Understanding the Importance of Van der Waals Bonds
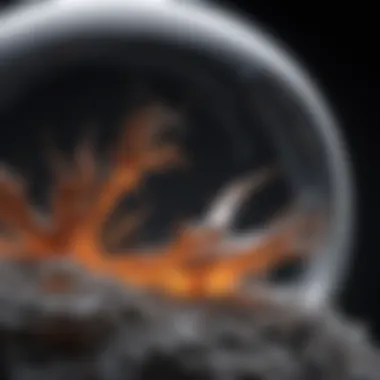
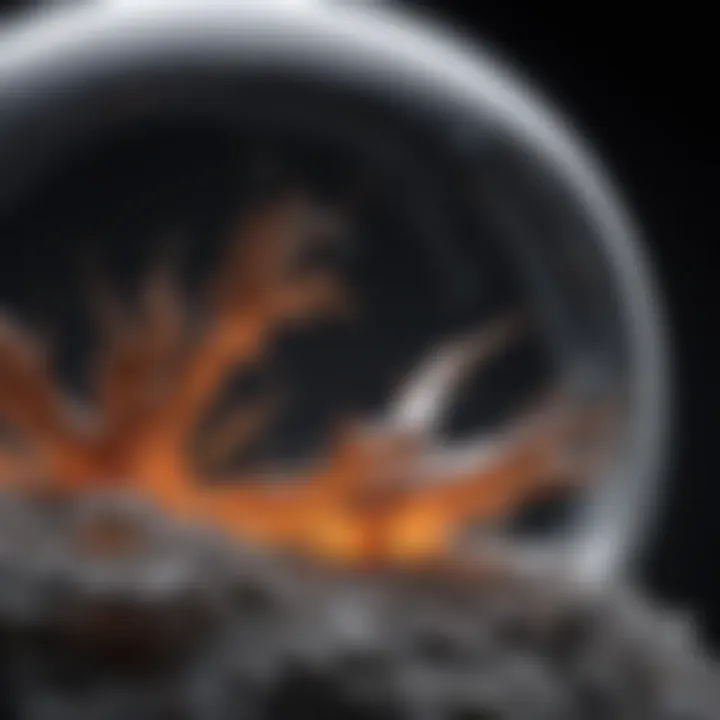
Intro
Van der Waals bonds are an intriguing aspect of molecular interactions, playing a significant role in various scientific fields. These bonds, although weaker than covalent or ionic bonds, are essential in understanding the behavior of gases, liquids, and solids. The nature of these bonds is grounded in the presence of induced polarization in molecules, allowing for attractions even among neutral species. Understanding Van der Waals forces sheds light on many processes in physics and chemistry, particularly those involving molecular stability and material properties.
In this exploration, we will highlight important aspects of Van der Waals bonds. We will delve into their classification, characteristics, and their impact on biological systems, which may seem mundane but hold tremendous implications for fields like drug design and material science. Through this discussion, readers will gain comprehensive insights into the significance of Van der Waals bonds in the broader context of intermolecular forces and their practical applications.
Preface to Van der Waals Bonds
Van der Waals bonds represent a group of weak intermolecular forces that play a crucial role in determining the physical and chemical properties of substances. While often overlooked in favor of stronger covalent or ionic bonds, the understanding of Van der Waals interactions is essential for a comprehensive grasp of molecular behavior. This section will present an overview of the significance of Van der Waals bonds, outlining their characteristics, historical background, and relevance in various scientific domains.
Definition and Characteristics
Van der Waals bonds can be defined as weak, non-covalent interactions that arise from temporary dipoles in molecules. These dipoles can result from fluctuations in electron distribution, leading to attractions between neutral molecules. There are several different types of Van der Waals interactions; these include London dispersion forces, dipole-dipole interactions, and dipole-induced dipole forces. Each type varies in its strength and applicability, depending on the nature of the molecules involved.
The characteristics of Van der Waals bonds include a dependence on molecular size, shape, and surface area. Larger and more polarizable molecules tend to exhibit stronger forces. Itβs important to note that, despite their relatively low strength compared to other bonds, Van der Waals interactions are significant contributors to molecular stability. Their cumulative effect can stabilize structures such as biological macromolecules including proteins and nucleic acids.
Historical Background
The concept of Van der Waals bonds is rooted in the early studies of molecular forces and interactions. Johannes Diderik van der Waals, a Dutch physicist, first introduced the idea in the late 19th century. He observed that gases did not behave ideally under certain conditions and developed his equation to describe gas behavior under these circumstances.
In understanding how molecules interact, Van der Waalsβ work laid the foundation for the field of molecular chemistry. His insights demonstrated that even weak forces could have a profound impact on molecular properties and behaviors. This recognition had significant implications for future research, influencing the study of various fields including physical chemistry, biology, and materials science.
Through his work, the importance of these weak interactions became apparent. Van der Waals bonds are integral to processes such as protein folding and the assembly of complex molecular structures.
"Understanding the subtleties of Van der Waals forces allows scientists to manipulate molecular interactions for various applications in chemistry and biology."
In summary, Van der Waals bonds are fundamental to the comprehension of molecular interactions. Their study not only highlights the diversity of forces in molecular chemistry but also underscores the importance of looking beyond stronger bonds to appreciate the full spectrum of interactions that govern material and biological systems.
Types of Van der Waals Bonds
Van der Waals bonds, though weaker than covalent and ionic bonds, play a crucial role in the stability and interaction of molecules. Understanding the various types of Van der Waals bonds offers insight into how these interactions govern many fundamental processes in chemistry and biology. In this article, we will delve into three primary types of Van der Waals bonds: London dispersion forces, dipole-dipole interactions, and dipole-induced dipole forces. Each type is significant in terms of molecular behavior and stability, contributing to the cohesion and properties of different substances.
London Dispersion Forces
London dispersion forces are the weakest form of Van der Waals interactions. They arise due to temporary shifts in electron density within molecules, resulting in fluctuations that create short-lived dipoles. These forces are present in all molecules, regardless of whether they are polar or nonpolar.
The significance of London dispersion forces can be observed in various contexts, such as:
- Nonpolar Molecules: Even nonpolar molecules can exhibit these forces, leading to attractions that facilitate condensation into liquids under certain conditions.
- Molecular Size: As molecular size increases, the strength of London dispersion forces increases due to the availability of more electrons for fluctuations.
- State Changes: These forces influence phase transitions, including boiling and melting points.
It is important to consider London dispersion forces when studying substances like noble gases or hydrocarbons, where these interactions dictate physical properties.
Dipole-Dipole Interactions
Dipole-dipole interactions occur between polar molecules, where the positive end of one molecule attracts the negative end of another. This type of interaction is stronger than London dispersion forces and can significantly impact the physical properties of substances.
The importance of dipole-dipole interactions is evident in several ways:
- Solubility: Polar substances tend to dissolve well in polar solvents due to dipole-dipole interactions, which enhances solute-solvent interactions.
- Melting and Boiling Points: Molecules with strong dipole-dipole attractions typically have higher melting and boiling points compared to nonpolar molecules of similar size.
- Biological Systems: These interactions play a critical role in the stability of biomolecules. For instance, the specific arrangements of atoms in a protein can be maintained through dipole-dipole interactions, influencing protein folding and function.
Thus, understanding dipole-dipole interactions helps explain the behavior of many materials and their applications in various fields.
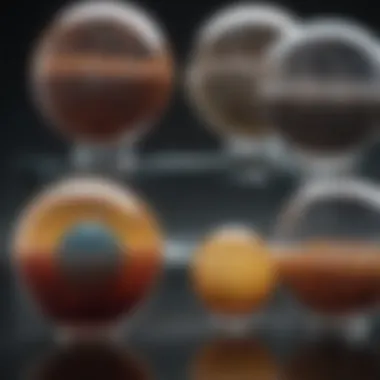
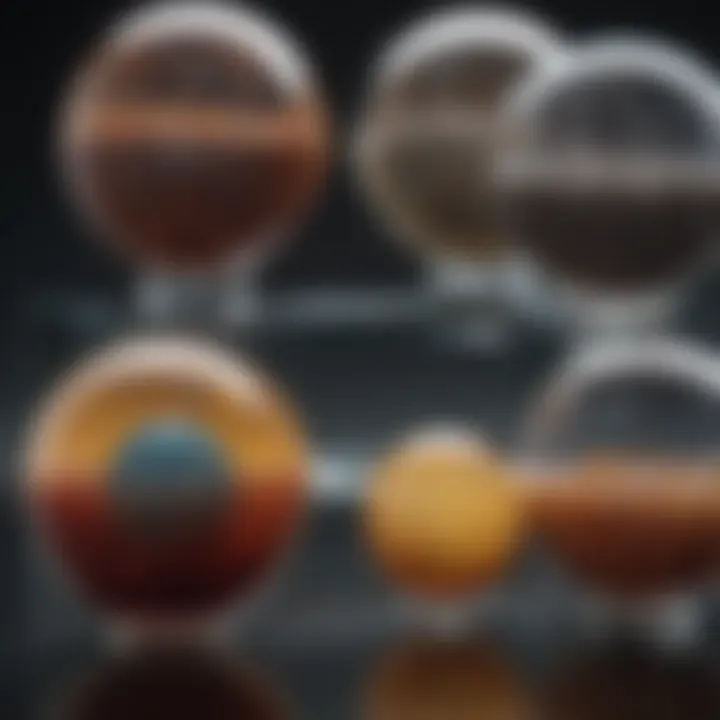
Dipole-Induced Dipole Forces
Dipole-induced dipole forces occur when a polar molecule induces a dipole in a neighboring nonpolar molecule. Though generally weaker than dipole-dipole interactions, they can still significantly affect molecular behavior.
This type of interaction holds relevance in scenarios such as:
- Mixing Different Substances: When a polar molecule approaches a nonpolar one, the temporary dipole created can lead to some interaction, influencing how substances mix.
- Impacts on Properties: The ability of a nonpolar molecule to be influenced by a polar molecule can affect various properties, including such phenomena as solubility and boiling points.
- Biological Significance: In the context of biochemical systems, dipole-induced dipole forces can impact interactions between nonpolar regions of proteins or lipids with polar environments.
Recognizing the presence and significance of dipole-induced dipole forces can enhance our understanding of molecular interactions across various chemical contexts.
Mechanisms of Van der Waals Interactions
Understanding the mechanisms of Van der Waals interactions is crucial for grasping the broader implications these forces have in molecular chemistry and physics. Van der Waals bonds are often weak but play a key role in various physical and biological processes. Their mechanisms are intricate and involve the interplay of electrons and the spatial organization of molecules.
Role of Electrons
Electrons are fundamental to the formation of Van der Waals interactions. The distribution of electrons around a molecule contributes to its polarizability. This means some parts of a molecule may develop a temporary dipole due to fluctuations in electron density. These temporary dipoles can induce dipoles in neighboring molecules, leading to attractive forces.
This interaction results in what we refer to as London dispersion forces. Although these forces are minimal, they become significant in large systems, contributing notably to the stability of molecular structures. As molecules approach one another, these interactions become stronger and can influence various properties, such as boiling points and solubility.
Understanding how electrons behave in these scenarios can provide insights into molecular behavior under different conditions.
Molecular Geometry and Orientation
Molecular geometry and orientation are crucial factors in the effectiveness of Van der Waals interactions. The spatial arrangement of atoms in a molecule determines how closely it can approach others. For instance, molecular symmetry can enhance the strength of these forces, as closely aligned molecules can maximize their surface area contact, leading to increased interaction.
The orientation of polar molecules can further intensify dipole-dipole interactions. In condensed phases like liquids or solids, the orientation becomes particularly important. As molecules align themselves, the resultant forces can alter physical properties significantly.
In summary, both the behavior of electrons and the geometry of molecules are central to understanding Van der Waals interactions. These mechanisms are not just theoretical but provide a basis for numerous applications in biological and materials science.
"Van der Waals interactions, though comparatively weak, are pivotal in stabilizing complex structures in both biological systems and synthetic materials."
This foundation allows researchers to design experiments and applications, harnessing the significance of such interactions within various scientific domains.
Importance of Van der Waals Bonds
Understanding the importance of Van der Waals bonds is crucial in many areas of science, particularly in chemistry and biology. Van der Waals bonds are significant due to their role in stabilizing molecular structures and influencing interactions among molecules. These forces are much weaker than ionic and covalent bonds, yet they are ubiquitous in nature. Their presence is evident in various biological systems and material sciences, underscoring their wide-ranging implications.
Biological Relevance
Protein Folding
The role of Van der Waals interactions in protein folding is particularly important. Proteins adopt specific shapes to perform their functions, and these shapes are determined by interactions between their constituent amino acids. Van der Waals forces contribute to the stability of the folded structure. This is essential for the protein's activity. When proteins fold, the nonpolar side chains tend to cluster together to minimize exposure to water, which is driven by hydrophobic forces. The compact arrangement allows for optimal interaction and efficiency.
A key characteristic of protein folding is its inherent complexity. Each protein has a unique sequence of amino acids, leading to distinct folding patterns. This is a beneficial aspect for the article because it emphasizes that even small changes in sequence can result in significant alterations in structure and function. However, improper folding can lead to diseases, such as Alzheimer's. Therefore, understanding these interactions can also guide biomedical research.
DNA Structure
Van der Waals forces also play a critical role in the structure of DNA. The double helix shape of DNA is held together, in part, by these interactions between the bases on opposite strands. A vital aspect of DNA structure is its stability, which allows for the replication and storage of genetic information. The stacking of base pairs and their alignment depend on these weak interactions. This makes it a focus in genetic research and molecular biology.
The unique feature of DNA's structure is its ability to form various conformations depending on the conditions in which it is found. This flexibility is advantageous in processes like transcription and replication. However, it also poses challenges; mutations or abnormal interactions can lead to genetic disorders.
Material Science Applications
Nanotechnology and Devices
In material science, nanotechnology leverages Van der Waals bonds in the creation of devices at the nanoscale. These interactions are crucial for assembling nanomaterials, such as carbon nanotubes and graphene. They exhibit remarkable strength and electrical properties, making them ideal for advanced applications. The use of Van der Waals forces in this context is beneficial as it can lead to innovations in electronic devices, sensors, and energy storage systems.
A key characteristic of nanotechnology is the ability to manipulate materials at a molecular level. With proper understanding of these forces, researchers can develop new materials that meet specific requirements. However, controlling these interactions can be challenging due to the variability in material properties at the nanoscale.
Composite Materials
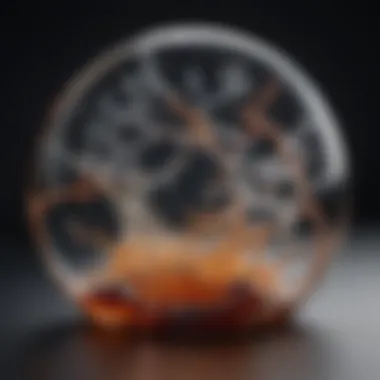
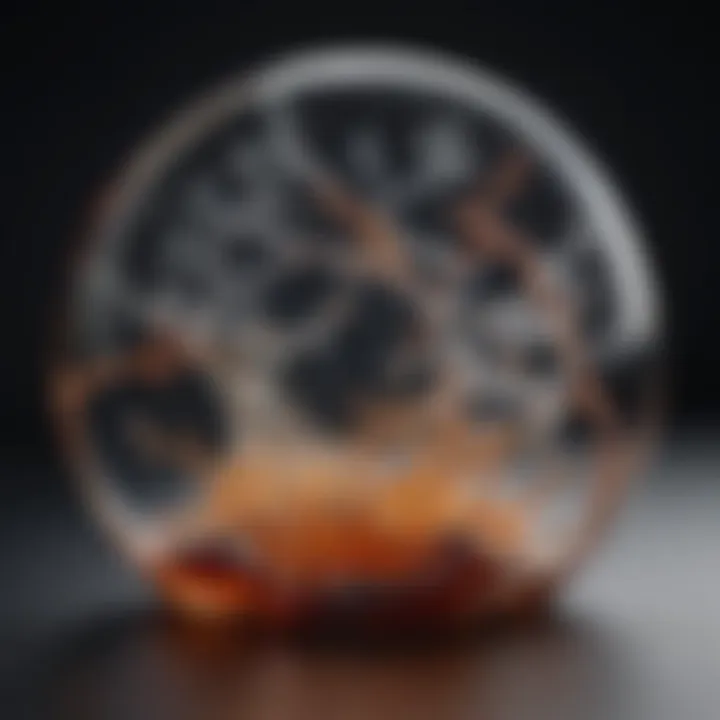
Van der Waals bonds also play a significant role in the development of composite materials. These materials combine different substances to enhance performance while maintaining lightweight characteristics. Van der Waals interactions help to improve the adhesion between fibers and matrices, which is essential for the structural integrity of composites.
The unique feature of composite materials is their customizable properties. By varying the components, scientists can create materials tailored for a wide range of applications, from aerospace to construction. However, challenges exist in ensuring uniform distribution of components and understanding the full effect of Van der Waals forces on material performance.
In summary, Van der Waals bonds are fundamental forces that contribute significantly to biological processes and technological advancements. Their roles in protein folding and DNA structure highlight their importance in biology, while their implications in nanotechnology and composite materials underscore their relevance in material science.
Van der Waals Forces vs. Other Intermolecular Forces
Van der Waals forces are crucial when comparing different types of intermolecular forces. Understanding these forces provides insight into many chemical and physical processes. These types of forces help in dictating how molecules interact. They play a key role in the properties of materials as well as biological systems.
Importance of Van der Waals Forces
Van der Waals forces include interactions such as London dispersion forces, dipole-dipole interactions, and dipole-induced dipole forces. These interactions are weaker than ionic or covalent bonds but are still significant. They affect properties like melting points, boiling points, and solubility in various substances. Most importantly, Van der Waals forces are present in all molecules, making them universally applicable across different systems.
Comparison with Ionic Bonds
Ionic bonds form through strong electrostatic attractions between charged ions. These bonds are very strong and are essential in forming compounds like sodium chloride. In contrast, Van der Waals forces are considerably weaker. This weakness means that ionic compounds have much higher melting and boiling points than substances held together by Van der Waals interactions.
- Strength: Ionic bonds are stronger, forming stable structures. Van der Waals forces are weak and transient.
- Role in Compounds: Ionic bonds are key to creating solid lattices. Van der Waals forces allow for transient molecular interactions.
- Relevance in Solutions: In solutions, ions can dissociate and affect properties differently than molecular substances primarily held together by Van der Waals forces.
Thus, while ionic bonds provide stability, Van der Waals forces allow for flexibility and adaptability in molecular interactions. Their roles often converge in complex systems like proteins and polymers.
Comparison with Covalent Bonds
Covalent bonds arise from the sharing of electrons between atoms. This electron sharing creates strong and stable molecules. When we compare this to Van der Waals forces, the differences become clear. Covalent bonds are formed through direct interactions and can be quite strong.
- Bond Strength: Covalent bonds are significantly stronger than Van der Waals forces, leading to more stable molecules.
- Structural Influence: Covalent bonds dictate the framework of a molecule, while Van der Waals forces affect how those molecules interact with each other.
- Molecular Dynamics: In a covalent compound, the absence of strong Van der Waals forces can lead to a lack of interactions crucial for biological processes, like enzyme-substrate binding.
In summary, while Van der Waals forces are critical in a variety of scientific contexts, their nature is fundamentally different from both ionic and covalent bonding. They complement these stronger interactions, filling gaps in molecular stability and providing the necessary flexibility for various chemical behaviors.
Computational Approaches to Studying Van der Waals Bonds
Understanding Van der Waals bonds requires a strong foundation in computational methods. These methods provide powerful tools that enable researchers to explore the nature of these interactions with precision and depth. Computational approaches allow for the modeling of molecular systems under various conditions and can simulate environments that are difficult to replicate experimentally. This section will delve into two primary methodologies: Molecular Dynamics Simulations and Quantum Mechanical Methods, both of which play critical roles in enhancing our understanding of Van der Waals interactions.
Molecular Dynamics Simulations
Molecular Dynamics (MD) simulations are essential for studying the behavior of molecules over time. By modeling the motion of atoms and molecules, MD simulations can reveal how Van der Waals forces influence molecular configurations and dynamics. The simulations work by solving Newton's equations of motion, which govern the movement of particles under the influence of intermolecular forces.
Key advantages of Molecular Dynamics include:
- Visualization of Molecular Behavior: MD simulations can create a visual representation of molecular interactions. This aids in understanding how Van der Waals forces contribute to structural changes within biomolecules or materials.
- Long Timescale Simulation: MD can simulate processes that occur over longer timescales compared to typical experimental techniques. This provides insights into the stability of structures influenced by Van der Waals interactions, especially in biological contexts like protein folding.
- Thermodynamics and Kinetics: With MD simulations, researchers can calculate thermodynamics properties and study reaction kinetics that depend on Van der Waals bonds, allowing for a comprehensive analysis of molecular behavior.
However, the accuracy of MD simulations relies on the force field used, which describes how atoms interact. Force fields must accurately capture the nuances of Van der Waals interactions; otherwise, the results can be misleading.
Quantum Mechanical Methods
Quantum Mechanical Methods (QMM) provide a more fundamental approach to understanding molecular interactions, including Van der Waals bonds. These methods rely on quantum mechanics principles to predict the electronic structure of molecules.
QMMs have distinct benefits:
- Accurate Energy Calculations: These methods are capable of providing highly accurate energy profiles for molecular systems, essential for understanding the strength and nature of Van der Waals interactions.
- Ab Initio and Density Functional Theory: Methods such as Ab Initio calculations and Density Functional Theory (DFT) are widely used for examining intermolecular forces. DFT, in particular, has become a popular choice due to its balance between computational cost and accuracy.
- Integration with Other Techniques: QMM can be combined with other computational methods, providing a more comprehensive understanding of molecular systems influenced by Van der Waals forces. For instance, coupling QMM with MD can enhance the realism of simulations while maintaining high accuracy in interaction modeling.
Despite their advantages, Quantum Mechanical Methods can be computationally intensive, limiting their application to smaller systems or requiring significant computational resources.
Challenges in Quantifying Van der Waals Interactions
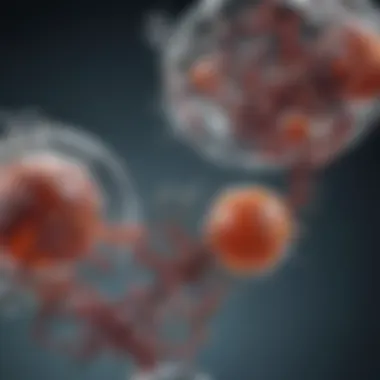
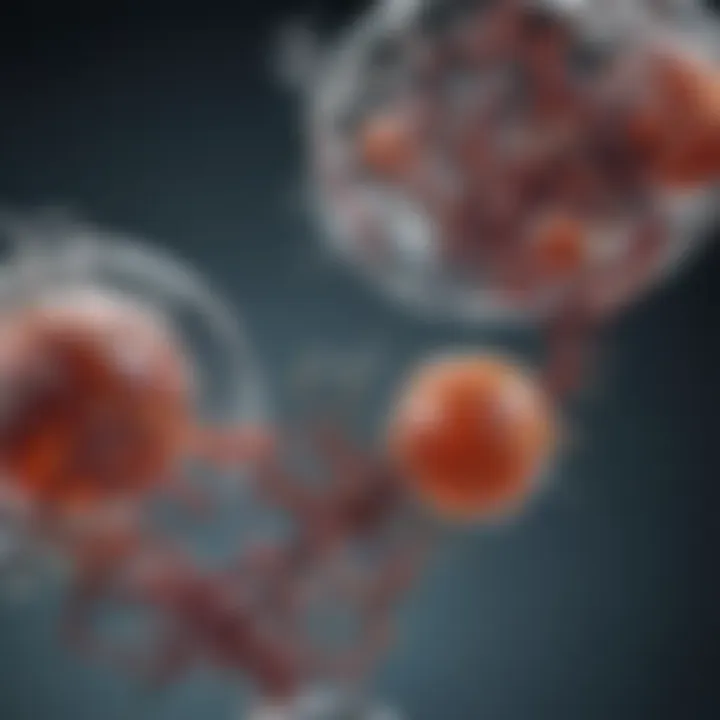
The study of Van der Waals interactions reveals numerous complexities in quantification. Recognizing these challenges is vital for both practical applications and theoretical understanding. Quantifying these bonds can have significant ramifications in various fields, such as materials science and biochemistry. The difficulty lies not just in measurement but also in interpreting these results in light of existing theories. Accurate quantification can aid in the design of new materials and the understanding of biological systems.
Measurement Difficulties
Measuring Van der Waals forces presents a range of problems that stem primarily from their intrinsic weakness. These interactions are generally much weaker than other types of chemical bonds, such as covalent or ionic bonds. As a result, high precision is required to detect and quantify their presence. Standard methods, such as atomic force microscopy, can capture these interactions; however, experimental setup must be meticulously designed to eliminate noise and interference.
Moreover, environmental factors complicate measurements. Variations in temperature, humidity, and electromagnetic interference can all skew results. The range of these forces is also a contributing factor; since they act over a short distance, pinpointing the exact moment when Van der Waals forces become significant can be challenging.
Van der Waals forces are often overshadowed by stronger interactions, making their identification and measurement a continual challenge in molecular research.
Theoretical Limitations
The theoretical tools used to describe Van der Waals interactions also face limitations. Conventional models, such as the Lennard-Jones potential, strive to approximate these forces but often do so insufficiently. These models may fail to account for the complexities present in multi-component systems or environments. As systems become more complex, the assumptions made by these models may no longer hold, leading to inaccurate predictions.
Furthermore, computational methods, while powerful, require significant computational resources. Even then, results can differ based on the chosen algorithm or the degree of approximation. Peer consensus on the most accurate theoretical models is often lacking, which creates discrepancies between observed and predicted values.
In summary, the challenges in quantifying Van der Waals interactions are twofold: measuring weak forces in a noisy environment and navigating the limitations of current theoretical frameworks. Addressing these issues is essential for advancing our understanding of molecular interactions.
Future Directions in Van der Waals Research
The study of Van der Waals bonds is evolving. New research continuously unravels their complex nature and broad applicability. This section highlights key areas of future exploration, emphasizing not just new technological avenues but the interdisciplinary nature of these advancements.
Innovations in Material Design
Recent insights into Van der Waals forces are shaping material design in unprecedented ways. This involves utilizing these weak interactions to craft materials with specific traits. For example, materials that exhibit enhanced flexibility or improved thermal conductivity are possible through strategic manipulation of Van der Waals interactions.
In nanotechnology, the application of Van der Waals bonds is crucial. Researchers are exploring how to engineer two-dimensional materials, like graphene, to exploit their unique properties. These materials promise applications in electronics, energy storage, and beyond.
Key benefits include:
- Customized material properties tailored for specific applications.
- Reduction of waste during the manufacturing process due to efficient design techniques.
- The potential for sustainable materials that minimize environmental impact.
Interdisciplinary Approaches
Van der Waals research benefits from collaborative efforts across various scientific disciplines. Fields such as physics, chemistry, biology, and materials science all intersect in this area, facilitating a comprehensive understanding of these bonds.
For example, integrating computational models from physics with experimental results in biology can lead to breakthroughs in drug design. Understanding how biomolecules interact via Van der Waals forces sheds light on numerous biological processes, from protein folding to enzyme activity.
The collaboration also extends to fields like engineering, where insights into molecular interactions inform the design of smart materials that can adapt to changing environmental conditions.
"Interdisciplinary work can amplify innovations in the use of Van der Waals interactions, providing methods to address complex problems in modern science.β
Finale
The conclusion serves an essential role in summarizing the topics covered in this article on Van der Waals bonds. It is crucial because it synthesizes the critical information presented and reinforces the significance of these interactions. Van der Waals bonds, while often overlooked, hold considerable implications in various scientific fields, including chemistry, biology, and material science. By reviewing the key points, readers can consolidate their understanding of how these interactions function and their impact on molecular stability and material properties.
Summary of Key Points
In summary, several vital elements have emerged throughout this discussion:
- Definition and Types: Van der Waals bonds encompass diverse interactions, primarily including London dispersion forces, dipole-dipole interactions, and dipole-induced dipole forces.
- Mechanisms: The nature of electrons and molecular geometry play critical roles in the manifestation of these forces, facilitating interactions across various environments.
- Biological and Material Applications: These bonds are fundamental to numerous biological processes, including protein folding and DNA structure, as well as material applications in nanotechnology and composite development.
- Comparative Forces: The distinctions between Van der Waals forces and other intermolecular forces like ionic and covalent bonds underscore their unique role in chemistry.
- Challenges and Future Directions: Research faces challenges in quantifying these interactions, yet future advancements in material design and interdisciplinary approaches hold promise for more in-depth understanding and applications.
Implications for Future Research
The implications for future research into Van der Waals bonds are expansive. Further investigations will likely reveal even deeper insights into:
- Advanced Material Design: The application of Van der Waals interactions in crafting new materials with tailored properties is a frontier for engineers and scientists. Understanding these forces can aid in the development of innovative nanomaterials.
- Interdisciplinary Insights: As research integrates various disciplines, the potential for breakthroughs in fields such as biochemistry and materials science becomes significant. Collaboration across these boundaries can yield novel applications.
- Refinement of Theoretical Models: Improved methodologies to measure Van der Waals interactions could lead to enhanced theoretical frameworks. This advancement will contribute to a more nuanced understanding of molecular interactions.
In sum, the future of Van der Waals bond research is not only promising but also essential for progress in multiple scientific domains. Emphasizing the fundamental role of these interactions will likely lead to innovative solutions to real-world problems.