability to form covalent bonds, creating complex structures such as chains or rings. This property lends them versatile functionalities, making them critical in biological systems.
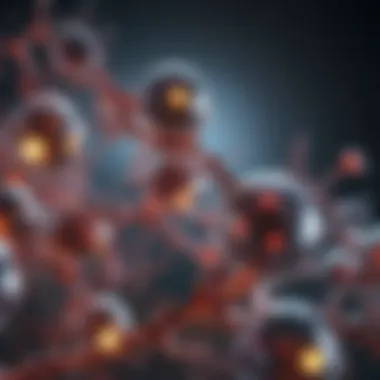
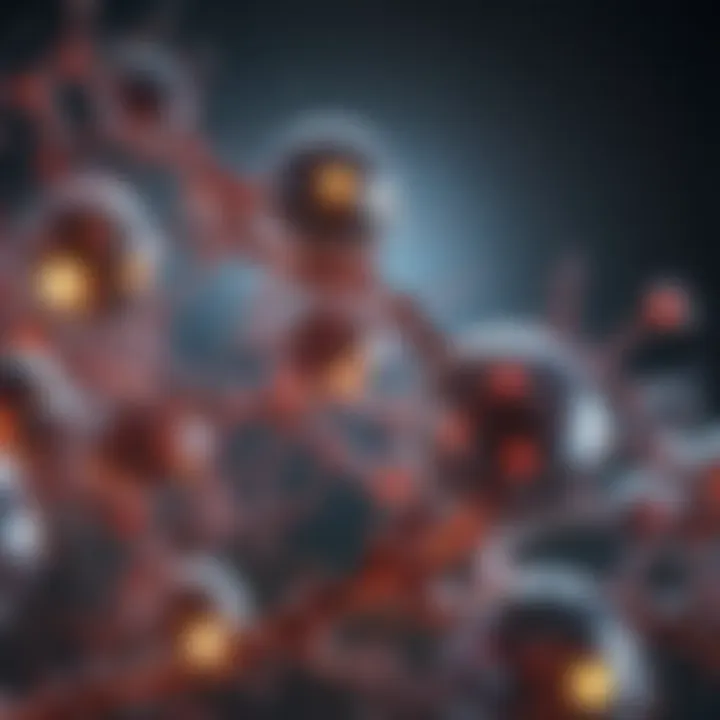
The unique feature of organic molecules is their structural diversity. This diversity enables a variety of biological processes, including metabolism and cellular respiration. Thus, their importance in this article cannot be overstated as they form the foundation of life.
Examples in Nature
Many organic molecules exist naturally and play vital roles in ecosystems. For instance, carbohydrates, proteins, and lipids are basic food molecules for humans and animals. Their presence in nature illustrates the importance of organic molecules in sustaining life processes.
One unique aspect of organic molecules is their susceptibility to reactions. This feature can be beneficial, leading to energy production in living organisms, but it can also result in instability under certain conditions. The balance between these benefits and drawbacks highlights the significance of organic molecules in both natural and experimental contexts.
Inorganic Molecules
Classification
Inorganic molecules are typically defined as those not containing carbon-hydrogen bonds. They encompass a wide range of substances, including salts, minerals, and metals. A key aspect of classification is dividing inorganic molecules into further categories, such as elements, compounds, and coordination complexes.
This classification is beneficial as it simplifies the study of reaction mechanisms involving inorganic substances. For this article, understanding the classification of these molecules helps delineate their specific properties and applications.
Reactivity and Stability
Reactivity in inorganic molecules varies significantly. Some inorganic compounds, like sodium chloride, are stable under a variety of conditions. Others, like hydrogen peroxide, can react unpredictably. The key characteristic of many inorganic molecules is their ability to act as catalysts or inhibitors in chemical reactions.
This trait is advantageous as it plays a crucial role in industrial applications, such as in the production of fertilizers or pharmaceuticals. However, the same reactivity can also lead to unwanted side reactions, emphasizing the need for careful study in contexts like material science or environmental chemistry.
Biomolecules
Proteins
Proteins are complex molecules composed of amino acids and play countless roles in biological systems. Their functional diversity stems from their structure, which can change according to the environment. This adaptability makes proteins critical for various biochemical processes.
A significant characteristic of proteins is their ability to catalyze biochemical reactions. Enzymes, which are proteins, speed up reactions and lower the energy required for these processes. However, they can also be sensitive to environmental shifts, such as temperature or pH, affecting their functionality.
Nucleic Acids
Nucleic acids, including DNA and RNA, are vital for storing and transmitting genetic information. Their unique structure allows for the formation of complex molecules capable of encoding information. This characteristic is central to all biological processes.
A key aspect of nucleic acids is their role in gene expression. Variations in sequences can lead to differences in protein synthesis, influencing traits of living organisms. While they are remarkably stable, changes in environment can cause breakdown or mutations, which are areas of active research in molecular biology.
Carbohydrates
Carbohydrates serve as primary energy sources for most living organisms. They are characterized by their structural simplicity, including sugars and polysaccharides. This feature makes them readily available for metabolism, providing energy as needed by cells.
The unique trait of carbohydrates is their ability to store energy efficiently. For instance, starch and glycogen are polysaccharides that serve as energy reserves. Their breaking down when energy is required showcases their essential function. However, excessive carbohydrate intake can lead to health issues, making protein and fat also important in a balanced diet.
"Molecular understanding is crucial for advancements in biotechnology and medicine. The types of molecules discussed here form the basis of this understanding."
Molecular Structure
Understanding molecular structure is fundamental to grasping the complexities of chemistry and biology. Molecular structure refers to the arrangement of atoms within a molecule, which determines its physical and chemical properties. The knowledge of molecular structure allows scientists to predict how molecules will interact. This includes reactions, stability, and biological activity.
Atomic Composition
Atomic composition is the first element in determining a molecule's structure. Each molecule is made up of atoms, which are the building blocks of matter. An atom consists of a nucleus, containing protons and neutrons, surrounded by electrons. The number and type of these atoms define the molecular formula. For example, water is composed of two hydrogen atoms and one oxygen atom, resulting in the formula HβO.
The specific elements that make up a molecule influence its reactivity and properties. For instance, carbon atoms can bond with various other atoms, leading to the vast diversity of organic compounds. This makes carbon the backbone of biological molecules.
Molecular Geometry
Molecular geometry is crucial because it describes the three-dimensional arrangement of atoms. This spatial arrangement directly impacts how a molecule behaves. Various molecular shapes, such as linear, bent, or tetrahedral, result from the bonding between atoms.
The geometry can be influenced by factors such as bond angles and the presence of lone pairs of electrons. Using VSEPR (Valence Shell Electron Pair Repulsion) theory assists in predicting these geometries. For example, methane (CHβ) has a tetrahedral shape, affecting its interaction with other molecules.
Polarity and Intermolecular Forces
Polarity is a critical aspect of molecular structure, influencing solubility and boiling points. A polar molecule has a distribution of electrical charge leading to partial positive and negative charges. Water is a classic example of a polar molecule, with strong hydrogen bonding between water molecules.
Intermolecular forces arise due to these charges, dictating how molecules behave in different environments. Forces such as hydrogen bonding, dipole-dipole interactions, and van der Waals forces contribute to various physical properties like viscosity and surface tension. Understanding these interactions aids in fields such as material science and biochemistry.
Key Takeaway: The molecular structure encompasses atomic composition, geometric shapes, and polarity, shaping the behavior and properties of substances in chemistry and biology.
Molecular Functions and Interactions
Molecular functions and interactions represent a core aspect of molecular science. This field encompasses various processes that govern how molecules engage with one another. Understanding these functions is crucial to grasp the dynamics of chemical reactions and the biological systems they affect. The interactions among molecules dictate the behavior of substances in biological, chemical, and environmental contexts.
One key benefit of studying molecular functions is its application in predicting reaction outcomes and biological activities. For researchers and practitioners, this knowledge can streamline the development of new materials and pharmaceuticals. Moreover, the interplay between molecular interactions contributes to innovations across various scientific domains.
Chemical Reactions
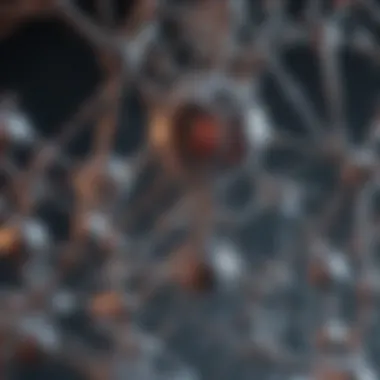
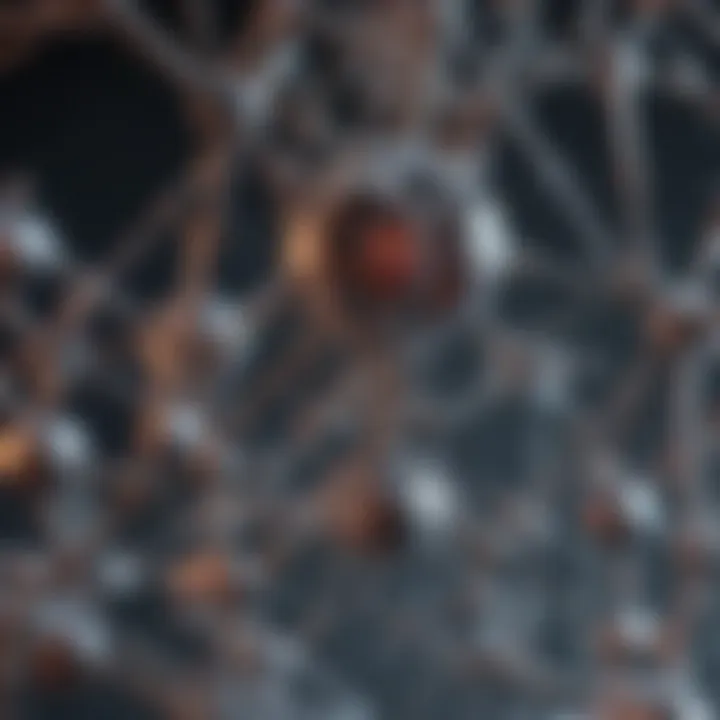
Reaction Mechanisms
Reaction mechanisms are essential for explaining how chemical transformations occur at the molecular level. They detail the step-by-step processes that lead to products from reactants. A specific aspect of reaction mechanisms is that they enable scientists to predict the outcomes of reactions more accurately. This predictive capability is vital in fields like organic chemistry, where understanding the flow of electrons can dictate the course of a reaction.
The key characteristic of reaction mechanisms is their intricacy. Different pathways can lead to the same product, making them a popular choice for critical analysis in this article. A unique feature of these mechanisms is their ability to provide insight into the stability of reaction intermediates, which can have substantial influences on reaction rates. One advantage is that understanding these pathways allows chemists to manipulate conditions for desired outcomes. However, elucidating complex mechanisms can also present challenges, requiring sophisticated techniques and deep knowledge.
Catalysis
Catalysis refers to the process of increasing reaction rates by introducing a substance known as a catalyst. In the realm of chemical reactions, this aspect is significant for enhancing efficiency and reducing energy consumption. Catalysis is especially crucial in industrial applications where large-scale reactions are conducted.
The key characteristic of catalysis is its transformative ability without being consumed in the reaction. This property makes it a beneficial approach for sustainable chemistry, reflecting its emphasis on lowering environmental impact. A unique feature of catalysis is the specificity of catalysts, which can streamline reactions to yield specific products, reducing byproducts. The advantage lies in its ability to facilitate reactions under milder conditions, often making processes more economical. However, one disadvantage may be the challenges in developing highly selective catalysts for certain reactions, which remains a prominent area of research.
Biological Interactions
Biological interactions are fundamental to understanding various life processes. They include interactions between molecules that result in crucial biological functions. These interactions can dictate physiological responses and influence cellular mechanisms.
Enzyme-Substrate Complexes
Enzyme-substrate complexes represent a vital aspect of molecular functions. This refers to the transient association between an enzyme and its substrate, resulting in a biochemical reaction. The specific aspect of enzyme-substrate complexes is their precision in catalyzing reactions. This precision is critical for maintaining metabolic pathways and ensuring cellular function.
The key characteristic of these complexes is their specificity. Each enzyme typically binds to a specific substrate, leading to a reaction that the enzyme enhances. Recognizing these dynamics is a beneficial aspect for this article as it highlights the significance of enzymes in biochemical processes. A unique feature is the formation of an intermediate state, which can further stabilize the reaction pathway. One advantage is that enzyme kinetics can often be manipulated, enhancing the efficiency of various biological processes. However, a disadvantage may include the conditions under which enzyme activity can decrease, such as changes in pH or temperature.
Signal Transduction
Signal transduction refers to the process by which cells respond to external signals through networks of molecules. This aspect is crucial for cellular communication and the resulting responses, such as growth or stress reactions. Signal transduction pathways are complex and involve multiple interactions, representing a specific aspect of molecular biology.
The key characteristic of signal transduction is its ability to amplify a signal, leading to a significant cellular response. This topic is relevant in this article because it emphasizes the importance of molecular interactions in orchestrating biological activities. A unique feature is the presence of feedback mechanisms, allowing cells to regulate responses effectively. Advantages include cellular adaptation and coordination of physiological processes, but disadvantages can arise from dysregulation, leading to diseases such as cancer.
Understanding molecular functions and interactions helps to illuminate the intricate dance of biology and chemistry, paving the way for innovations in various scientific fields.
Techniques in Molecular Analysis
Understanding molecules requires a range of analytical techniques. These methods allow scientists to determine molecular structure, composition, and interactions. The techniques provide vital information that underpins advances in various fields like chemistry, biology, and materials science. Knowing how to effectively use these methods can lead to significant discoveries and developments.
Spectroscopy Methods
NMR Spectroscopy
Nuclear Magnetic Resonance (NMR) Spectroscopy is a powerful technique for determining the structure of organic compounds. It works by applying a strong magnetic field to nuclei in molecules. This interaction provides information about the environment surrounding specific atoms, particularly hydrogen and carbon.
A key characteristic of NMR Spectroscopy is its non-destructive nature. Researchers can study samples without altering them. This aspect is particularly valuable in fields like drug development, where preserving the integrity of samples is crucial. The unique feature of NMR is its ability to offer detailed insights into molecular dynamics and geometry.
However, NMR has its disadvantages. It often requires relatively large samples and can be less effective with complex mixtures. Additionally, interpreting NMR spectra can be challenging for novices, requiring expertise for accurate analysis.
Mass Spectrometry
Mass Spectrometry (MS) is another essential technique in molecular analysis. This method measures the mass-to-charge ratio of ions to identify chemical substances present in a sample. Its contribution to molecular research is significant, as it allows for the analysis of complex mixtures, enabling the identification of unknown compounds.
A primary advantage of MS is its sensitivity. It can detect very low concentrations of substances, which is particularly beneficial in fields like forensic science and environmental studies. The unique feature of Mass Spectrometry is its ability to provide structural information about molecules through fragmentation patterns.
Yet, Mass Spectrometry also has limitations. Sample preparation can be time-consuming and may result in the loss of volatile compounds during the process. Additionally, the interpretation of mass spectra requires significant experience for reliable results.
Molecular Imaging Techniques
Molecular imaging techniques provide visual representations of molecules, enhancing our understanding of their spatial relationships and dynamics. These methods are crucial for studying structures at the atomic level and contribute significantly to fields such as drug development and materials science.
X-Ray Crystallography
X-Ray Crystallography is a cornerstone technique for determining the three-dimensional structure of crystalline substances. By analyzing how X-rays scatter when they hit a crystal, researchers can uncover the arrangement of atoms within a molecule. This method has played a pivotal role in understanding biomolecules, like proteins and nucleic acids, providing insights into their function and interactions.
A critical characteristic of X-Ray Crystallography is its ability to provide high-resolution structural data. It is widely regarded in the scientific community for its accuracy. The unique feature is the requirement of crystalline samples, which can sometimes limit its application to compounds that can be crystallized.
One of the disadvantages is that not all substances can form suitable crystals. This limitation necessitates alternative methods for those compounds lacking crystalline structure.
Electron Microscopy
Electron Microscopy (EM) is another advanced imaging technique used to study molecules and materials at very high resolutions. By utilizing electron beams, EM achieves significantly higher magnification than light microscopy. This capability is essential for visualizing small biological samples, such as cells and viruses.
A key characteristic of Electron Microscopy is its unparalleled resolution. Researchers can observe fine details of cellular structures and molecular arrangements. The unique feature is the ability to visualize live specimens under specific conditions.
However, Electron Microscopy has its drawbacks. Sample preparation can alter properties, and the technique often requires a vacuum environment. This factor can prevent the observation of live processes directly. Moreover, interpreting electron micrographs demands considerable expertise, which can limit accessibility for less experienced researchers.
Applications of Molecular Science
The exploration of molecular science has profound implications across numerous fields, including biology, medicine, and materials science. Understanding how molecules interact and function can lead to significant advancements in these areas. This section elucidates the various applications of molecular science, with an emphasis on nanotechnology and drug design.
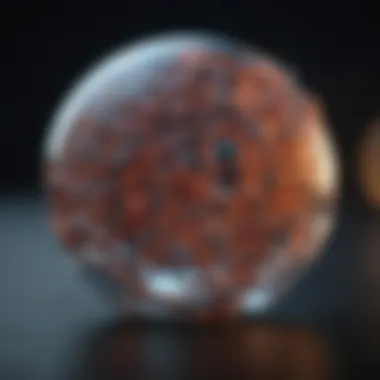
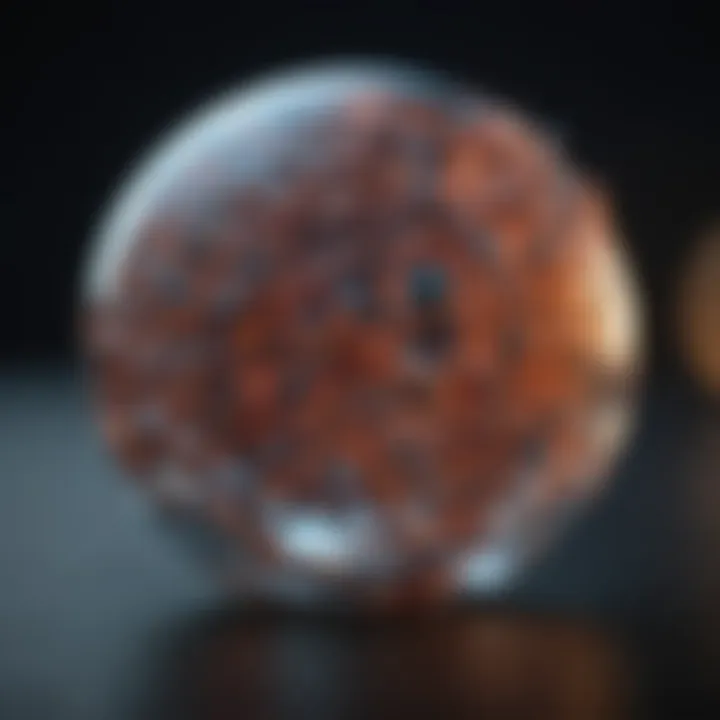
Nanotechnology
Nanoscale Materials
Nanoscale materials refer to structures that exist on the scale of 1 to 100 nanometers. These materials exhibit unique physical and chemical properties compared to their bulk counterparts, primarily due to their high surface area to volume ratio. One key characteristic of nanoscale materials is their ability to enhance reactivity and strength. This makes them a popular choice for many applications across different fields, including electronics, energy storage, and biomedicine.
Nanoscale materials are beneficial in the context of this article because they open avenues for innovation that were not previously possible at larger scales. A distinctive feature of these materials is their ability to be engineered for specific applications, such as targeted drug delivery systems in medicine. While their advantages include improved functionality and efficiency, there are also concerns regarding environmental impact and biocompatibility that have to be carefully considered.
Applications in Medicine
The importance of nanoscale materials extends into medicine, where they have transformative potential. Nanoscale structures can be used to improve drug delivery systems. This aspect of application is crucial for developing therapies that require precise targeting of diseased cells while minimizing side effects on healthy tissues. A vital characteristic of applications in medicine is their ability to enhance imaging and diagnostic methods, allowing for better detection of diseases at an earlier stage.
The unique features of medical applications include their capability for real-time monitoring of drug administration and targeting specific cell types. The advantages here are substantialβimproved therapeutic efficacy and reduced side effects. However, challenges such as regulatory hurdles and long-term safety must not be overlooked in their development.
Drug Design and Development
Structure-Activity Relationships
Structure-activity relationships (SAR) define the relationship between the chemical structure of a molecule and its biological activity. This understanding is crucial for rational drug design, where scientists aim to optimize molecules to maximize their therapeutic effectiveness. A key characteristic of SAR is its ability to predict how changes in molecular structure can affect activity, making it a valuable tool in pharmaceutical research.
SAR is beneficial to this article as it enables a systematic approach to developing new drugs, reducing trial-and-error methods. The distinctive feature of structure-activity relationships lies in their predictive power, which allows scientists to design better-targeted compounds. However, the disadvantage can be that relying solely on computational models may not account for the complexity of biological systems, leading to potential misinterpretations.
Biopharmaceuticals
Biopharmaceuticals are products derived from biological sources, including proteins, nucleic acids, and living cells. They represent a growing sector in medicine, with a significant contribution to therapeutic solutions. A key characteristic of biopharmaceuticals is their tailored approach to treatment, often involving complex molecules that can address specific pathways in diseases. This specificity makes them a popular choice for modern medicine.
The unique feature of biopharmaceuticals is their ability to provide targeted therapies, such as monoclonal antibodies designed to bind to particular antigens on cancer cells. While the advantages of biopharmaceuticals include high efficacy and fewer side effects than traditional medications, their complexity in manufacturing and higher cost pose challenges that need consideration.
"The applications of molecular science, particularly in nanotechnology and drug design, are set to redefine therapeutic landscapes and improve patient outcomes."
Future Directions in Molecular Research
The realm of molecular research is at a transformative juncture, driven by advancements in technology and a deeper understanding of biological systems. As scientists explore the intricacies of molecules, the future appears promising. It promises not only innovation in existing fields but also the emergence of novel disciplines. This section will illuminate key areas shaping future molecular research, emphasizing the significance of emerging technologies and the evolving landscape of molecular medicine.
Emerging Technologies
CRISPR and Gene Editing
CRISPR technology has revolutionized the field of molecular biology. It allows for precise alterations in DNAβproviding a tool for researchers to edit genes with unprecedented accuracy. One key characteristic of CRISPR is its simplicity; it employs a guide RNA to direct the Cas9 enzyme to specific DNA sequences, resulting in efficient gene modifications. This technology has gained popularity for its potential applications, ranging from agriculture to complex disease treatments.
The unique feature of CRISPR lies in its adaptability. Researchers can customize guide RNAs to target almost any gene of interest, which enhances its versatility in various biological systems. The advantages include the feasibility of large-scale genetic modifications and the potential to correct genetic defects. However, challenges remain, including off-target effects, which could lead to unintended genetic alterations.
Computational Chemistry
Computational chemistry represents another frontier in molecular research. This discipline utilizes computer simulations to model molecular behavior and predict interactions. A key characteristic of computational chemistry is its ability to minimize trial-and-error experimentation. It enables researchers to explore vast chemical spaces efficiently.
The technology's unique feature is the predictive power it offers. By using quantum mechanics and molecular mechanics, scientists can simulate complex reactions and gain insights before conducting laboratory experiments. This can save significant time and resources. Nonetheless, the accuracy of models is contingent on the quality of computational methods and the assumptions made in simulations, which highlights an inherent limitation of this approach.
Molecular Medicine
Personalized Medicine
Personalized medicine strives to tailor medical treatment based on individual patient characteristics. This focus on specific genetic, environmental, and lifestyle factors marks a shift away from the traditional one-size-fits-all model. By analyzing genetic information, healthcare providers can develop more effective treatment plans that consider individual variability.
A key characteristic of personalized medicine is its reliance on genomic data. By utilizing genetic sequencing and bioinformatics, researchers can pinpoint mutations linked to diseases. The unique aspect of personalized medicine lies in its potential to optimize therapeutic efficacy and minimize adverse effects. However, ethical concerns related to genetic privacy and the high costs of genetic testing are vital considerations that cannot be overlooked.
Targeted Therapies
Targeted therapies represent a sophisticated approach to treating diseases, especially cancer. This method involves using drugs designed to specifically attack molecular targets associated with particular diseases. One defining feature of targeted therapies is their precision; they aim at specific molecular pathways or targets, leading to fewer side effects compared to conventional therapies.
The advantage of targeted therapies is their ability to disrupt disease mechanisms at a molecular level, potentially resulting in improved treatment outcomes. However, one must also consider the challenges involved, such as the potential for resistance, where tumors may adapt to evade targeted interventions. Understanding these nuances is essential for developing more effective therapeutic strategies.
"The intersection of emerging technologies and molecular medicine paves the way for innovative solutions to complex health challenges."
Closure
The significance of concluding this exploration into molecular science cannot be overlooked. This final section synthesizes the insights gained throughout the article, reiterating the essential points and emphasizing their relevance to various fields of study. Understanding molecules is not merely an academic exercise; rather, it is foundational to advancements in chemistry, biology, physics, and technology. The discussions highlight that molecular interactions dictate a host of essential processes in living organisms, enabling researchers to delve into areas such as drug development and genetic engineering.
Moreover, the role of molecules extends beyond biological systems. In materials science, for instance, molecular composition informs the properties and behaviors of new materials, shaping innovations in nanotechnology. This dual significance illustrates why continuous research in molecular science is imperative.
Summary of Key Points
- Broad Importance: Molecules are fundamental to all scientific disciplines.
- Types of Molecules: Understanding organic, inorganic, and biomolecules reveals their diverse roles.
- Molecular Structure and Characteristics: The arrangement and interactions of atoms define the molecule's behavior and function.
- Applications: Knowledge of molecular properties influences real-world applications, such as in nanotechnology and medicine.
- Future Roles: Emerging technologies will continually reshape our understanding of molecular principles.
The Importance of Continuous Research
The field of molecular science is always evolving. As scientists uncover more about molecular interactions and behaviors, new applications and implications emerge. Continuous research is crucial for several reasons:
- Innovation Generation: Research drives innovation in advanced materials, pharmaceuticals, and biotechnologies.
- Understanding Complex Systems: The complexity of molecular interactions requires constant study to unravel new pieces of knowledge.
- Global Challenges: Many global challenges, like environmental sustainability and healthcare, can be addressed through better understanding of molecular science.
- Educational Advancement: Ongoing research enriches education, providing students and professionals with up-to-date knowledge and techniques.
"The study of molecules is indispensable for advancing modern science and addressing pressing global issues."
For more information on molecular science, visit Wikipedia or Britannica.