The Impact of Mechanics Across Diverse Fields
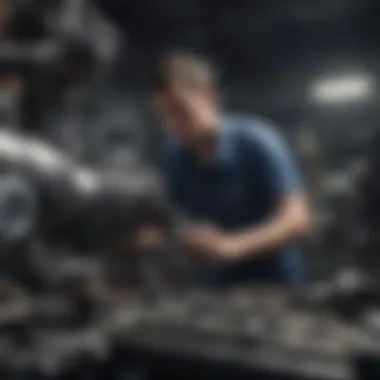
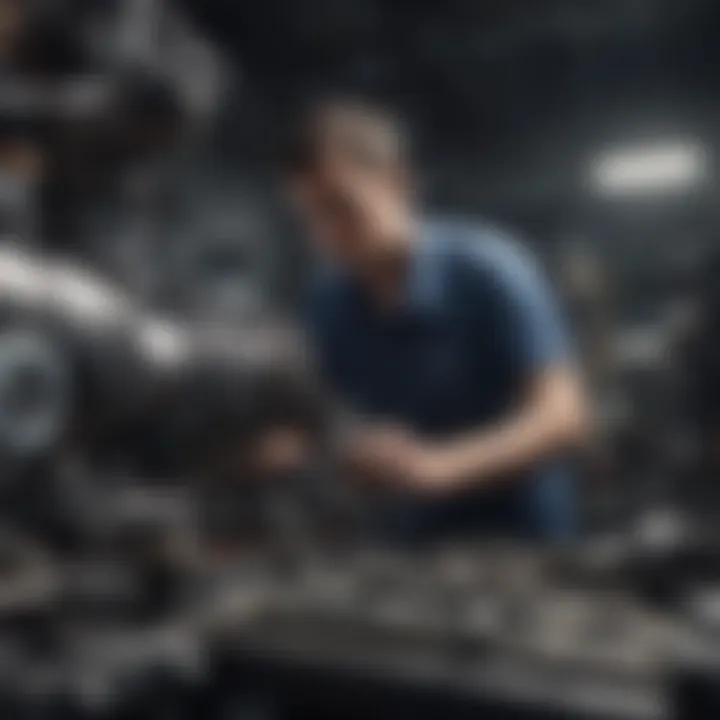
Intro
Mechanics is oftentimes viewed as a dry subject, a necessary evil that students must grapple with as they pursue their scientific ambitions. However, what many fail to recognize is that mechanics forms the backbone of a multitude of disciplines, acting as a bridge between theoretical knowledge and practical application. Whether itโs the architects designing skyscrapers that touch the clouds, or the engineers working behind the scenes to create sustainable transportation, the principles of mechanics underpin it all.
Across various domainsโ engineering, biomechanics, astrophysics, and even environmental scienceโ mechanics holds profound relevance. Each field employs its tenets, often in ways that are not immediately visible. Take biomechanics, for example. Itโs not merely about understanding how muscles and bones work in isolation; instead, it provides insights into optimizing movement and enhancing human performance, which could have implications ranging from athletics to rehabilitation.
In the realm of astrophysics, mechanics helps demystify the cosmos, allowing scientists to understand celestial phenomena and predict the behavior of planets and stars. Meanwhile, environmental science engineers leverage mechanics to devise solutions that tackle climate change, demonstrating how fundamental principles can lead to groundbreaking innovations that improve our planet.
As we dig deeper, this article will cast light on the intricate ways mechanics influences these diverse fields. By examining its far-reaching impact and applications, we will clarify how essential mechanics is to scientific progress and practical solutions.
"Mechanics is the art of balancing forces, demonstrating that everything is in constant motion, be it a planet orbiting a star or a human taking a step."
Understanding mechanics is not just academic; itโs a critical lens through which we can view and shape the world. Through a careful examination of these applications, we seek not only to educate our audience but to inspire curiosity about the underlying forces that govern the phenomena we observe in every corner of life.
Understanding Mechanics
The field of mechanics is foundational to our understanding of the physical world. It delves into the behavior of objects in motion and the forces acting upon them. Mechanics not only serves as a bedrock of scientific inquiry but also greatly influences various disciplines ranging from engineering to the natural sciences. Without a firm grasp of mechanics, many advancementsโbe it in transportation or architectural designโwould simply not be possible. The beauty of mechanics lies in its universality; principles derived from its study hold true across multiple contexts, simplifying complex scenarios into manageable principles.
Definition and Scope of Mechanics
Mechanics can be defined as the branch of physics that deals with the motion of objects and the forces that affect them. It encompasses a wide range of phenomena, making it an expansive area of study. To break it down:
- Kinematics: This is the description of motion without considering the forces that cause it. Understanding kinematics helps in predicting the trajectory of an object, be it a thrown ball or a spacecraft navigating through the cosmos.
- Dynamics: Unlike kinematics, dynamics examines the forces that cause motion. It dives deeper into how various forces interact to either accelerate, decelerate, or change the direction of objects. For example, the complex interactions in a mechanical engine involve principles of dynamics to ensure optimal performance.
In essence, mechanics covers:
- Classical Mechanics: Traditional theories primarily laid down by Newton, essential for everyday applications.
- Quantum Mechanics: This deals with particles at the microscopic level, radically altering our perception of physical interactions.
- Relativistic Mechanics: Developed by Albert Einstein, it examines the effects of velocity nearing the speed of light, notably in astrophysical contexts.
Historical Development of Mechanics
The journey of mechanics is a tale of evolution and revolution. Beginning in ancient times, the Greeks, especially Archimedes, laid the groundwork with concepts of levers and buoyancy. His work characterized early mechanics, yet was modded for centuries.
Fast forward to the late 17th century, Isaac Newton revolutionized mechanics with his laws of motion and gravitation. His work unifying celestial with terrestrial mechanics set the stage for advancements in various fields. Newton's laws explained how forces and motion interplay, providing a framework that remains critical today.
Throughout the 19th and 20th centuries, mechanics saw further refinements. The advent of thermodynamics illustrated the interplay of heat and work, effectively tying mechanics to energy considerations in processes ranging from engines to refrigerators. Meanwhile, the development of quantum theory in the 20th century forced a reconsideration of mechanistic principles, challenging classical norms while expanding the scope of physics.
Thus, the trajectory of mechanics reveals an intricate tapestry of ideas built upon by brilliant minds through time. Each step forward not only broadened our comprehension but also laid the groundwork for ongoing innovations that impact our lives every day.
Core Principles of Mechanics
The domain of mechanics serves as the bedrock for understanding how physical systems behave. When exploring the core principles of mechanics, we unveil the underpinnings of not just physics but also many applied sciences. By establishing a strong grasp of these principles, students, researchers, and professionals can enhance their analytical skills and optimize various engineering solutions. In essence, the core principles guide us through the intricate dance of forces and motions that govern our world.
Newtonian Mechanics
Newtonian mechanics, established by Sir Isaac Newton in the 17th century, lays the groundwork for classical mechanics. It's like the tried-and-true compass that navigates the terrain of motion and forces. This principle focuses on three fundamental laws:
- The Law of Inertia: An object at rest stays at rest unless acted upon by an external force. This principle reminds us that everything craves to maintain its status quo until something shakes it up.
- The Law of Acceleration: The acceleration of an object is directly proportional to the net force acting on it and inversely proportional to its mass. This law reveals why it's easier to push a shopping cart than a car โ lighter objects respond more readily to the forces imparted to them.
- The Law of Action-Reaction: For every action, there is an equal and opposite reaction. Itโs this principle that makes rockets launch skyward; as gases are expelled downward, the rocket moves upward. Such vivid interactions are the fabric of much of classical mechanics and have substantial applications in engineering disciplines.
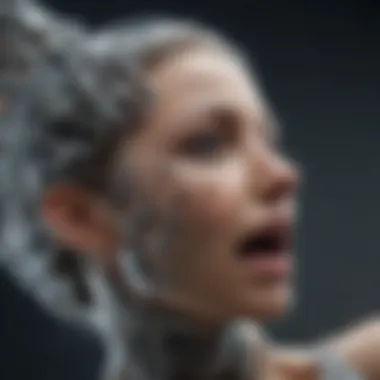
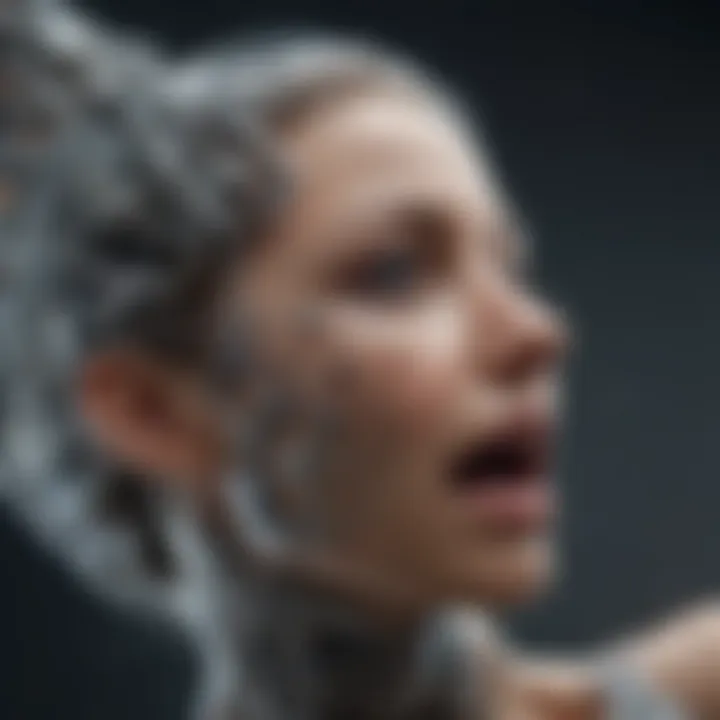
Understanding these laws is crucial for areas ranging from civil and aerospace engineering to everyday problem-solving scenarios. When engineers design bridges or aircraft, Newtonian mechanics helps optimize safety and efficiency, thus ensuring public trust in their creations.
Thermodynamics and Mechanics
Thermodynamics serves as the bridge between heat and mechanical work, transforming energy concepts into tangible applications. It encompasses the laws governing energy conversions and flows, laying the groundwork for many systems that operate in our daily lives. The four fundamental lawsโeach one a cornerstoneโdescribe how energy behaves in different states:
- Zeroth Law: If two systems are in thermal equilibrium with a third system, then they are in equilibrium with each other. This principle is vital for temperature measurement, allowing scientists to establish common references.
- First Law: Energy cannot be created or destroyed, only transformed from one form to another. This law reminds us to conserve energy, echoing through industries that rely on heat engines, such as automotive and manufacturing.
- Second Law: In any energy transfer, the total entropy of a closed system always increases. This notion emphasizes that energy transfers are inherently inefficient, a factor engineers must account for when designing systems.
- Third Law: As the temperature of a system approaches absolute zero, the entropy of a perfect crystal approaches zero. This law has profound implications in fields like cryogenics and material science.
Thermodynamics is intricately woven into the fabric of mechanical innovations. Whether it's air conditioning systems or the internal workings of car engines, deciphering these principles leads to improved performance and cost-efficiency.
Fluid Mechanics
Fluid mechanics involves the study of fluids (liquids and gases) and the forces acting upon them. Water flow in rivers, air currents that weather the planet, and even the blood circulating in our bodies fall under this umbrella. The behavior of fluids presents peculiar challenges due to their inherent characteristics, like viscosity and turbulence.
The main principles and equations used in fluid mechanics include:
- Continuity Equation: It stipulates that for an incompressible fluid, the mass flow rate must remain constant from one cross-section of a pipe to another.
- Bernoulliโs Principle: An increase in the speed of a fluid occurs simultaneously with a decrease in pressure. This principle elegantly explains why airplane wings generate lift and highlights its significance in hydraulic systems.
- Navier-Stokes Equations: These equations describe how the velocity field of a fluid behaves over time, encapsulating the physics of fluid motion. The complexity of these equations often makes them a topic of in-depth research in mathematics and engineering.
Fluid mechanics plays a crucial role in designing hydraulic systems, predicting weather patterns, and understanding biological systems. Its principles can enhance fluid-related innovations to address industry challenges effectively and sustainably.
"Through understanding mechanics, we unlock not just theoretical concepts but practical solutions to real-world challenges."
The core principles of mechanics not only inform theoretical explorations but also underpin advances in technology and industry. A nuanced understanding of these concepts allows professionals and researchers alike to contribute meaningfully to their respective fields.
Applications of Mechanics
The applications of mechanics weave through the fabric of our daily lives and propel numerous advancements across various sectors. Understanding how mechanical principles manifest in different domains reveals not only their utility but also their transformative potential. From engineering marvels to the intricacies of our physical bodies, mechanics plays a crucial role. This section elucidates its applications, covering key areas where mechanics is not just relevant but essential.
Mechanical Engineering
Mechanical engineering, a pillar of the engineering disciplines, relies heavily on the principles of mechanics to design and innovate. The blend of creativity and technicality helps engineers solve real-world problemsโbe it through developing robust machinery or optimizing complex systems. Notably, mechanical engineering integrates several sub-disciplines like mechanics of materials, dynamics, and thermodynamics, which are foundational in crafting anything from bridges to complex automotive systems.
The use of computer-aided design (CAD) software exemplifies how mechanics is harnessed in modern engineering. Designers use CAD to simulate physical systems, enabling a deep analysis of stresses and limits under various conditions. Advantages include, but are not limited to:
- Cost Efficiency: Mechanics-based simulations can cut expenses by reducing the waste of resources in prototyping.
- Safety Enhancement: Engineers can forecast potential failures, thus prioritizing safety in the designs.
- Innovation Growth: Mechanics inspires innovative designs and modifications that cater more effectively to user needs.
Biomechanics
In the realm of biomechanics, mechanics examines biological systems and how forces impact human movement and functionality. This field sheds light on how our physiological structure interacts with mechanics, which is vital in sports science, rehabilitation, and ergonomics. For example, analyzing the gait cycle (the way we walk) involves understanding forces acting upon bodies, which can guide rehabilitation processes.
Understanding joint mechanics aids in designing prosthetics that mimic natural movements. Key elements in biomechanics include:
- Kinematics: The study of motion without concern for forces. Itโs crucial for understanding how bodies move in different sports environments.
- Kinetics: This delves into forces and their effects on human bodies. Knowing how forces affect performance can improve training methods.
"Biomechanics bridges the gap between science and application, focusing on optimizing performance by understanding mechanical functions within anatomy."
Astrophysics and Mechanics
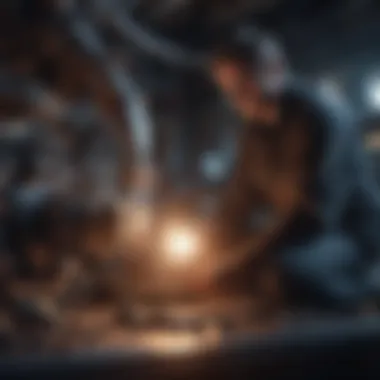
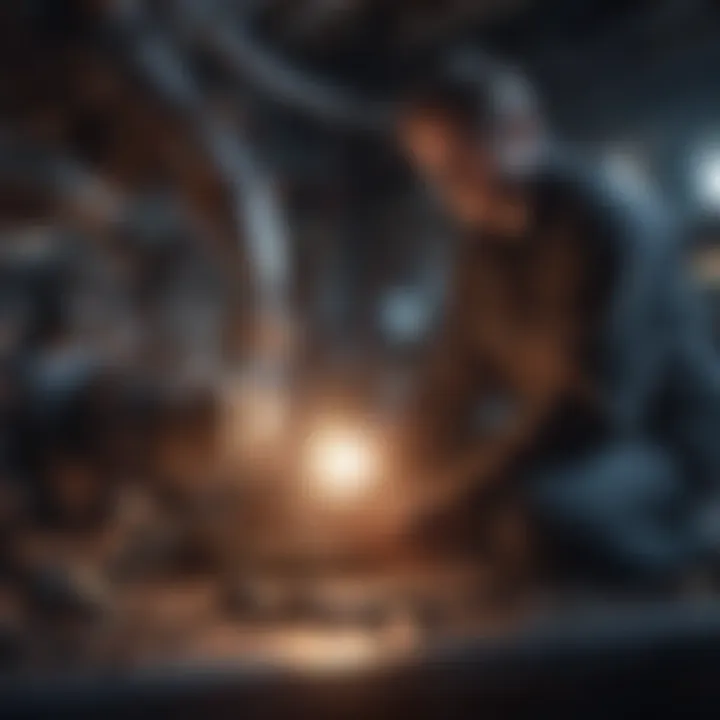
When mechanics strides into the vastness of space, we find an entirely different playground. Astrophysics, by its nature, relies on mechanics to elucidate celestial bodies and phenomena. From gravitational interactions to the motion of galaxies, the principles of mechanics are integral in understanding the universe.
For instance, Kepler's laws of planetary motion, which explain how planets move in elliptical orbits, are grounded in classical mechanics. This field contributes to:
- Space Missions: Mechanics informs trajectory calculations for spacecraft, ensuring successful launches and reentries.
- Stellar Dynamics: Mechanics helps decipher the movements and interactions of stars in clusters.
Environmental Mechanics
The intersection of mechanics and the environment offers insights essential for managing natural resources and mitigating climate change impacts. Methods from fluid mechanics and material analysis help in designing solutions to combat environmental challenges.
Key applications include:
- Hydraulic Engineering: Here, fluid mechanics principles guide the design of infrastructure such as dams and levees, crucial in flood control efforts.
- Renewable Resources: Understanding mechanical systems in the context of wind turbines or solar energy systems advances sustainable energy solutions.
In each of these domains, mechanics not only supports innovation and efficiency but also underlines the interconnectedness of technology and the challenges we face as a society.
Mechanics in Everyday Technologies
Mechanics pervades everyday technologies, weaving through various modalities that enrich our lives and enhance our practical capabilities. It's the backbone of countless innovations we often take for granted, from the simplest tools to sophisticated machines. Understanding mechanics is not just an academic pursuit; it has real-world implications that impact efficiency, safety, and sustainability in our day-to-day experiences.
Every technology, whether it's a pocket calculator or a high-speed train, relies on fundamental mechanical principles. These principles govern motion, energy transfer, and forces at play in each scenario. As a result, mastering the art of mechanics can lead to more effective design processes, smarter troubleshooting methods, and a deeper appreciation of technologyโs role in society.
Key Elements of Mechanics in Everyday Technologies
- Efficiency: Mechanics ensures that machines work at their optimal capacity. For instance, when designing a vehicle, engineers must consider aerodynamics to minimize drag, enhancing fuel efficiency.
- Safety: The effectiveness of safety systems in vehicles, such as airbags and seatbelts, springs from mechanical principles, which protect passengers during abrupt stops or collisions.
- Interdisciplinary Impact: Mechanical systems are not limited to one domain. For example, the principles of mechanics influence innovations in robotics and biomedical devices, linking multiple disciplines.
In summary, understanding the mechanical underpinnings of everyday technologies is crucial. It equips learners and professionals with the tools needed to drive innovation and improve existing systems. The following subsections delve into two significant facets of how mechanics shapes daily life: transportation systems and robotics.
Transportation Systems
Transportation systems exemplify the practical application of mechanical principles. From the design of roads to the functionality of vehicles, mechanics plays a pivotal role. When we look at public transport, for instance, trains and buses utilize mechanics to navigate difficult terrains, ensuring timely arrivals.
Additionally, innovations such as electric and hybrid vehicles bring mechanics into sharper focus. These cars incorporate advanced mechanical designs that enhance energy efficiency and reduce emissions. It's not just about getting from point A to point B; it's about doing so in a manner that is environmentally conscious and mindful of resource consumption.
Robotics and Automation
Principles of mechanics serve as the foundation for robotics and automation technologies. Robots mimic complex movements and perform tasks that humans either cannot or would prefer not to do. This ranges from simple household tasks like vacuuming to intricate operations in manufacturing plants. Each motion must be meticulously planned, leveraging knowledge of mechanics to ensure precision and functionality.
Moreover, automation spans various organizations, leading to increased productivity and reduced costs. Understanding how mechanics applies to these high-tech machines can unearth new opportunities for innovation and efficiency. As industries lean toward automation, a solid grasp of mechanical principles become more pivotal than ever before.
Challenges in Mechanical Applications
In the realm of mechanics, understanding the challenges faced during its practical application is paramount. These challenges not only influence the performance of mechanical systems but also dictate the advancement of technology across various domains. By dissecting these hurdles, a clearer perspective emerges, allowing students, researchers, and professionals alike to navigate these complexities with more informed strategies.
Some of the key challenges in mechanical applications include material limitations and energy efficiency issues. Grasping the essence of these topics sheds light on the broader implications of mechanics in engineering and technology. These aren't merely obstacles; they are pivotal points that drive innovation and evolution in the field.
Material Limitations
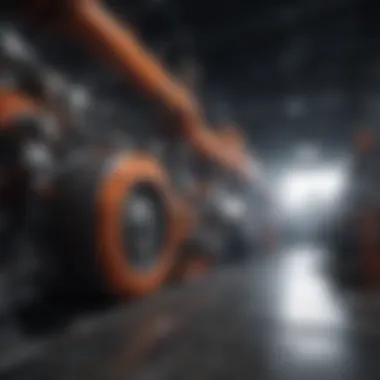
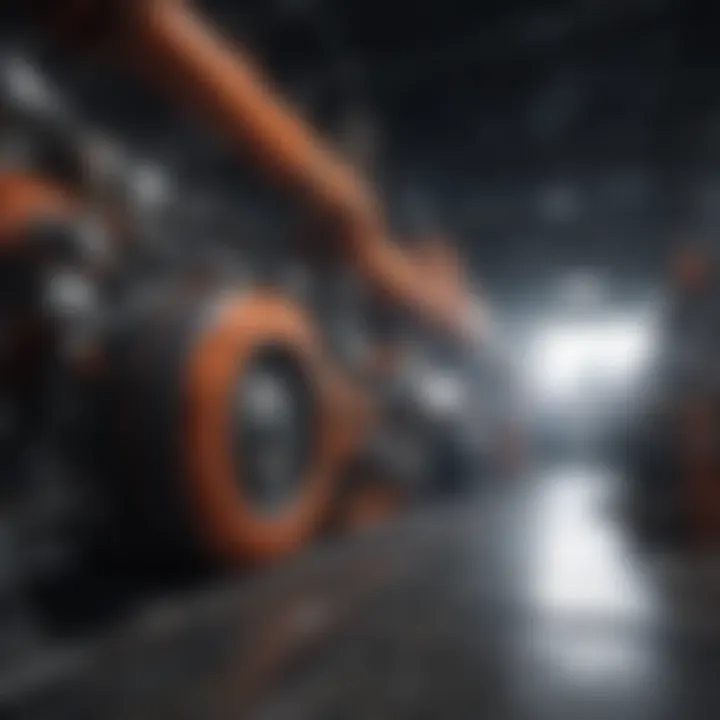
Mechanical systems are products of materials, each with their own characteristics, advantages, and limitations. The journey of a mechanical engineer begins here; the selection of materials can make or break a project, impacting durability, weight, and performance. For instance, consider the use of carbon fiber in aerospace applications. Its lightweight and high strength make it ideal, yet it comes with exorbitant costs and fabrication difficulties. Alongside this, typical materials like steel and aluminum face their own restrictions in terms of fatigue resistance and weight.
- Strength vs. Weight: Engineers are often tasked with achieving the right blend of strength and lightness. This compromise can lead to designs that either over-engineer or under-engineer a solution.
- Thermal Limits: Materials can behave differently under varying temperatures. For example, some polymers may lose rigidity when heated, limiting their use in high-stress applications.
- Corrosion and Wear: Selecting materials resistant to wear and environmental degradation is crucial for longevity, particularly in machinery that operates in rigorous conditions.
Moreover, advancements in material science can alleviate some of these issues, but they also come with their own learning curves. As the technology escalates, so does the difficulty in keeping pace with new materials entering the market.
Energy Efficiency
Energy efficiency is another challenge that has garnered increasing attention in mechanics. It's not merely about making a machine run; itโs about ensuring it runs optimally in the long run. Inefficient designs can lead to increased operational costs and environmental impacts, not to mention the technological setbacks that come from inadequate energy use.
Consider the debate about internal combustion engines versus electric motors. While internal combustion engines have dominated for decades, their findings present substantial inefficiencies compared to electric alternatives. The real challenge lies in making both systems better.
- Maximizing Output: Engineers constantly seek innovative ways to enhance the output-to-input energy ratio. This quest directly influences the viability of technologies in a competitive landscape.
- Sustainability Considerations: With the world leaning more towards sustainable practices, integrating energy-efficient solutions in mechanical design is no longer optional. Designers are challenged to think outside the box and reduce waste without compromising performance.
- Technological Integration: Emerging technologies, like smart sensors and IoT devices, offer ways to monitor and improve energy consumption but come with complexity and added costs.
"The challenge of mechanics isn't merely about resolving immediate problems; it's about anticipating future hurdles and refining our approaches to material and energy usage in real-world applications."
The Future of Mechanics
In considering the future of mechanics, one finds it akin to standing at a crossroads where tradition meets innovation. The vital role that mechanics plays in shaping technologies across various fields cannot be overstated. As we progress deeper into the 21st century, several factors beckon a transformation in how mechanical principles are approached and utilized. From greener energy solutions to nanoscale engineering, the next wave of developments will likely redefine our very understanding of mechanics.
Emerging Trends in Mechanics Research
As new challenges emerge, the realm of mechanics is constantly evolving. Several trends are presently making waves:
- Sustainable Engineering Practices: With climate change looming large, sustainable practices in mechanics are gaining traction. Research in this area seeks to minimize environmental impact while maximizing efficiency. Engineers are focusing on eco-friendly materials and processes that consume less energy and produce less waste.
- Nanomechanics: The exploration of mechanical properties at the nanoscale is opening doors that we previously thought were sealed. As it turns out, materials can behave unexpectedly when reduced to such tiny dimensions. This facet of mechanics has significant implications for improving materials in various industries, from electronics to medicine.
- Robust Computational Mechanics: The advent of cutting-edge computer simulations is revolutionizing the discipline. Today, complex mechanics problems that once took mountains of time to unravel can be simulated with remarkable accuracy in mere hours. This trend not only accelerates research but also allows engineers to test theories without the high costs associated with physical prototypes.
- Bio-inspired Mechanics: Nature often holds the answers to the most complex engineering problems. By studying how organisms workโbe it the elasticity of a spiderโs silk or the aerodynamics of a bird wingโmechanical engineering is leaning more towards biophilic design. This approach not only enhances performance but also promotes sustainability through the use of organic materials.
"Research trends indicate that the boundary between mechanical engineering and other scientific fields are becoming increasingly blurred, allowing for more integrated approaches to solving complex problems."
Interdisciplinary Approaches
One cannot ignore the growing necessity for interdisciplinary collaboration in the field of mechanics. This blending of knowledge from different domains enhances our approach to complex problems. Here โs how:
- Engineering and Biology: The marriage of mechanics with biological sciences is proving to be vital. Engineers are utilizing biological concepts to innovate, especially in medical applications like prosthetics and tissue engineering. This cross-pollination promotes solutions that are not just mechanically sound but biologically viable as well.
- Physics and Environmental Science: The intermingling of mechanics with physics is crucial for understanding phenomena that affect our environment. For instance, studies on fluid dynamics can aid in tackling pollution and climate issues, providing crucial data for policy-making on environmental protection.
- Robotics and Computer Science: The surge in robotics has shifted the focus of mechanics to accommodate a fusion of mechanical and computational disciplines. As robots become an integral part of daily life, the alignment between mechanics and artificial intelligence creates possibilities for smarter, more efficient machines.
As we look ahead, the integration of various disciplines with mechanics will not only enhance technological advances but could also lead to breakthroughs that we cannot currently foresee. Thus, the future of mechanics isnโt simply a continuation of the past; itโs a pathway toward complex, multifaceted solutions to the challenges of tomorrow.
End
The discussion on mechanics provides pivotal insights into various scientific and engineering disciplines. This conclusion aims to encapsulate the key points explored in the previous sections while highlighting their relevance within the broader context of modern research and applications.
Summary of Key Insights
- Interconnectivity of Mechanics: Mechanics is not confined to purely physical systems; it intersects with biology, technology, and environmental sciences. Understanding these relationships is crucial for multidisciplinary strategies in research and innovation.
- Real-World Applications: Practical implementations of mechanics in everyday technologies such as transportation systems and robotics reveal the significance of principles derived from this field. These applications underscore the necessity of integrating mechanics into educational curriculums at various levels.
- Challenges and Evolution: The discussion of existing challenges, like material limitations and energy efficiency, highlights the ongoing evolution of mechanics. Technologies must adapt to address these challenges while achieving greater sustainability and functionality.
Implications for Future Studies
The exploration of mechanics offers fertile ground for future inquiries in multiple domains:
- Emerging Technologies: As technologies develop, more sophisticated mechanics will be required to drive advancements. This suggests the necessity for sustained research in areas like nanotechnology and biophysics.
- Interdisciplinary Research: Future studies should prioritize interdisciplinary approaches that incorporate insights from various fields. Collaborative efforts between engineers, biologists, and environmental scientists could yield transformative solutions to pressing global issues.
- Sustainability Focus: Research exploring the environmental impacts of mechanical systems can create pathways for sustainable design principles, ensuring that technological progress does not compromise ecological balance.
"The future of mechanics hinges not just on understanding the principles, but on applying them in innovative and responsible ways."
In closing, it is evident that the multifaceted nature of mechanics shapes not only the future of science and technology but also societal advancement as a whole. By fostering a deep understanding of these principles, individuals and teams can harness their potential in meaningful and impactful ways. The journey through mechanics remains one of constant discovery, and its implications will only continue to grow in significance.