Microfluidic Manufacturing: Applications and Advances
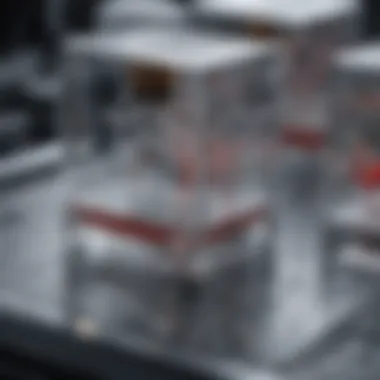
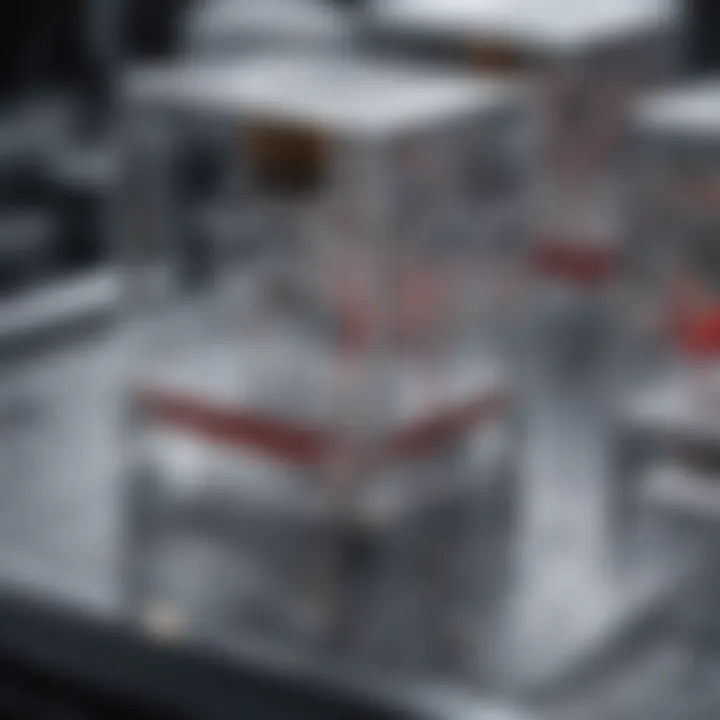
Intro
Microfluidics has emerged as a game-changer in various fields such as biotechnology, pharmaceuticals, and even environmental monitoring. The ability to manipulate tiny fluid volumes has significant implications for research and industry. This article serves as an in-depth guide to understanding microfluidic manufacturing, touching on the unique technologies utilized, applications across different domains, and the challenges that the industry grapples with.
With a focus on how these miniaturized systems are designed and produced, we’ll shine light on the innovative processes that set microfluidics apart from traditional manufacturing. From lab-on-a-chip devices used for rapid diagnostics to complex systems enabling precise drug delivery, the potential is vast and largely untapped.
As we embark on this exploration, consider it as a lens through which we can observe how microfluidics is poised to shape the landscape of modern science and technology.
Research Overview
The realm of microfluidics is deep and multifaceted. A comprehensive understanding necessitates looking at the research that has paved the way for advancements in the field. This exploration delves into the nuances, providing a summary and objectives that will guide the discourse.
Summary of Key Findings
Research highlights not only the technological innovations involved but also the interdisciplinary nature of microfluidics which blends engineering, physics, and biology. Here are some key findings:
- Versatility: Microfluidic devices can be tailored for various applications, from medical diagnostics to environmental testing.
- Cost-efficiency: Manufacturing processes are often more economical than traditional methods.
- Precision: Control over micro-scale fluid flow allows for highly accurate experiments and product development.
- Scalability: Microfluidic technologies can be easily scaled, making them adaptable for both small labs and large production facilities.
Research Objectives and Hypotheses
The article seeks to answer several fundamental questions within the microfluidics domain.
- What are the current limitations faced by manufacturers?
- How do innovations in technology affect product design and application?
- In what ways can microfluidic devices be integrated into existing healthcare solutions?
Each hypothesis will guide the examination of methodologies and applications in subsequent sections of the article.
Methodology
Understanding how research in microfluidics is conducted will provide a solid foundation for our later discussions. The methodologies employed offer insight into how findings are derived and validated.
Study Design and Approach
A mixed-methods approach is often favored in this field, combining quantitative analyses with qualitative insights. This hybrid model allows for a comprehensive assessment of both experimental data and user experience.
Data Collection Techniques
Data is collected through various means including experiments, simulations, and user feedback.
- Experimental Tests: Controlled studies to evaluate the performance of microfluidic devices under different conditions.
- Surveys: Gathering input from users in research and industry, highlighting real-world challenges and needs.
- Case Studies: Analyzing specific applications to illustrate successful implementations and outcomes.
In the sections to follow, we will dive deeper into the technologies driving microfluidic manufacturing, applications that are revolutionizing processes, and the unavoidable obstacles that practitioners encounter in this evolving field.
Defining Microfluidics
Microfluidics is a domain at the intersection of engineering, physics, and biology, dealing with the manipulation of fluids at a microscopic scale. This area has gained immense traction in recent years, linking to advancements in diverse fields such as healthcare, environmental science, and chemical processes. By examining the intricacies of microfluidics, one can appreciate how this technology underpins many of today’s innovative solutions.
Understanding microfluidics involves delving into its fundamental principles and historical developments. These elements explain why microfluidics is not merely a niche concern but a vital component that shapes various technological advancements. The careful control of small fluid volumes—often in the nanoliter to picoliter range—affords significant benefits, including reduced reagent usage, faster reaction times, and the ability to conduct complex analyses in real time.
Consider the convenience of a lab-on-a-chip device; it’s designed to perform multiple analytical functions concurrently. This ability revolutionizes diagnostics, enabling timely decisions in clinical settings. Furthermore, microfluidic systems can integrate with other technologies, enhancing functionality and user accessibility. As we will explore, the principles of microfluidics are foundational to its applications, influencing everything from drug delivery systems to environmental monitoring.
Principles of Microfluidics
At its heart, microfluidics relies on three main principles: capillary action, laminar flow, and surface tension. Each plays a pivotal role in controlling how fluids move and mix at tiny scales.
- Capillary Action: This is the ability of a liquid to flow in narrow spaces without the assistance of external forces. In microfluidic devices, this property is exploited to drive the movement of fluids through channels. A small drop of liquid can traverse a microchannel, providing precise control over the volume and velocity of solutions.
- Laminar Flow: In microfluidic systems, the flow of liquids is typically laminar, meaning that the fluid moves smoothly in parallel layers, without turbulence. This minimizes dispersion and enhances the precision of fluid handling, allowing for accurate mixing and reaction processes.
- Surface Tension: A significant factor, surface tension affects how droplets form and how they interact within microfluidic environments. This principle enables the formation of segments or bubbles, essential for processes like droplet-based microfluidics.
Recognizing these principles allows researchers and professionals to leverage microfluidics for various innovative applications such as point-of-care diagnostics and targeted drug delivery systems. This understanding drives ongoing research in material science and engineering, aiming to refine processes and broaden the capabilities of microfluidic devices.
Historical Context
The journey of microfluidics is both captivating and complex, tracing back to inventions that laid the groundwork for today's devices. Early developments, such as the use of capillary action in inkjet printer technology in the 1980s, began to showcase the potential of controlled fluid handling at small scales.
However, it wasn't until the 1990s that the term "microfluidics" solidified its place in scientific literature. During this era, significant strides were made, particularly in the design of microfabrication techniques. Researchers began experimenting with photolithography and soft lithography, paving the way for the creation of more sophisticated microfluidic structures.
Noteworthy achievements include the development of the first microfluidic chip designed for biological analysis. It was based on existing technologies yet introduced an elegant solution to practical challenges in diagnostics and chemical analysis. The shift to biomolecular applications, particularly in genomics and proteomics, marked a critical expansion in the relevance of microfluidics.
In the subsequent decades, technological advancements have propelled the industry forward, marrying traditional chemistry with modern computing feedback systems. The march of innovation continues as research institutions and companies explore applications that range from personalized medicine to rapid environmental assessments.
As we consider the legacy and progress of microfluidics, it’s apparent that this field represents the convergence of multiple disciplines, offering insights into both scientific questioning and practical application. The compelling history of microfluidics invites us to ponder its future, where novel developments promise to redefine what’s possible in fluid manipulation.
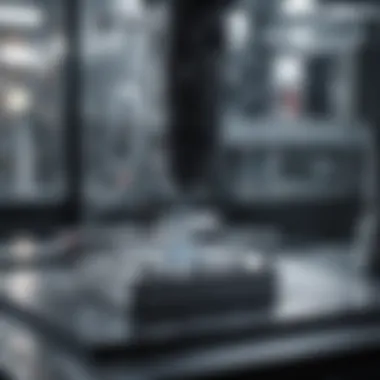
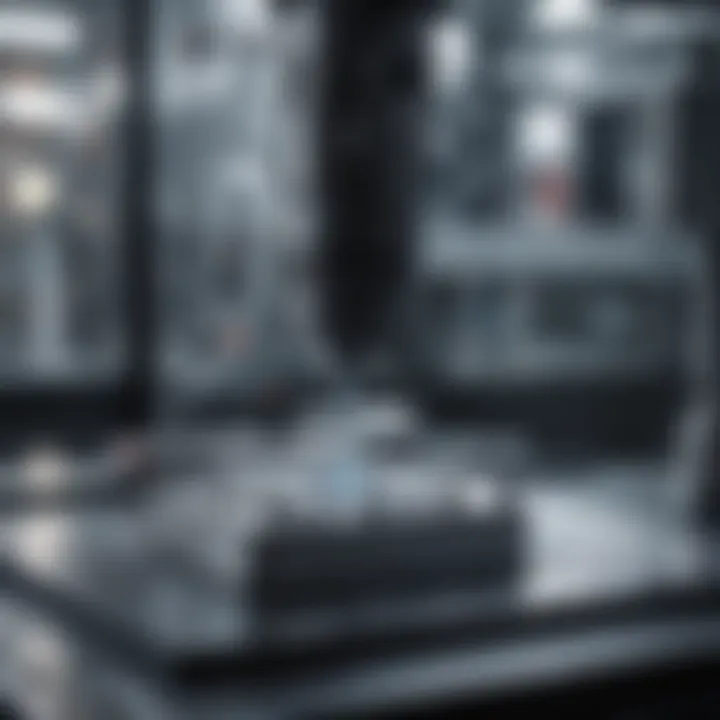
The Microfluidics Industry Landscape
The microfluidics sector has burgeoned into a significant area of focus in both academic and industrial realms. Understanding this landscape is essential for those involved in research or the development of microfluidic technologies. A multitude of stakeholders—including researchers, manufacturers, and investors—are engaging with this field due to its wide-ranging applications, substantial economic potential, and its ability to address pressing global challenges.
Central to this industry landscape are two key sections: market analysis and the identification of key players. Each of these elements not only contributes to the growth of microfluidics but also helps illuminate the trends and opportunities that lie ahead.
Market Analysis
The market for microfluidic devices has undergone a notable transformation in recent years. Its growth trajectory can be attributed to several critical factors:
- Rising Demand for Point of Care Testing: The need for rapid diagnosis and monitoring, especially in healthcare, has driven the necessity for efficient, portable microfluidic devices.
- Technological Advancements: Innovations in materials and production techniques, such as soft lithography and 3D printing, have greatly enhanced microfluidics, making them more accessible and versatile.
- Expanding Applications Across Various Fields: Beyond biomedical uses, microfluidics is diversifying into areas such as chemical analysis and environmental monitoring, broadening its market appeal.
According to various industry reports, the market is projected to witness a compound annual growth rate (CAGR) of over 20% in the coming years. This growth indicates not just the expansion of existing technologies but also the emergence of novel applications. For instance, approaches in personalized medicine are redefining how treatments are tailored to patients, opening avenues for collaboration across biotechnology, software, and hardware sectors.
Key Players
The microfluidics industry is populated by a variety of players, ranging from established companies to promising startups, each contributing uniquely to the ecosystem:
- Thermo Fisher Scientific: Known for their advanced laboratory equipment, they lead in providing microfluidic platforms for applications in genomics and proteomics.
- Illumina: Dominating the genetic testing space, Illumina utilizes microfluidic chips to streamline processes in DNA sequencing and analysis.
- Fluidigm: A frontrunner in high-throughput and integrated microfluidics solutions, they have carved out a niche in single-cell analysis and genomics.
- Startups: Numerous new firms are emerging, offering innovations that challenge the status quo. Companies like Cellix are revolutionizing the way we view cell interactions with their microfluidic chips, presenting potential for unprecedented insights in drug development.
Understanding the roles of these key players provides insight into where resources and innovations are being concentrated. It also highlights the competitive nature of the industry, with each participant striving to push the boundaries of what microfluidics can achieve.
"The microfluidics market thrives on innovation and collaboration, shaping a future where complex biological and chemical processes can be tackled with unprecedented precision."
In summary, the microfluidic industry landscape is not only about quantifying economic growth but also about recognizing the intricate web of interactions between technology, application, and commercialization. This intricate interplay will continue to propel forward the field of microfluidics, making it a pivotal sector for research and development.
Microfluidic Device Fabrication Techniques
Microfluidic device fabrication techniques hold paramount significance in the realm of microfluidics. These methods are the backbone of producing devices that manipulate fluids at a micro scale, resulting in a broad array of applications from diagnostics to environmental monitoring. Understanding these techniques not only sheds light on how these devices are constructed but also how innovations in fabrication lead to advancements in microfluidic applications. As the desire for more efficient and versatile devices grows, mastering these fabrication methods is crucial for students, researchers, and professionals alike.
Soft Lithography
Soft lithography stands out as a prominent fabrication technique in the microfluidics world. This method, utilizing elastomeric polymers, excels in creating intricate, micro-scale features with high fidelity. The principal material typically used is polydimethylsiloxane (PDMS). PDMS is both flexible and biocompatible, making it an excellent choice for biological applications.
One of the major advantages of soft lithography is its relative simplicity and low cost compared to traditional photolithographic techniques, which often require expensive materials and equipment. The process involves creating a master mold using conventional photolithography, which is then used to cast the PDMS. The final product can easily integrate with various compatible materials, enabling the development of complex microfluidic networks. This versatility is especially pertinent in biomedical applications, where devices must interact with a variety of biomolecules.
Moreover, soft lithography enables
- rapid prototyping,
- a feature valuable in fast-paced research settings.
- Its ability to produce re-usable molds allows manufacturers to streamline their workflows as well.
In sum, soft lithography serves as a stepping stone for many in the microfluidics field, simplifying the journey from concept to prototype.
Injection Molding
Injection molding is another significant technique for the mass production of microfluidic devices. This process involves melting polymer granules and injecting them into a precise mold under high pressure. This technique is well-suited for producing large quantities of uniform microfluidic devices with high throughput.
Key benefits of injection molding include:
- Scalability: Once a mold is fabricated, thousands of identical devices can be produced efficiently, making it ideal for commercial applications.
- Cost-Effectiveness: While initial costs for mold creation can be high, the per-unit cost decreases substantially with production volume.
- Material Variety: A broader range of thermoplastics can be used, allowing for tailored mechanical and chemical properties.
Despite its advantages, injection molding does come with certain considerations. The complexity of the channel designs can pose challenges. Highly intricate designs may require advanced techniques or multiple molds, which can increase production costs and time. Nonetheless, for industries looking to produce microfluidic devices on a large scale, injection molding represents a viable and often preferred option.
3D Printing in Microfluidics
3D printing has emerged as a game-changer in the microfluidic manufacturing landscape. This technology allows for the layer-by-layer fabrication of complex structures without the need for molds, offering unmatched flexibility. Researchers can easily tweak designs, creating custom devices tailored to specific applications or experiments.
Some benefits of 3D printing include:
- Rapid Prototyping: The turnaround time from design to prototype can be remarkably short, fostering innovation.
- Customization: Users can produce devices with unique features, such as varying channel dimensions or integrated sensors, which are difficult to achieve using traditional methods.
- Integration: It’s possible to incorporate various materials into a single device, enhancing functionality.
However, there are hurdles to overcome. The resolution of 3D printing can be a limiting factor; achieving the fine features required for effective microfluidics often requires high precision. Additionally, the mechanical properties of printed materials may not always mimic those of commercially available materials, which can affect device performance.
As development continues, 3D printing holds exciting potential for future applications, providing a pathway to more innovative microfluidic devices.
In summary, methods like soft lithography, injection molding, and 3D printing shape the landscape of microfluidic manufacturing. Each technique presents distinct advantages and challenges, influencing the development process and the application of microfluidic devices in numerous fields.
Materials Used in Microfluidic Manufacturing
Microfluidics is a rapidly evolving field, and the materials used in its manufacturing are pivotal in shaping the effectiveness and functionality of microfluidic devices. The choice of materials influences not only the performance but also the cost and scalability of microfluidic systems. Understanding the properties and applications of these materials provides insight into how they serve the specific needs of various applications, from biomedical devices to environmental sensing. Thus, having a solid grasp on which materials are best suited for microfluidics can lead to innovative solutions and advancements in this niche area.
Polymeric Materials
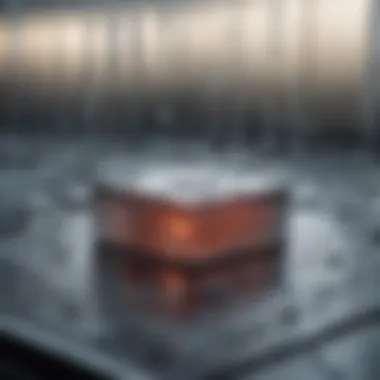
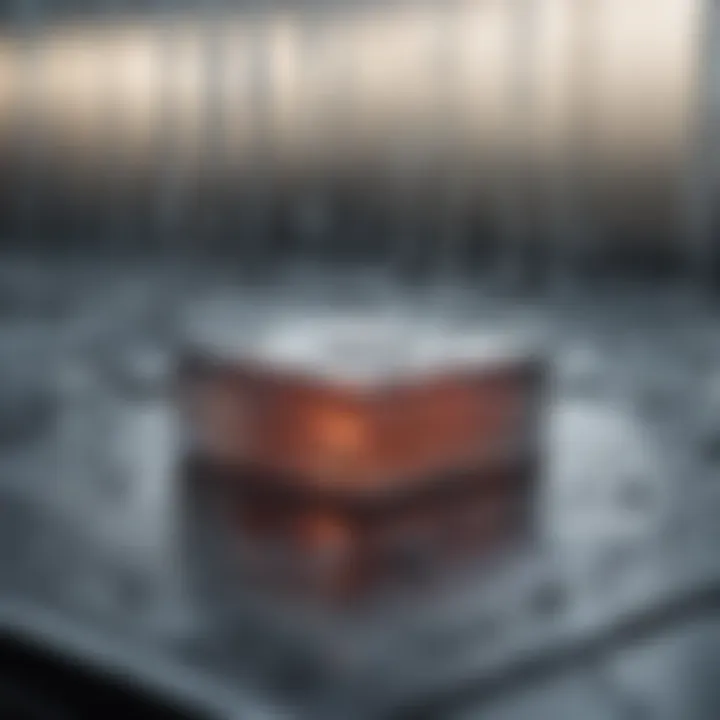
Polymeric materials are often preferred in microfluidic manufacturing due to their versatility and ease of processing. These materials include low-cost options such as polydimethylsiloxane (PDMS), poly(methyl methacrylate) (PMMA), and polycarbonate. PDMS is particularly notable for its excellent optical transparency and biocompatibility, making it a preferred choice in biological applications. The ability to mold PDMS into complex geometries is a game changer in fabricating microfluidic channels, imitating the natural environments of various biological processes.
Advantages of using polymeric materials include:
- Flexibility: They can be easily shaped, allowing for intricate designs that are crucial in fluid manipulation.
- Cost-effective: Generally, the costs associated with polymers are lower compared to silicon or glass.
- Biocompatibility: Many polymers are compatible with biological samples, which is essential for applications in medical diagnostics and drug delivery.
However, there are also considerations when using polymeric materials. For instance, their susceptibility to absorption of certain chemicals can be a downfall for specific applications. Additionally, long-term stability might be questioned in some environments, especially where exposure to harsh chemicals is prevalent.
Silicon and Glass
Silicon and glass represent the traditional materials used in microfluidic device manufacturing. Their importance cannot be overstated, especially in contexts where precision and robustness are of utmost concern. Silicon, often used in combination with etching techniques, allows for precise control over channel dimensions at the microscale.
Glass is hailed for its superior optical properties and chemical inertness, ideal for applications requiring high-resolution imaging or containing aggressive reagents. Devices made of glass are often more stable under extreme conditions, giving them longer lifespans compared to their polymeric counterparts.
Benefits of using silicon and glass include:
- Durability: These materials can withstand higher temperatures and chemical exposures.
- Exceptional surface properties: Silicon can be modified to enhance surface chemistry, facilitating various applications, including cell culture and chemical reactions.
- Integration possibilities: Silicon can readily integrate with electronic components, making it ideal for lab-on-a-chip devices.
Despite their advantages, the use of silicon and glass can entail higher manufacturing costs and more complex fabrication processes. The brittleness of glass, for example, could pose problems during assembly and usage, demanding careful handling.
"Choosing the right material is crucial in the realm of microfluidics. Each material has its own strengths and weaknesses that can affect the entire performance of the device."
Applications of Microfluidics
The realm of microfluidics has expanded remarkably, becoming a cornerstone in various industries. Applications of microfluidics are not just a cherry on the cake; they form the very bread and butter of modern scientific endeavors. By manipulating fluids at a microscopic scale, researchers and engineers have unlocked potentials that were previously inconceivable. The essence of this section is to explore the multifaceted applications of microfluidics, highlighting their importance in advancing technology and improving lives.
Biomedical Applications
Diagnostics
Diagnostics in the field of microfluidics is akin to having a Swiss Army knife for healthcare professionals. The capability to analyze biological samples rapidly provides valuable insights into diseases, potentially saving lives. When we talk about diagnostics, we focus on its unparalleled accuracy and speed. Traditional methods require larger sample sizes and more time, making them cumbersome in urgent situations. Microfluidic diagnostic tools, on the other hand, use tiny droplets and channels to deliver quick results, presenting an excellent choice for point-of-care testing.
A unique feature of microfluidic diagnostics lies in its integration with lab-on-a-chip technologies. These devices can perform multiple tests simultaneously, making them not just efficient but also cost-effective. However, the technology is not without its challenges; issues related to reproducibility and yield can occasionally throw a spanner in the works.
Drug Delivery
The landscape of drug delivery is experiencing a renaissance thanks to microfluidics. This technology allows for targeted release mechanisms, which enhance the bioavailability of medications and reduce side effects. The key characteristic of microfluidic drug delivery systems is their ability to fine-tune dosages and control drug release profiles. This targeted approach is particularly beneficial in the treatment of chronic diseases, where precision is crucial.
One standout feature is the capability of these systems to mimic biological conditions for better absorption of drugs. While the advantages are many—like improved patient compliance and minimized systemic exposure—there are drawbacks too. The complexity of designing such systems means that it may require more time and investment than traditional approaches. Still, the positive impact of microfluidic drug delivery on patient care is undeniable.
Environmental Monitoring
The application of microfluidics extends beyond healthcare into environmental monitoring, where real-time assessments of pollutants are crucial. These small-scale systems can sense and analyze environmental parameters, such as toxins in water or air quality, with exceptional sensitivity. Their portability allows for on-site testing, which is invaluable when immediate results are necessary for public safety.
Incorporating microfluidics in this realm not only enhances the accuracy of measurements but also democratizes access to vital information, empowering communities to make informed decisions about their environment.
Chemical Analysis
When it comes to chemical analysis, microfluidics is leading the charge in miniaturizing processes that were once only feasible in large laboratories. This technology can analyze chemical reactions, monitor reaction conditions in real-time, and even control experimental conditions with remarkable precision. The distinct advantage of using microfluidics for chemical analysis lies in its ability to reduce reagent consumption and minimize waste, aligning with current sustainability initiatives.
However, the intricate design and fabrication of microfluidic systems can complicate the analytical processes, thus demanding interdisciplinary knowledge from chemists and engineers alike. Still, the opportunities for efficiency in chemical experimentation make microfluidics a valuable tool for researchers in the field.
Microfluidics consistently blurs the lines between various domains, showcasing its versatility and potential across multiple applications.
Challenges in Microfluidic Manufacturing
Microfluidic manufacturing has made significant strides over the years, contributing to various advancements in healthcare, environmental monitoring, and chemical analysis. However, despite its promise, there are a number of challenges that this field faces. Understanding these challenges is crucial for uncovering methods to overcome obstacles and push the boundaries of what microfluidics can achieve. It’s important to delve into the complexities of this topic, as it has substantial implications on future developments, innovations, and overall market potential.
Technical Limitations
Microfluidic devices are heralded for their precision and efficiency but are not without their limitations. One of the primary technical challenges is the scaling of manufacturing processes. While creating small devices in a lab setting can yield impressive results, replicating these processes at a larger scale poses difficulties. Not all techniques work equally well beyond the small-scale environment. Additionally, the fabrication methods, like soft lithography and injection molding, can sometimes compromise on accuracy when mass-producing microfluidic components.
Another area of concern is the material compatibility within microfluidic systems. Various materials behave differently when in contact, and it's quite common to observe problems such as leakage or limited durability over time. For instance, polymeric materials may yield excellent results in terms of flexibility and low cost but can struggle under varied chemical environments or high temperatures. This creates a revolving door of challenges regarding how to optimally combine materials without compromising device performance.
"Advancements in microfluidic technology must address these technical limitations through innovative materials and improved fabrication processes to truly advance the field."
This quest for innovation also entails a significant investment of time and resources. R&D efforts in this area often require specialized skill sets, which can elevate costs. Thus, manufacturers must balance the need for cutting-edge technologies with economic feasibility.
Regulatory Hurdles
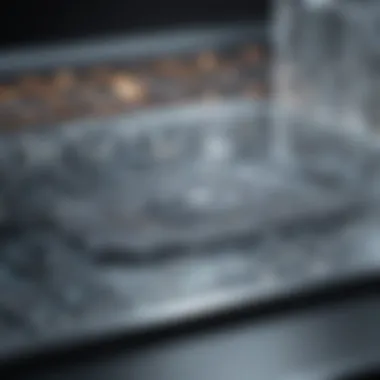
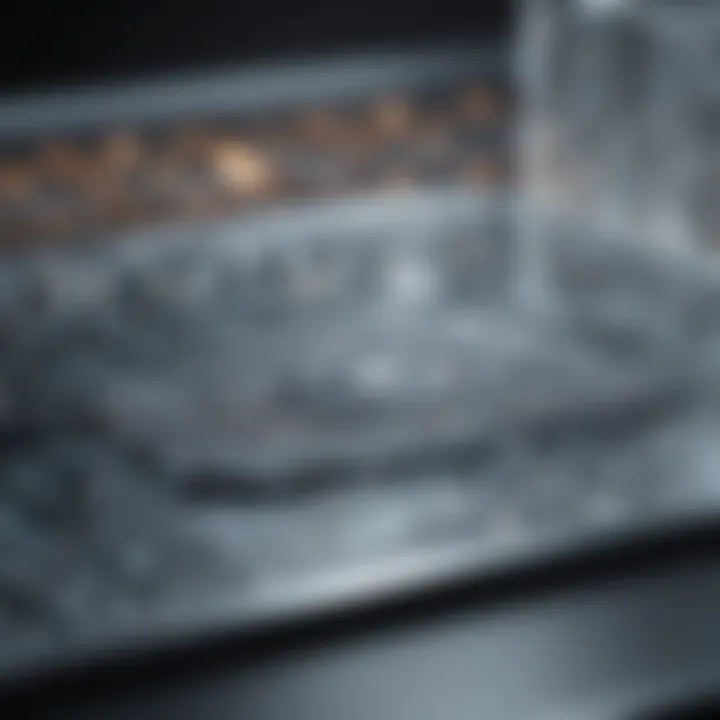
Another key challenge in the microfluidics landscape involves regulatory hurdles. As devices drift closer to clinical applications, they must comply with stringent regulatory standards. The path to regulatory approval can be labyrinthine, often varying significantly between regions like Europe and North America.
Understanding the 'dos and don'ts' of regulatory compliance is critical for manufacturers looking to scale their innovations. For example, the extensive quality assurance processes mandated in the U.S. Food and Drug Administration (FDA) regulations can be daunting. These lengthy processes require comprehensive validation and may involve multiple rounds of testing and documentation. For small companies or startups, this can become a potential roadblock, consuming resources and extending time-to-market.
Moreover, staying up-to-date with changing regulations can be a challenge itself. What may be compliant today might not hold true tomorrow. This necessitates continual adjustments in manufacturing practices and quality assurance protocols. As a result, adaptability becomes a key trait for manufacturers operating in the microfluidics space.
It's evident that without navigating these regulatory challenges successfully, the promising applications of microfluidics in diagnostics, drug delivery, and other critical areas may find themselves stymied at an early stage.
Innovations in Microfluidics
Microfluidics is rapidly evolving, with constant innovations reshaping the landscape. This section emphasize the significance of advanced practices, highlighting how they not only enhance existing products but also usher in entirely new functionalities and applications. With ongoing advancements, the potential of microfluidic systems continues to grow, impacting areas such as biomedical engineering, environmental monitoring, and many more.
Emerging Technologies
Emerging technologies in microfluidics are like a breath of fresh air. These innovations are pivotal for pushing boundaries, leading to breakthroughs that were once deemed far-fetched. Technologies like lab-on-a-chip systems, which integrate multiple analysis functions into a single device, exemplify the transformative prospects that lie in this field.
- Lab-on-a-chip: This miniaturization enables faster results, lower costs, and reduced sample volumes. It allows for complex analyses, previously requiring large lab setups, to now be accomplished on a handheld device.
- Organ-on-a-chip: This technology replicates the physiological environment in vitro, providing insights into human biology and disease without the need for animal models.
- Digital Microfluidics: Here, droplets are manipulated on an array of electrodes, giving researchers control over minute volumes. This versatility is invaluable for precise dosing in drug testing and other applications.
These innovations are vital. They not only enable new applications, but also promise more efficient processes, a boon for industries that depend on rapid and accurate data.
Integration with Other Technologies
Integrating microfluidics with other technologies is where the magic truly happens. Such combinations allow the best of both worlds, fostering innovations that can address complex challenges. Consider the synergy with:
- Artificial Intelligence (AI):
The use of AI algorithms to analyze data derived from microfluidic processes is already transforming research methodologies. Machine learning can enhance the interpretation of results, leading to quicker and more accurate predictions. - Wearable Technologies:
The fusion of microfluidics with wearables has opened doors for real-time health monitoring. Devices equipped with microfluidic sensors can analyze sweat or interstitial fluid, enabling personalized healthcare tailored to individual needs. - Biotechnology: Incorporating microfluidics into biotechnological applications can streamline various processes, from gene editing to bioproduction, making these processes more efficient and cost-effective.
As these technologies continue to converge, the ability to innovate within microfluidics expands exponentially. The future lies in such integrations that can foster new products while enhancing the functionality of existing ones.
"Microfluidic innovations hold the key to unlocking new paradigms in diagnostics, therapeutics, and beyond, reflecting our unending quest for precision and efficiency in scientific inquiry."
Future Trends in Microfluidic Manufacturing
The evolution of microfluidic manufacturing is ever-present, often mirroring technological advancements in related fields. Focusing on future trends not only unearths the potential changes in microfluidic design and application but also highlights the broader implications on healthcare, environmental science, and beyond. Analyzing this landscape equips researchers and industry stakeholders with the insights needed to remain at the forefront of innovation.
Sustainability Initiatives
In recent years, environmental concerns have gained significant traction in multiple industries. Microfluidics is no exception. The push for sustainability initiatives is increasingly shaping manufacturing processes. This includes the use of eco-friendly materials and energy-efficient production methods. For instance, using biodegradable polymers can enhance the sustainability profile of microfluidic devices, reducing long-term waste.
Furthermore, companies are exploring the development of microfluidic chips that utilize fewer resources in their production. This not only leads to cost savings but also minimizes the carbon footprint associated with manufacturing.
- Alternative Materials: Exploring materials that are both efficient and sustainable can open new avenues for research.
- Green Production Techniques: Techniques like recycling and repurposing existing materials are becoming critical in the manufacturing checklist.
"In the face of climate change and resource depletion, innovative pathways toward sustainable microfluidic technologies will not only comply with regulations but will also attract environmentally conscious consumers."
Personalized Medicine
The realm of personalized medicine is burgeoning, and microfluidics is a vital cog in this wheel. The ability to analyze biological samples at the microscale fosters tailored therapeutic strategies. Utilizing microfluidic devices, healthcare professionals can obtain rapid and precise diagnostic information, paving the way for customized treatment plans for patients.
Such devices can handle samples with remarkable precision, leading to highly accurate assessments. This not only enhances patient care but also contributes to better clinical outcomes. The trend toward miniaturization aligns seamlessly with the demand for precise diagnostics.
Incorporating machine learning and AI into microfluidic devices permits real-time data analysis, which can further refine personalization. As these technologies converge, the impact on healthcare will likely grow significantly.
- Case Studies in R&D: Companies are innovating by integrating AI algorithms to optimize the performance of microfluidic devices.
- Future Directions: The shifts towards patient-centered care dictate that research in microfluidics must prioritize scalability and adaptability to accommodate diverse medical needs.
As we glance into the future, it becomes evident that the crossroads of sustainability and personalized medicine are shaping the path forward in microfluidic manufacturing.
Finale
Reaching the conclusion of our exploration into microfluidic manufacturing reveals key insights that are pivotal for understanding its significance in the contemporary landscape of technology and research. Microfluidics is not merely a trend; it's a transformative approach that holds impressive potential across various domains—from biomedical research to environmental monitoring—addressing critical needs in society.
Summary of Key Insights
We have ventured through several notable points regarding microfluidics:
- Diversified Applications: The spectrum of applications, such as diagnostics and drug delivery, showcases how microfluidics streamlines processes ineffibly.
- Innovative Techniques: The examination of fabrication methods includes soft lithography and 3D printing, both of which present unique advantages and challenges.
- Industry Dynamics: Understanding the market and key players helps outline the competitive landscape, enhancing our grasp on how innovations emerge within the industry.
- Sustainability Considerations: The push towards sustainable manufacturing practices marks an essential direction that aligns with global imperatives on environmental health.
These insights emphasize the complexity and multifaceted nature of microfluidics as a discipline, sparking interest among students, researchers, and professionals alike.
Call for Continued Research
As we reflect on the rapid advances in microfluidic technology, it's crucial to acknowledge the ongoing need for research. The landscape is evolving quickly, and several areas demand further exploration:
- Standardization Processes: Developing benchmarks for microfluidic devices ensures reliability and acceptance in the market.
- Cross-disciplinary Collaborations: Engaging experts from diverse fields can lead to innovative solutions in tackling fundamental challenges.
- Longitudinal Studies on Efficacy: Exploring the long-term efficacy of microfluidic devices, especially in the biomedical field, can yield invaluable data for enhancing device design.
In closing, investing in continued research within microfluidics opens the door to not just technological advancements but also addresses global challenges in health, environmental sustainability, and more. The synergy between innovation and research lays the groundwork for a future where microfluidic manufacturing evolves in tandem with our growing demands.