The Essential Role of Microscopes in Biological Research
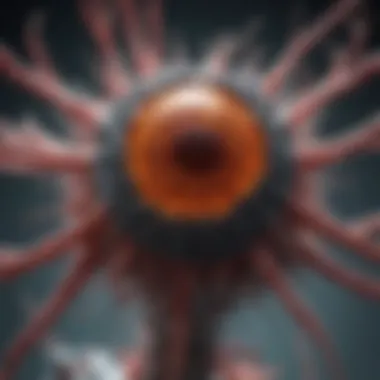
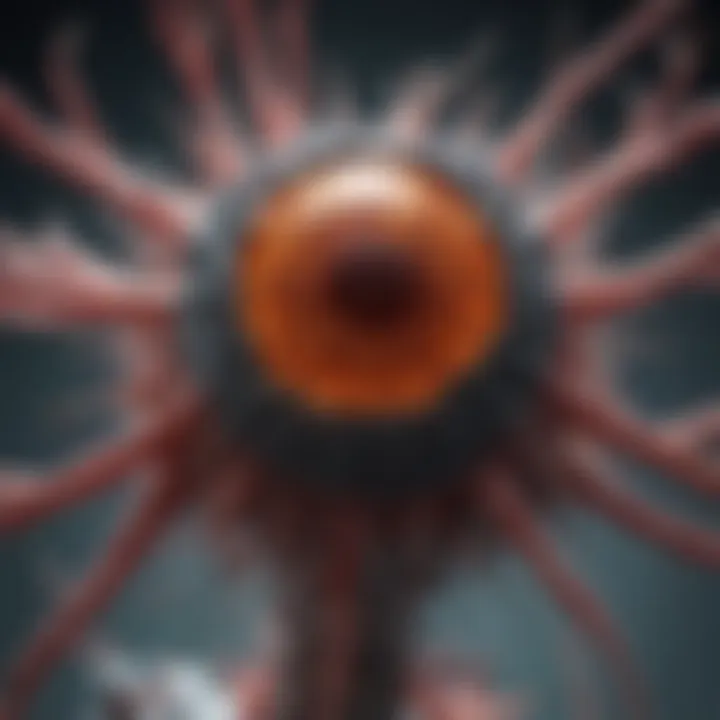
Intro
Microscopes have fundamentally changed the landscape of biological research. They offer scientists the ability to observe and analyze structures that are invisible to the naked eye. This advanced technology plays a crucial role in several disciplines, ranging from cell biology to microbiology. Understanding the different types of microscopes, their operational principles, and their applications is essential for anyone involved in biological research.
This article will present a detailed exploration of microscopy. We will examine the historical development of these instruments, the rise of digital imaging technologies, and the unique biological specimens that microscopes can reveal. Additionally, we aim to confront various challenges researchers may encounter within microscopy. Lastly, we will contemplate the future directions for microscopic techniques, considering their potential impacts across numerous biological disciplines.
By comprehensively discussing these topics, we hope to illuminate the indispensable role microscopes fulfill in advancing biological knowledge and research.
Prelude to Microscopy in Biology
Microscopy serves as the backbone of biological research, offering tools that allow scientists to delve into the world of the microscopic. Without these instruments, understanding biological structures and functions at the cellular level would remain elusive. The introduction of advanced microscopic techniques has transformed how researchers approach various biological inquiries.
Understanding Microscopy
Microscopy can be defined as the science of observing objects that are too small to be seen with the naked eye. It utilizes various technologies to magnify images of biological specimens, enabling researchers to explore cellular structures, tissues, and microorganisms in detail. Microscopes can provide images at various resolutions and magnifications, revealing intricacies of life on a scale that is almost invisible in everyday observation.
There are several types of microscopy, including light microscopy, electron microscopy, and fluorescence microscopy, each with unique properties and applications. Light microscopes use visible light to detect specimens, while electron microscopes employ electrons for higher resolution imagery. The precise understanding of these methodologies helps researchers select the appropriate tools for their specific needs.
Importance of Microscopes in Biology
Microscopes are crucial in biological research for several reasons:
- Detailed Analysis: They allow for in-depth analysis of cell structure and organization. Understanding how cells function is fundamental to biology. For instance, studying the cellular organization of cancer cells can lead to insights into tumor behavior.
- Pathogen Research: In microbiology, identification of pathogens relies heavily on microscopic techniques. Determining the characteristics of bacteria and viruses has critical implications for public health and medicine.
- Histological Studies: In fields like histology and pathology, microscopes help identify abnormalities in tissue samples. This is essential for diagnosing diseases and understanding their progression.
In numerous biological disciplines, microscopy offers a lens into phenomena otherwise hidden from view. The ability to visualize cellular processes has propelled advances in genetics, molecular biology, and cellular biology. Overall, the role of microscopes in biology cannot be overstated; they are indispensable in driving forward research and expanding our understanding of life itself.
Types of Microscopes
The examination of various types of microscopes is crucial in understanding their unique capabilities and applications in biological study. Each type serves specific needs, evaluated based on factors such as resolution, magnification power, and ease of use. Knowledge of these devices allows researchers and students alike to select the best option for their particular inquiries, thus advancing overall scientific endeavors.
Light Microscopes
Light microscopes are perhaps the most common type used in biological research. They operate using visible light and lenses to magnify specimens. Generally, these microscopes can magnify objects up to 1,000 times their original size. As a result, they can clearly display various cellular structures. The simplicity of operation makes light microscopes accessible for classroom settings and laboratories. They are particularly valuable for examining live cells, offering real-time observations of biological processes like cell division.
The primary components include an objective lens and an eyepiece. The magnification depends on the combination of these two lenses. Also, preparing the sample is often less complicated than other microscope types. Staining techniques can enhance visibility, allowing researchers to highlight specific structures and study morphology.
Electron Microscopes
Electron microscopes offer much higher resolution than light microscopes because they use electron beams instead of light. This advancement allows for magnifications of up to 10,000,000 times, revealing intricate details of cellular structures. There are two types of electron microscopes: transmission electron microscopes (TEM) and scanning electron microscopes (SEM).
TEMs provide insights into the internal architecture of cells by transmitting electrons through samples. They are instrumental in studying organelles and viruses. In contrast, SEMs scan the surface of samples, providing three-dimensional images that represent surface morphology. However, the need for vacuum conditions and intensive sample preparation means that researchers often cannot observe live specimens. Despite this limitation, electron microscopy remains indispensable in numerous fields, particularly for structural biology.
Confocal Microscopes
Confocal microscopes represent a significant advancement in imaging techniques, employing laser sources for illumination. These microscopes create sharp images by using a pinhole to eliminate out-of-focus light. Therefore, they enable researchers to obtain high-resolution images across various depths of a specimen, constructing 3D reconstructions with remarkable clarity.
Confocal microscopes shine in studying complex structures, such as tissues. They are frequently used in biological research to visualize cells in their native environment. A key distinction lies in their ability to combine fluorescence techniques, allowing for observation of multiple cellular components simultaneously. This unique feature adds versatility in assessing interactions within and among cells.
Fluorescence Microscopes
Fluorescence microscopes harness the properties of fluorescent stains and dyes to visualize specimens. When subjected to specific wavelengths of light, these stains emit light at a different wavelength. This unique process enables researchers to target specific proteins or cellular structures, offering insights into dynamic processes such as protein interactions and localization.
Utilizing fluorescence microscopy requires precise selection of filters that match the fluorescent tags used. These microscopes can effectively visualize live and fixed samples, making them essential in molecular biology and cell biology. Common applications include evaluating gene expression and tracking cellular components throughout life cycles.
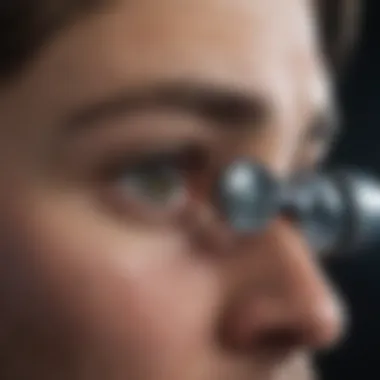
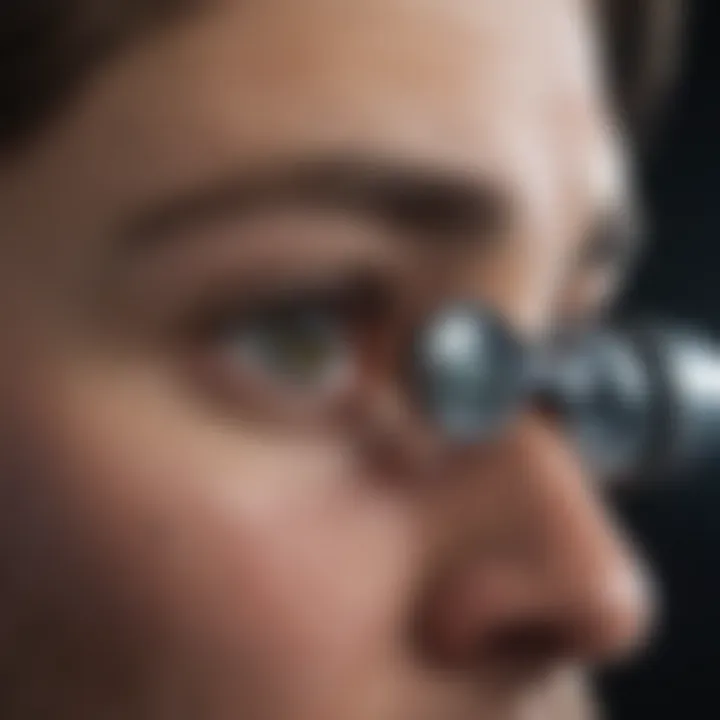
Digital Microscopes
Digital microscopes incorporate visual displays and imaging software directly into their design. The integration of digital imaging offers intuitive interaction, as researchers can view and capture images on monitors. Often used in educational and research contexts, digital microscopes facilitate easy sharing of findings.
Moreover, many digital microscopes allow for image enhancement and detailed analysis through their associated software. They can provide real-time measurements, annotations, or image processing, making them valuable tools. Their once-basic function has rapidly evolved into a powerful and versatile instrument in microscopy, bridging gaps in more classical methods.
"The evolution of microscopy technologies signifies the continuous pursuit of clarity and understanding in biological research."
Principles of Microscopy
Understanding the principles of microscopy is fundamental for anyone involved in biological research. These principles not only provide the basis for how microscopes function but also highlight their essential role in enhancing biological understanding. A solid comprehension of microscopy principles allows researchers to select the right tools for their work, whether examining cellular structures or studying microbial interactions. Additionally, knowledge of these principles aids in troubleshooting common issues faced during microscopic observation.
Basic Principles of Light Travel
Light microscopy operates on straightforward but critical principles of light travel. When light passes through a sample, it interacts with the sample's components, which may absorb, reflect, or refract the light. This interaction is crucial for creating images. Understanding how light travels and behaves is essential for optimizing image quality.
Light can be transmitted directly through samples such as thin sections of tissues or bacteria. In contrast, thicker samples require additional techniques like illumination adjustments. Contrast is enhanced through techniques such as phase contrast or differential interference contrast. These methods make it possible to see transparent or unstained specimens. Light travel is the foundation of using all light microscopy techniques.
Resolution and Magnification
Resolution and magnification are key concepts in microscopy. Both factors determine the clarity and detail of the images produced.
- Resolution refers to the ability of a microscope to distinguish two separate points as distinct entities. It is influenced by the wavelength of light used and the numerical aperture of the objective lens. Higher resolution allows scientists to observe finer details within a sample, which is crucial in many fields of biological research.
- Magnification is the process of enlarging an image of a sample. It can make objects appear much larger than they really are, aiding researchers in detailed examination. However, enhanced magnification without adequate resolution can lead to blurry images. This is why resolving power is as important as magnification power.
"To achieve effective microscopic imaging, one must balance resolution and magnification to analyze biological systems accurately."
In summary, the principles of microscopyβlight travel, resolution, and magnificationβare vital in the field of biological research. They empower researchers to unlock the secrets of cellular and microbiological worlds, leading to advancements in science and medicine.
Historical Development of Microscopy
The historical development of microscopy marks a pivotal journey in the evolution of biological sciences. Understanding the trajectory of microscope technology is essential. It provides insights on how scientific principles have advanced, enabling biologists to explore and elucidate the intricacies of life forms at microscopic levels. This section will review early microscopy inventions and the subsequent advancements that have shaped current practices.
Early Microscopy
The inception of microscopy dates back to the late 16th century. The earliest microscopes were rudimentary glass lenses, invented by figures like Hans Janssen and Zacharias Janssen. They crafted the first known compound microscope. Their design featured multiple lenses, which significantly improved the ability to magnify small objects.
Subsequently, Anton van Leeuwenhoek played a crucial role in microscopy's early development. Using his handcrafted single-lens microscopes, he observed microorganisms, thereby opening doors to microbiology. His detailed documentation of observations in the late 1600s led to groundbreaking discoveries, including his description of bacteria and sperm cells. This foundational work underlined the potential of microscopy in biological research, showcasing how unseen life forms could be studied.
Advancements in Microscopic Technology
As scientific knowledge progressed into the 19th and 20th centuries, microscopy underwent significant advancements. The introduction of achromatic lenses by Joseph Jackson Lister helped in overcoming chromatic aberration, enhancing image clarity. The invention of the electron microscope in the 1930s by Ernst Ruska was revolutionary. This technology allowed scientists to visualize entities at a cellular and subcellular level, expanding the horizon of biological research.
Recent advancements continue to refine microscopy techniques. For instance, the development of fluorescence microscopy has enabled researchers to tag specific cells or cellular components with fluorescent markers. This method facilitates the exploration of complex biological processes in real time.
Moreover, digital imaging technology has further enhanced microscopy's utility. High-resolution images can now be captured and analyzed using specialized software. This not only improves the accuracy of observations but also supports quantitative analysis in research.
In summary, the historical evolution of microscopy reflects a continuous quest for clarity and understanding in biological exploration. Each advance builds upon earlier discoveries, creating a complex tapestry of innovation that continues to guide contemporary biological research.
Applications of Microscopy in Biological Research
The application of microscopy in biological research is significant. It provides tools for detailed examination of various biological structures that are not visible to the naked eye. Microscopy aids in the identification and analysis of cellular components, microorganisms, and tissue architecture. This level of examination is important for advancing scientific knowledge, enhancing diagnostics, and developing treatments.
Cell Biology
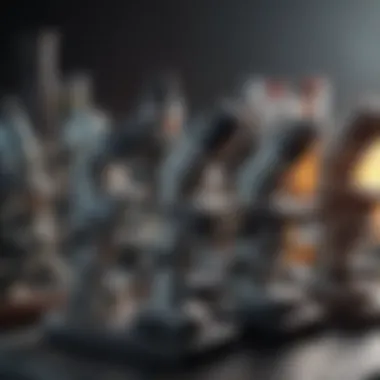
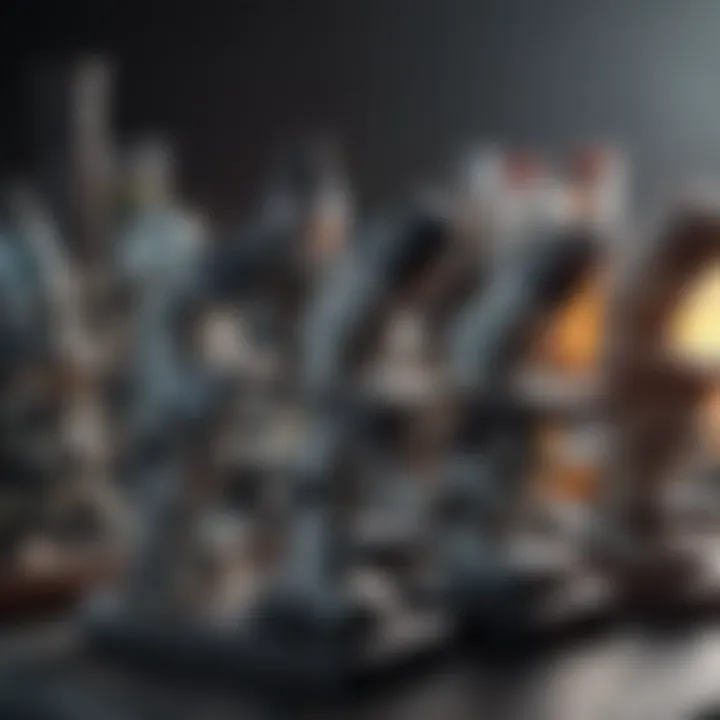
Cell biology heavily relies on microscopy. It allows researchers to view and study cells in their environment, understand cellular processes, and observe interactions. Light microscopy is often utilized for this purpose, providing a view of live cells and their dynamics. With higher magnification techniques, such as electron microscopy, structures like organelles can be examined in detail.
Key benefits include:
- Real-time observation: Scientists can monitor cell behaviors over time.
- Detailed structure analysis: Fine features can be analyzed, such as the nucleus or cell membrane.
The advances in fluorescence microscopy have made it easier to tag and watch proteins within live cells. This has resulted in numerous discoveries in how cells communicate and function.
Microbiology
In microbiology, microscopes are indispensable for the study of microorganisms like bacteria, viruses, fungi and protists. Standard light microscopes enable researchers to examine samples, but electron microscopes grant deeper insights at much higher resolutions.
Applications include:
- Pathogen identification: Diagnosing infections by analyzing pathogen morphology.
- Study of microbial behavior: Understanding growth patterns, reproduction and interactions with hosts.
The use of specialized staining techniques allows for differentiation between various cell types. For example, Gram staining helps in classifying bacteria into two major groups, which is crucial for treatment decisions.
Histology and Pathology
Microscopy is also critical in histology and pathology. Studies of tissues allow for the understanding of abnormalities, disease presence, and cellular organization. The process involves thinly slicing tissue specimens and applying specific stains to highlight different cell types and structures.
In pathology, this knowledge is applied to diagnose diseases. The main uses are:
- Cancer detection: Identifying malignant cells through tissue examination.
- Understanding tissue responses: Evaluating how tissues react to injury or disease.
Overall, the applications of microscopy in biological research serve as foundational methodologies that support ongoing research and practical applications such as medical diagnosis and therapeutic advances. Incorporating these technologies enhances the understanding of life at the microscopic level.
Challenges in Microscopy
Microscopy, despite its crucial role in biological research, presents numerous challenges that can impact the accuracy and reliability of results. These challenges stem from various procedural, technical, and inherent limitations within microscopy techniques. Addressing these obstacles is essential for ensuring that researchers can obtain precise and reproducible data in their studies.
Preparation of Samples
The first challenge in microscopy lies in the preparation of samples. This step is paramount, as it determines how well cellular structures or biological materials can be visualized under the microscope. An improper sample preparation can lead to distorted images or misinterpretations of what is observed.
Key aspects to consider include fixation methods, staining protocols, and sectioning techniques. Fixation helps preserve tissue structure but can introduce artifacts if done incorrectly. For example, over-fixation may cause tissues to become too rigid, complicating further analysis. Additionally, the choice of stains can significantly influence the final result. Some stains may emphasize certain cellular components while obscuring others, leading to incomplete data presentation.
Moreover, each biological sample type may require specific preparation techniques. For instance, preparing a plant cell will differ from preparing a bacterial culture. This variability demands that researchers thoroughly understand the biology of the samples they are studying.
Artifact Preamble
Another significant challenge in microscopy is the potential introduction of artifacts during imaging. Artifacts are misleading features that do not represent the actual structure being examined. They can occur due to several factors, including sample preparation, imaging settings, or the inherent characteristics of the microscopy technique used.
Artifacts can obscure or alter critical data, such as cellular morphology or spatial relationships. Common types of artifacts include:
- Staining artifacts: Overstaining or uneven distribution of stains can mislead interpretation.
- Optical artifacts: Optical effects may arise from the microscope's lens system, leading to incorrect perceptions of depth or focus.
- Mechanical artifacts: Handling samples or using damaged slides can introduce unforeseen variables into an experiment.
It is thus essential for researchers to be aware of these artifacts and consider them when analyzing results. Developing rigorous standards for sample preparation and using control samples can help mitigate the impact of these artifacts.
Addressing challenges in microscopy not only improves the reliability of results but also enhances the overall credibility of biological research.
Advancements in Digital Imaging Techniques
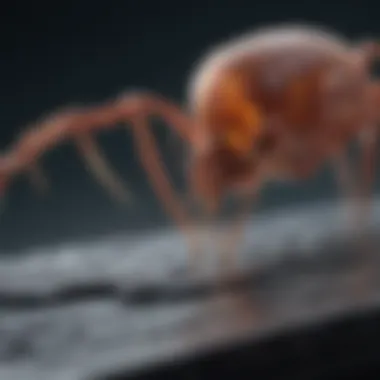
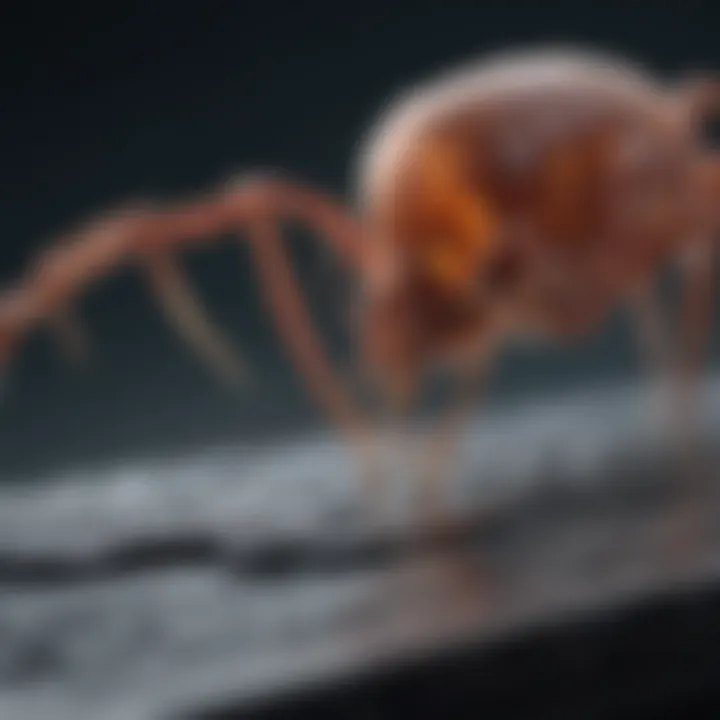
Advancements in digital imaging techniques have transformed the field of microscopy. These innovations enhance the capability to capture and analyze biological specimens with unprecedented clarity and detail. Digital imaging plays a crucial role in biological research by facilitating high-resolution image capture, efficient data management, and improved accessibility for scientists.
One significant benefit of digital imaging is the ability to easily store and retrieve images. Unlike traditional film methods, digital formats allow researchers to manage vast amounts of data without degradation of quality. This has particular implications in longitudinal studies where tracking changes over time is essential. Moreover, files can be compressed, facilitating easier sharing among researchers across the globe,
Recent digital techniques also provide advanced imaging modalities. This includes phase contrast imaging, time-lapse imaging, and hyperspectral imaging. These methods enable scientists to observe dynamic cellular processes in real-time, thus offering insights that were previously unattainable by static imaging approaches.
Importantly, the advancements in this area go beyond mere image acquisition. They enable quantitative analysis of biological data, which significantly enhances research methodologies. By applying sophisticated algorithms, researchers can extract meaningful information from images, allowing for metrics such as cell viability, structural integrity, and even molecular interactions.
βDigital imaging transforms not just how we see samples but also how we analyze them.β
Furthermore, digital imaging accommodates different imaging modalities, like fluorescence and phase contrast, which are critical in biological studies. These modalities allow researchers to label specific cellular components, making specific biological interactions more visible.
Challenges in digital imaging do exist. High-resolution imaging can lead to large file sizes, complicating storage and data management. Additionally, specialized training may be necessary to operate sophisticated digital imaging equipment effectively.
Digital Imaging in Microscopy
Digital imaging has become a foundational aspect of modern microscopy. It combines optics and computer technology to capture images of specimens in a more refined manner than standard techniques. The transition to digital has improved microscope functionality and efficiency, making it suitable for a range of biological applications.
The digital format allows for easy integration with various microscope types, including fluorescence, confocal, and electron microscopes. Researchers can apply this technology to capture data at multiple magnifications and resolutions, ensuring that intricate structures remain visible.
One notable advantage is the real-time imaging capability. Researchers can monitor live specimens under controlled conditions. This functionality aids in studying processes such as cell division, migration, and responses to stimuli in actual biological time.
Additionally, digital imaging facilitates high-throughput analysis, which is essential in genomics and proteomics. Automated imaging systems can rapidly capture images across multiple fields of view, enabling larger sample sizes in research studies.
Software Innovations for Image Analysis
Alongside imaging techniques, software innovations for image analysis have profoundly changed how research is conducted in biology. These applications improve the capability to interpret complex data derived from imagery.
A plethora of software programs are available for image analysis. These tools often use advanced mathematical algorithms to quantify images accurately. For example, ImageJ is widely used in biological research to perform operations such as counting cells, measuring size, and analyzing fluorescence intensity. This kind of quantitative analysis is paramount for conducting reproducible research.
Furthermore, machine learning and artificial intelligence are now entering the realm of image analysis. These technologies can identify patterns that human analysts might overlook. For example, neural networks can classify cell types based on microscopic images, significantly speeding up data interpretation.
Challenges arise with the adoption of software innovations. Researchers need adequate training to utilize these tools effectively. Additionally, the variability of software and hardware capabilities can lead to inconsistencies in data analysis across different laboratories.
To summarize, ongoing advancements in software for image analysis are propelling biological research forward. They enhance the ability to extract valuable insights from digital images, which ultimately supports the objectives of microscopy in biological studies.
Future Directions in Microscopy
Advancements in microscopy showcase an unending journey of discovery and innovation. Understanding these future directions is vital for biologists and researchers. The impact of emerging technologies holds the potential to reshape the way we observe and analyze biological systems. Improvements in resolution, speed, and accessibility will significantly enhance research capabilities.
Emerging Technologies
Emerging technologies in microscopy promise to refine and expand biological research. Notable advancements include super-resolution imaging techniques. These techniques surpass the optical diffraction limit. They allow for visualization of structures at the nanometer scale. Examples include STORM (Stochastic Optical Reconstruction Microscopy) and PALM (Photo-Activated Localization Microscopy). These methods are transforming our understanding of cellular processes.
Moreover, advancements in light-sheet microscopy are noteworthy. This technique illuminates samples with a thin sheet of light. This minimizes photo-damage and enables faster imaging. It is particularly useful for studying live organisms over extended periods.
Another trend involves integrating artificial intelligence with imaging. AI-assisted image analysis can drastically speed up data interpretation. Researchers can focus on complex biological questions instead of getting lost in data volume.
- Increased Resolution: New lenses and sensors reduce noise and improve clarity.
- Real-time imaging: Technologies allow for monitoring live cellular processes dynamically.
- 3D reconstruction: Advances enable the 3D reconstruction of tissues and organs from 2D images.
Integration with Other Scientific Fields
The integration of microscopy with other scientific disciplines offers significant benefits. For instance, pairing microscopy with genomics leads to insightful discoveries. Techniques like CRISPR are used alongside microscopy to visualize gene editing effects directly.
Moreover, collaboration between microscopy and bioinformatics is on the rise. This partnership enhances the capacity to handle large sets of image data. Biologists can employ machine learning to find patterns and generate hypotheses faster.
Fields such as material science also gain from microscopy advancements. Understanding biological materials at the microscopic level can lead to improved biomaterials for medical applications.
Researchers should consider interdisciplinary approaches in their work. By merging microscopy with disciplines such as chemistry, physics, and computer science, they can unlock new potentials.
"Merging technology with traditional microscopy will redefine biological research. The future lies in collaboration and innovation."