Exploring the Insights and Applications of SEM Images
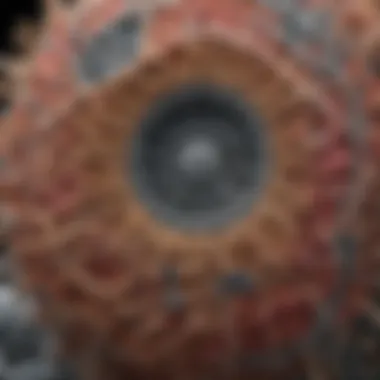
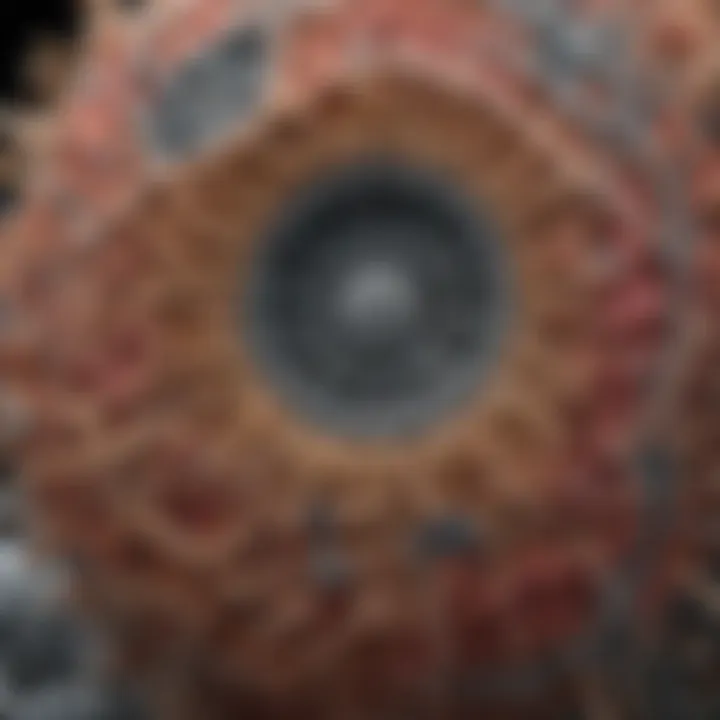
Intro
Scanning electron microscopy (SEM) has revolutionized the way we visualize materials at the nanoscale. Through advanced technologies, SEM pictures provide a detailed glimpse into the microstructure and topography of various substances. With increasing relevance in a broad range of scientific fields, understanding SEM images is crucial for researchers and professionals alike. Instead of just a means of imaging, scanning electron micrographs serve as essential tools for analysis and interpretation in disciplines such as biology and materials science. This article will explore the significance of SEM images, delving into their principles, technology, applications, challenges, and future advancements.
Research Overview
Summary of Key Findings
In examining SEM pictures and their applications, several key findings emerge:
- SEM provides high-resolution images that reveal surface details that are invisible to light microscopy.
- This imaging technique is indispensable in materials science for analyzing metals, polymers, and nanostructures.
- In biology, SEM plays a critical role in studying cellular structures and microorganisms, enhancing our understanding of biological processes.
- Though powerful, SEM does have inherent challenges, such as image distortion and the necessity of sample preparation.
Research Objectives and Hypotheses
This article aims to accomplish the following objectives:
- Clarify the principles of scanning electron microscopy.
- Investigate the technological advances in SEM that enhance image quality.
- Explore a variety of applications across diverse scientific fields.
- Identify the challenges inherent in SEM imaging and analysis procedures.
- Discuss ethical considerations in the use of SEM in research.
By addressing these objectives, we aim to equip the reader with a fundamental understanding of SEM pictures and their importance in contemporary research efforts.
Prelude to Scanning Electron Microscopy
Scanning electron microscopy (SEM) represents a critical tool in todayβs scientific landscape. Its ability to provide high-resolution, three-dimensional images of samples at the micro and nanoscale has reshaped fields ranging from material science to biology. Unlike conventional optical microscopy, SEM uses focused beams of electrons to illuminate specimens, resulting in enhanced detail and depth perception within the captured images. This introduction sets the stage for deeper discussions on the components, principles, and applications of SEM technology.
The importance of SEM cannot be overstated. As science progresses, the need for precision in imaging and analysis has intensified. The role of SEM in aiding researchers' understanding of complex structures is indispensable. With the ongoing advancements in imaging techniques, understanding SEM's historical context and modern significance becomes paramount.
Historical Background
The inception of scanning electron microscopy dates back to the 1930s. Initially, the objective was to address limitations present in light microscopy. Early pioneers, including Ernst Ruska, played a key role in the development of electron optics. The first practical SEM was developed by Manfred von Ardenne in 1938, which utilized a beam of electrons to provide images with much greater magnification than light microscopes. This marked a significant turning point in material analysis and biological studies.
As the technology evolved, modifications were made to improve both the resolution and usability of SEM. In the 1960s, the introduction of the first commercial SEM brought the technology to laboratories around the world. Over decades, refinements in electron source design and imaging detectors enhanced the capabilities and applications of SEM, leading to its acceptance as a standard tool in various scientific disciplines.
Significance in Modern Science
Today, SEM is invaluable across multiple realms, offering insights that were previously unattainable with prior imaging techniques. One of its core advantages is the ability to visualize the surface characteristics of a wide array of materials in minute detail. In material science, SEM assists in analyzing the microstructural features of metals, polymers, and ceramics. In biology, it allows for precise visualization of cellular structures and tissues, thus making it essential for histological studies.
Furthermore, SEM plays a pivotal role in nanotechnology, enabling researchers to observe and manipulate structures at the nanoscale. The precision that SEM provides is essential for advancements in drug delivery systems, electronics, and nanomaterials.
"The advent of scanning electron microscopy has revolutionized our understanding of the microscopic world, bridging gaps that once seemed insurmountable."
In addition, the versatility of SEM extends to its compatibility with various imaging modes, enhancing its functionality. The ongoing advancements in SEM technology suggest that its capacity to impact scientific research will continue to grow significantly. Understanding its historical evolution and modern applications allows for a more comprehensive perspective on SEM's integral role in advancing contemporary science.
Basic Principles of Scanning Electron Microscopy
Understanding the basic principles of scanning electron microscopy (SEM) is crucial for appreciating its role in modern science and research. SEM images provide detailed views that surpass traditional optical microscopes, giving insight into the structure and composition of materials at a micro and nanoscale. This section focuses on how SEM works, its components, and the image formation processes, elucidating the technology behind these remarkable imaging capabilities.
How SEM Works
The essence of SEM lies in its ability to scan a sample with a focused beam of electrons rather than light. When the electron beam strikes the surface of the specimen, interactions take place, leading to the emission of various signals, which include secondary and backscattered electrons, as well as characteristic X-rays. These emitted signals are collected and transformed into a high-resolution image.
One of the primary advantages of using electrons instead of light is that electrons possess shorter wavelengths, which allows for higher magnification and resolution. This aspect is particularly beneficial in observing fine structures that remain undetectable under other imaging techniques.
Components of a Scanning Electron Microscope
Every scanning electron microscope consists of several core components that work in harmony to produce high-resolution images:
- Electron Source: This generates the electron beam, often using a tungsten filament or a field emission gun.
- Lenses: Magnetic lenses are used to focus and direct the electron beam onto the sample, ensuring precision during scanning.
- Sample Chamber: Here, the specimen is placed. This area is usually under high vacuum to prevent electron scattering and to enhance image clarity.
- Detectors: These capture the signals emitted from the sample. Types of detectors used include secondary electron detectors and backscattered electron detectors, which help collect different types of information.
- Control Systems: These regulate the movement of the electron beam and the sample stage, allowing for detailed imaging of different parts of the specimen.
The collaboration of each component is essential for effective SEM operation.
Image Formation Process
The process of image formation in SEM is multifaceted. When the electron beam interacts with the surface of the sample, several outcomes occur, resulting in varied image signals:
- Secondary Electron Image Formation: Secondary electrons are generated when the primary electrons knock electrons off atoms in the sample. These low-energy electrons are crucial for depicting surface details.
- Backscattered Electron Imaging: This utilizes the electrons that bounce back from the sample. This type of imaging provides compositional contrast, enhancing understanding of the material properties.
- X-ray Imaging: In addition to electrons, X-rays produced during interactions provide elemental analysis of the specimen. This allows researchers to understand the material composition at a deeper level.
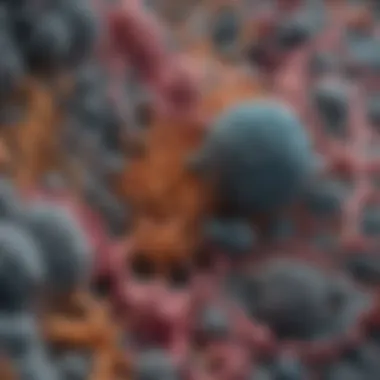
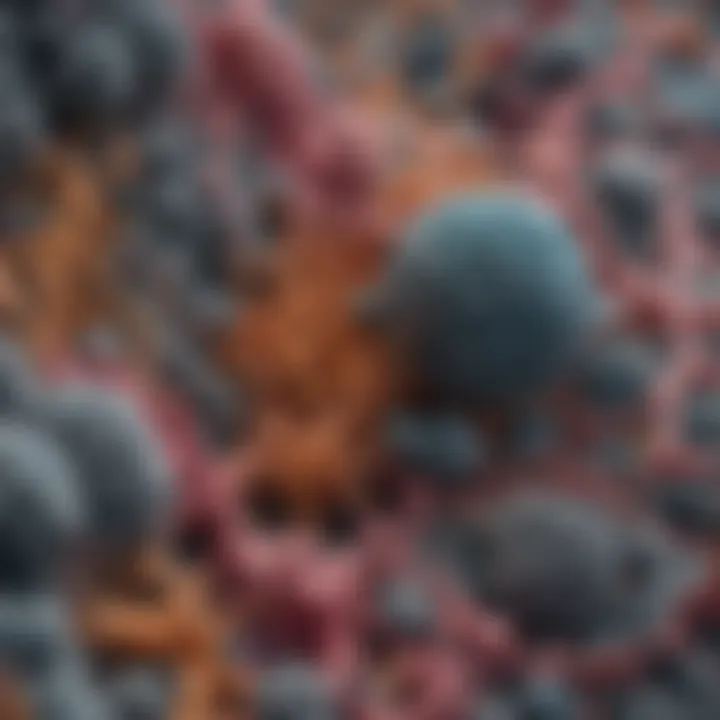
Important Note: The combination of these imaging modes enables a comprehensive analysis of samples, showcasing both surface morphology and material composition.
In summary, the basic principles of SEM are foundational for understanding its capabilities and applications. The intersection of electron microscopy and material science continues to evolve, opening new avenues for detailed examination in various fields. Recognizing these principles aids in appreciating the sophisticated technology employed in scanning electron microscopy.
Image Acquisition Techniques in SEM
Image acquisition techniques are central to the functionality of scanning electron microscopy (SEM). These methods determine how high-resolution images are generated from samples at the microscopic level. Understanding these techniques assists researchers in making informed decisions related to sample preparation and imaging strategies.
The importance of image acquisition techniques lies in their capability to provide clear and accurate representations of specimens. Effective image acquisition not only enhances the quality of data but also ensures that findings are reliable and reproducible. Moreover, optimizing these techniques can lead to discovering new insights into the structures being studied.
Sample Preparation Methods
Sample preparation is critical in SEM imaging. Properly prepared samples lead to more reliable and interpretable images. Techniques include coating non-conductive materials with a conductive layer to prevent charging during imaging. This is particularly relevant for biological samples and polymers which usually possess low electrical conductivity.
Effective sample prep can drastically improve electron signal and reduce artifacts.
Other methods involve fixing biological specimens, dehydrating them, and sometimes embedding them in resin for better mechanical stability. These approaches help preserve the cellular structure and morphology, enhancing image quality.
Imaging Modes
Imaging modes in SEM provide various ways to visualize samples, each serving distinct purposes. Two significant modes are Secondary Electron Imaging and Backscattered Electron Imaging.
Secondary Electron Imaging
Secondary Electron Imaging (SEI) is a prevalent imaging mode due to its high-resolution capabilities. SEI primarily records electrons that are ejected from the surface of the specimen upon electron beam impact. This results in detailed topographical images of the surface.
A notable characteristic of SEI is its sensitivity to surface features, making it an advantageous tool for imaging materials and biological samples alike. The unique aspect of SEI lies in its ability to reveal fine details such as surface roughness and morphology, which can be essential for materials science and biological research.
However, SEI does come with some disadvantages, such as being limited to imaging surfaces and potential sensitivity to contamination. Nonetheless, its benefits often outweigh these drawbacks, solidifying its role as a key method in SEM.
Backscattered Electron Imaging
Backscattered Electron Imaging (BSE) offers a different approach by detecting electrons that are backscattered from the sample. This modality is crucial for providing compositional contrast in a sample. A key characteristic of BSE is its ability to differentiate between materials of varying atomic weights, thus providing valuable insights into material composition without requiring chemical analysis.
The unique focus of BSE on providing compositional data makes it a beneficial choice for material science applications, particularly in failure analysis and surface characterization. However, BSE has some limitations, such as reduced surface detail compared to SEI, making it less suitable for studies demanding topographic information.
Resolution and Magnification
Resolution and magnification are essential aspects that dictate the quality of SEM images. Resolution refers to the smallest distance between two points that can still be distinguished as separate. Higher resolution allows researchers to observe finer details. SEM can achieve nanoscale resolution, which is crucial for applications in nanotechnology and semiconductor research.
Magnification allows users to observe objects at large scales. SEM can offer magnifications ranging from 10x to over 1,000,000x, facilitating significant flexibility in how specimens can be analyzed. Balancing resolution and magnification is vital for optimal imaging, ensuring relevant details are captured appropriately without loss of clarity.
In sum, mastering image acquisition techniques in SEM not only contributes to the clarity of images but also enriches the interpretations drawn from those images. Understanding how to effectively prepare samples, utilize imaging modes, and grasp the principles of resolution and magnification is essential for anyone looking to leverage the capabilities of SEM in their research.
Interpreting Scanning Electron Microscope Images
Interpreting scanning electron microscope images involves a critical examination of the visual data produced by SEM technology. This process is crucial for understanding material structures and behaviors at a microscopic level. A clear interpretation contributes significantly to drawing accurate conclusions in various scientific fields, including biology and materials science. Furthermore, a profound grasp of image characteristics can influence research outcomes and practical applications.
Understanding Image Quality
Image quality in scanning electron microscopy is fundamental to obtaining reliable results. High-quality images reveal details about surface structures, morphology, and other critical features. Several factors contribute to the overall image quality:
- Resolution: This is defined by the smallest distinguishable detail in an image. High-resolution images allow for more precise observations of tiny features, which is essential in fields that study nanoscale materials.
- Contrast: This refers to the difference in brightness between features in an image. Adequate contrast helps in distinguishing various components within a sample, enabling more effective analysis.
- Depth of Field: SEM images can have a greater depth of field compared to optical microscopes. This aspect is crucial when examining samples with three-dimensional topologies, providing a more comprehensive view of the structures.
Assessing these factors requires an informed perspective on what constitutes acceptable quality in SEM images. Researchers often employ calibration standards and image processing techniques to enhance image clarity and detail, further underpinning their analytical conclusions.
Common Artifacts in SEM Images
Artifacts in scanning electron microscope images can mislead interpretations and compromise data integrity. Recognizing these artifacts is essential for accurate image analysis. Common artifacts include:
- Charging Effects: Non-conductive samples may accumulate charge during imaging. This results in distortions, where areas of the image may appear brighter or darker.
- Motion Artifacts: Any movement of the sample or microscope during imaging can lead to blurring or distortion in the final image.
- Sample Preparation Artifacts: Inadequately prepared samples can introduce variations that do not reflect the true characteristics of the material. This includes damage from over-coating or insufficient surface cleaning.
Being aware of these artifacts aids researchers in accounting for potential discrepancies. By combining careful sample preparation with an understanding of the imaging system's limitations, one can reduce the impact of artifacts, leading to more reliable data interpretations.
"A thorough understanding of image quality and potential artifacts is key to leveraging SEM images for scientific advancement."
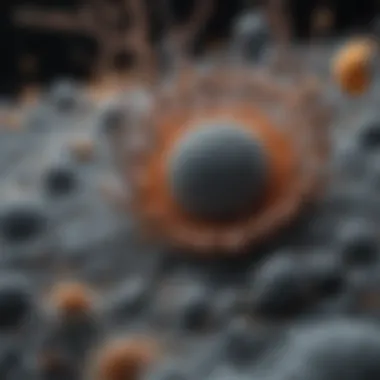
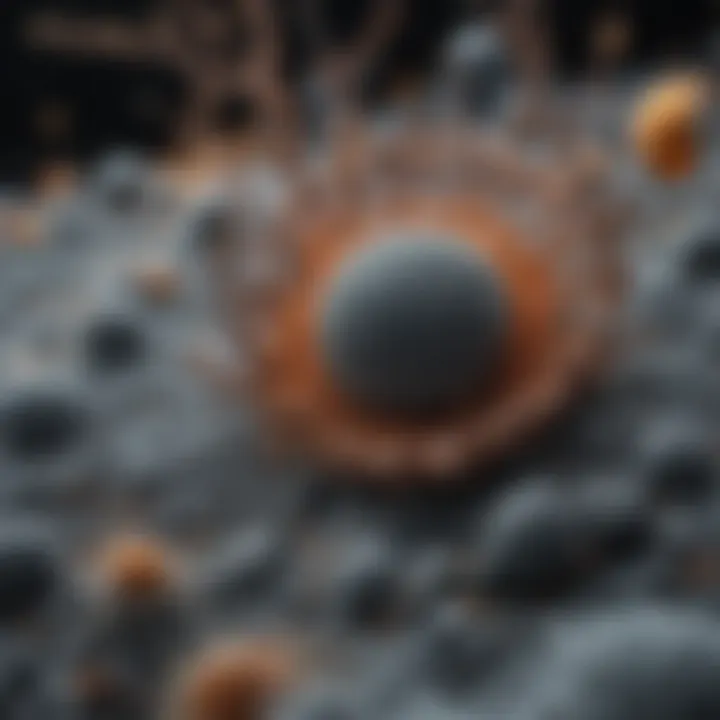
Applications of Scanning Electron Microscopy
The realm of scanning electron microscopy (SEM) spans a multitude of applications across various scientific fields. SEM enhances our understanding of material and biological structures through its high-resolution imaging capabilities. By utilizing electron beams, SEM provides detailed insights that conventional imaging technologies often cannot achieve. This section delves into the profound significance of SEM in diverse applications ranging from biology to materials science. It underscores the benefit of utilizing SEM for research and diagnostics, revealing its potential to inform future innovations.
Biological Applications
Cell Structure Analysis
Cell structure analysis stands as a pivotal application of scanning electron microscopy. SEM offers an unparalleled view of cell morphology and organization. This high-resolution imaging technique highlights the fine details of cellular components, such as membranes and organelles. By capturing these intricacies, SEM aids researchers in understanding cellular functions and their roles in health and disease. The distinct advantage of SEM is its ability to render three-dimensional images of cells, allowing for a comprehensive assessment of spatial relationships within biological tissues. However, it is essential to note that sample preparation for SEM may impact the natural state of cells, introducing potential artifacts that must be carefully managed.
Tissue Examination
Tissue examination through SEM is another crucial application that provides detailed insights into tissue architecture. This technique plays a significant role in histopathology, where it is used to investigate various diseases, including cancers. The ability to visualize tissue at the microstructural level enables better diagnosis and understanding of pathological changes. The key characteristic of tissue examination via SEM lies in its precision, which offers a distinct perspective compared to traditional histological techniques. Though SEM provides rich detail, there may be challenges concerning sample viability and preservation, which could limit the longevity of the findings in certain scenarios.
Materials Science Applications
Surface Analysis
Surface analysis is among the foremost applications of scanning electron microscopy in materials science. SEM allows researchers to examine surface topographies and compositions at micro and nanoscales. This capability is crucial in developing new materials and optimizing surface properties, especially in industries such as electronics and aerospace. The detailed surface information provided by SEM helps researchers to make informed decisions regarding material functionalities. A significant advantage of surface analysis through SEM is the precision in understanding surface defects and inconsistencies that could be detrimental to material performance. However, limitations exist regarding the depth of information SEM provides, as it primarily focuses on surface characteristics rather than bulk material properties.
Failure Analysis
Failure analysis is another vital application within materials science that utilizes SEM to investigate material failures. SEM can reveal the root causes of failure in manufacturing processes or mechanical structures. By examining fracture surfaces and failure modes, researchers gather critical information that guides improvements in materials and designs. A notable characteristic of failure analysis via SEM is its ability to link microscopic features to macroscopic failures, fostering a deeper comprehension of material behaviors under stress. While SEM excels in providing insightful analysis, challenges persist with sample preparation that may affect the results and interpretations of failures.
Nanotechnology Applications
Nanotechnology applications of scanning electron microscopy are vast and expanding. SEM is invaluable for visualizing nanoscale materials, enabling researchers to study structures such as nanoparticles and nanocomposites. The high-resolution imaging capabilities of SEM facilitate advancements in developing nanomaterials for various applications, including drug delivery and energy storage. By offering precise morphological details, SEM helps drive innovations in nanotechnology. While SEM effectively aids in advancing nanotechnology, it also presents challenges of resolution and sample manipulation at such small scales, necessitating careful consideration by researchers.
Challenges in Scanning Electron Microscopy
The remarkable capabilities of scanning electron microscopy (SEM) come with a set of challenges. Understanding these challenges is crucial for effectively utilizing SEM in scientific research and applications. This section will discuss several significant hurdles faced by practitioners in the field, namely cost and maintenance of equipment, sample limitations, and data interpretation difficulties. Recognizing these issues can help researchers make informed decisions when using SEM for their work.
Cost and Maintenance of SEM Equipment
Acquiring a scanning electron microscope is a substantial investment. The upfront cost is only the beginning. Maintenance is needed to keep the device functioning at optimal levels. Regular calibration and servicing can be expensive. Moreover, specialized technicians are often required to perform maintenance, further raising the total cost.
Factors influencing the cost include:
- Initial Purchase Price: High-end SEM systems can cost several hundred thousand dollars.
- Upgrades: As technology evolves, upgrades may be necessary to keep up with new applications or imaging capabilities.
- Consumables: Supply costs can also add up quickly. Regular use of SEM requires various materials such as vacuums, and other replacement parts.
Many institutions may find it difficult to justify the high operational cost for infrequent usage. This might lead to underutilization of potentially invaluable technology.
Sample Limitations
The type of samples that can be examined using SEM is another area of concern. Not every material or specimen is suitable for SEM imaging. This limitation often stems from the requirements of the SEM technique.
Consider these points regarding sample limitations:
- Conductivity: Non-conductive samples often require a conductive coating, which can alter the sample properties.
- Size and Shape: Larger samples may not fit into the SEM chamber, limiting the type of specimens that researchers can analyze.
- Fragility: Sensitive biological samples can be damaged by the vacuum environment required in SEM, leading to loss of critical structural information.
Researchers must navigate these restrictions creatively, sometimes compromising on sample quality or the specific information they seek.
Data Interpretation Challenges
Interpreting SEM images is not without its complexities. Even with high-quality images, the data derived is subject to misinterpretation or biases that can skew results.
Some challenges in data interpretation include:
- Complex Image Structures: SEM images can display intricate details that may require a high level of expertise to interpret correctly.
- Artifacts: Distortions and artifacts can arise during imaging, misleading researchers about the actual sample structure or properties.
- Quantitative Analysis: Extracting quantitative data from SEM images can be challenging due to resolution limits and varying imaging modes.
Fostering a better understanding of these interpretation challenges may improve the accuracy of conclusions drawn from SEM imagery.
"Understanding the limitations and challenges of scanning electron microscopy is as vital as recognizing its capabilities. It prepares researchers to engage with SEM technology more effectively."
Each of these challenges highlights the need for careful planning and execution when utilizing scanning electron microscopy. Inadequate attention to these issues can result in limited findings or erroneous conclusions, underscoring the importance of a well-informed approach to using SEM.
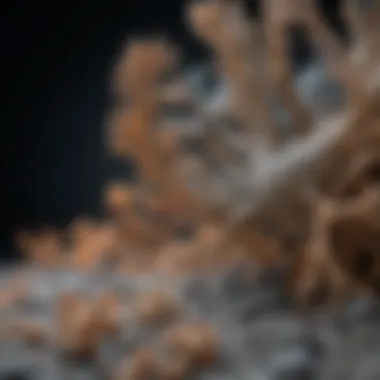
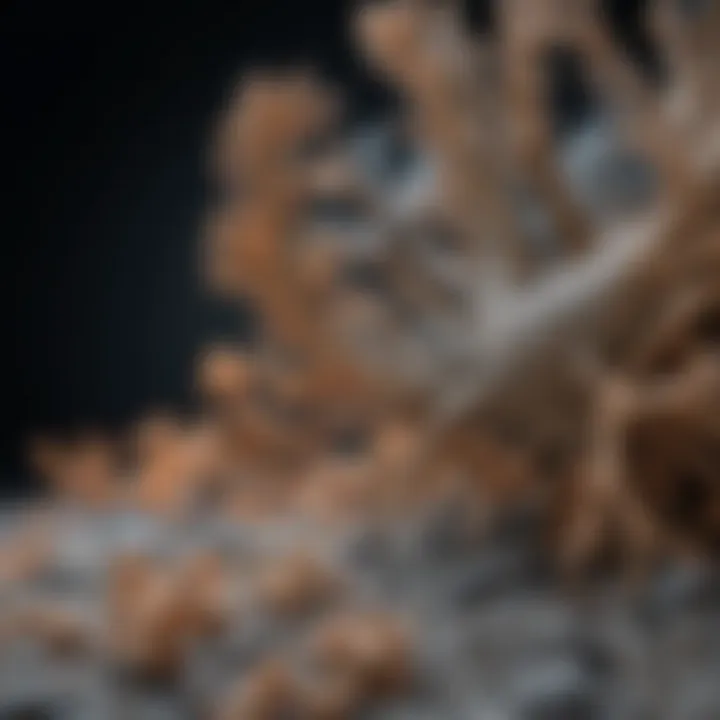
Advancements in Scanning Electron Microscopy Technology
The field of scanning electron microscopy (SEM) has seen notable advancements that enhance not just the quality of images but also the scope of applications in various scientific disciplines. These developments are crucial for researchers and professionals who rely on SEM for detailed analysis. The enhancements in technology provide better resolution, faster imaging, and greater versatility in imaging techniques, which collectively improve the efficiency of scientific investigations.
Technological Innovations
Recent innovations have transformed SEM capabilities considerably. Modern scanning electron microscopes are now equipped with advanced detectors, including field emission guns, which allow for higher-resolution images than traditional sources. This results in finer details being captured, which is essential for applications in nanotechnology and materials science. Additionally, software improvements facilitate easier data interpretation and analysis, allowing researchers to obtain quantitative measurements from their SEM images.
Some of the important innovations in SEM technology include:
- Automated Focus and Calibration: Advances in automation reduce the time a researcher spends on setup and calibration, improving overall productivity.
- 3D Imaging: With techniques such as serial block face imaging, SEM can now create 3D reconstructions of samples, providing richer data than 2D images alone.
- Integration with Other Techniques: The combination of SEM with techniques such as energy-dispersive X-ray spectroscopy (EDX) allows for more comprehensive analyses of material composition alongside morphology.
These innovations not only improve the imaging but also enhance the reliability of results, making SEM a more effective tool for various research tasks.
Future Directions in SEM Research
Looking ahead, the future of SEM appears promising, with ongoing research focusing on several areas to enrich its applications further. Possible directions include:
- In-situ Analysis: Developing techniques that allow SEM to capture dynamic processes within samples will enable real-time observations, particularly valuable in biological and materials research.
- Improved Resolution Techniques: Continued efforts to push the boundaries of resolution will facilitate the study of even smaller structures, critical in nanotechnology.
- Portable SEM Devices: The creation of smaller, more portable SEM units could expand the accessibility of SEM technology, allowing for field studies and real-time analysis.
In summary, advancements in scanning electron microscopy technology not only enhance imaging capabilities but also broaden the scope of its scientific applications. Researchers must remain informed about these changes, as they hold the potential to significantly impact various fields, enhancing our understanding of intricate structures at micro and nano scales.
Ethical Considerations in Scientific Imaging
Ethical considerations in scientific imaging play a vital role in maintaining the integrity and credibility of research in the field of scanning electron microscopy (SEM). The impact of these considerations extends from the reproducibility of results to publication ethics. In a discipline that relies heavily on visual evidence, the way that images are acquired, interpreted, and presented is crucial. Ensuring ethical practices not only safeguards scientific progress but also fosters public trust in scientific findings.
Reproducibility and Transparency
Reproducibility is a cornerstone of scientific research. In SEM studies, this means that other researchers should be able to replicate the experiments and obtain similar imaging results. Transparency in methodologies is necessary for establishing reproducibility. Providing detailed protocols for sample preparation, imaging settings, and data analysis is essential. When researchers share their methods, they allow others to verify their findings.
The importance of reproducibility becomes evident when considering the advancement of science. When results are repeatable, they strengthen the evidence for certain theories or conclusions. Conversely, non-reproducible results can lead to skepticism about a study's validity.
To promote reproducibility, researchers should:
- Document all steps in the imaging process. Include specifics about the SEM equipment and settings, as well as environmental conditions during imaging.
- Publish comprehensive data. Share original images and datasets, allowing peers to explore and analyze them further.
- Engage in open discussions. Contributing to forums and journals about the findings can greatly enhance transparency and provide opportunities for critique and improvement.
Publication Ethics
Publication ethics encompass the ethical responsibilities of scientists related to the submission and dissemination of research. In SEM studies, ethical concerns arise surrounding authenticity, authorship, and peer review processes.
One major aspect is the authenticity of images. Researchers must ensure that the images presented have not been manipulated or altered in ways that misrepresent the sample or findings. Misleading images can lead to erroneous conclusions and create a ripple effect of mistrust within the scientific community.
Furthermore, proper credit must be given to all contributors involved in the research. This includes acknowledgment of individuals or organizations that provided resources or insights. Disregarding these practices may not only tarnish reputations but could also compromise collaborative efforts in the future.
Lastly, the peer review process should remain impartial and fair. It is crucial that submitted works receive objective critiques without bias. This process supports the integrity of published research and ensures that only valid, high-quality findings reach the scientific community and, by extension, the public.
"Scientific integrity is not a luxury; it is essential to the advancement of knowledge and the trustworthiness of scientific inquiry."
In summary, adhering to ethical considerations in scientific imaging is fundamental to the robustness of SEM research. By emphasizing reproducibility, transparency, and publication ethics, researchers can contribute to a more reliable and reputable scientific discourse.
Culmination
The conclusion of this article is vital as it succinctly encapsulates the key themes and findings related to scanning electron microscopy. This section serves not only as a recap but also as a reflective opportunity for the reader to consider the broader implications of SEM photographs in various scientific domains.
Understanding the significance of scanning electron microscope imagery is crucial for students, researchers, and professionals. These images offer rich detail and insights impossible to capture using conventional methods. In disciplines such as biology, materials science, and nanotechnology, SEM pictures play an essential role in elucidating complex structures and properties.
One of the specific benefits outlined in this narrative includes the ability to analyze surface morphology and topography at a microscale. This capability can lead to groundbreaking discoveries or improvements in product design and testing. The necessity for reproducibility and transparency in scientific imaging cannot be understated either, as these factors underpin the integrity and advancement of research.
Summary of Key Points
- Importance of SEM Imagery: Provides detailed insights across multiple scientific disciplines.
- Applications in Various Fields: Key for advancing knowledge in biology, materials science, and nanotechnology.
- Challenges and Ethical Considerations: The need for reproducibility, transparency, and ethical practices in scientific imaging.
Future of Scanning Electron Microscopy
The future of scanning electron microscopy appears promising, given the rapid advancements in technology and its increasing accessibility. Researchers envision a landscape where SEM becomes more integral in various scientific inquiries, thanks to innovations in image acquisition and analysis techniques.
Emerging trends indicate that there will be a push toward enhanced resolution and faster imaging capabilities. Coupled with developments in software for image processing, these advancements aim to facilitate even deeper insights into structures at the nanoscale. This technology can be critical for breakthroughs in diverse areas like drug delivery systems in pharmaceuticals or the development of novel materials in engineering.
Moreover, interdisciplinary collaboration is likely to cultivate further refinements in SEM technologies. As needs in research evolve, the instrumentation and methodologies can adapt, ensuring that SEM remains at the forefront of scientific imagery.
In summary, scanning electron microscopy holds a pivotal role in the continued exploration of material and biological sciences and is expected to significantly improve as technology and ethical standards evolve.