Holography Microscope: Innovations and Uses in Science
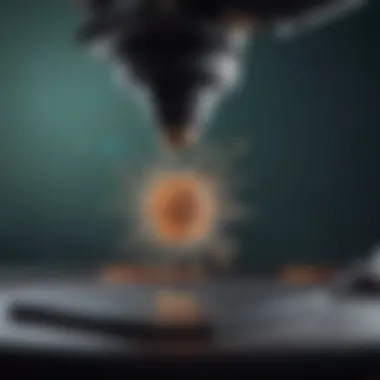
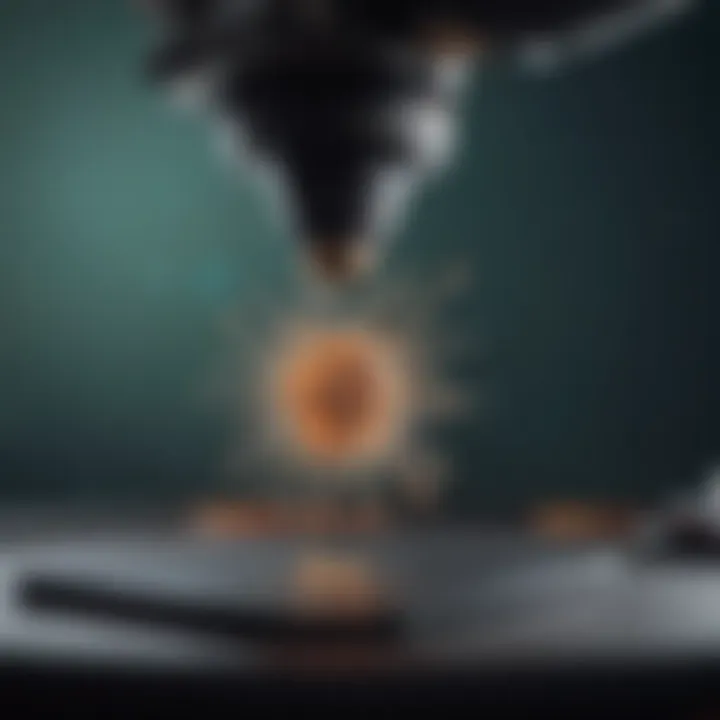
Intro
The realm of microscopy lies at the heart of understanding the microscopic world. Traditional microscopy methods, while invaluable, have certain limitations that often impede the observation of intricate details. This is where holography microscopy steps in, turning the tables on conventional techniques. With its unique capacity to visualize three-dimensional structures without the requirement for extensive sample preparation, holography microscopy has revealed a new frontier in various scientific domains.
Moreover, this technique employs laser light and computational algorithms, enhancing resolution and enabling researchers to gather detailed information about samples non-invasively. Whether in cellular biology, materials science, or even nanotechnology, holographic methods are garnering attention for their ability to obtain high-quality images and quantitative data with astounding capabilities.
As the field continues to evolve, understanding the advancements and applications of holography microscopy becomes crucial for students, researchers, and professionals seeking to leverage these technologies in their work. With that in mind, it’s pertinent to dissect the progress made in this field, the underlying principles guiding these innovations, and the multitude of applications it encompasses.
Foreword to Holography Microscopy
Holography microscopy represents a significant leap forward in the field of imaging and analysis. It has carved out a niche for itself as an innovative approach that offers detailed insights into microscopic structures. As the scientific community seeks enhanced ways to visualize and understand the intricacies of biological and material systems, holography microscopy stands out for its ability to capture three-dimensional information without the need for traditional staining or elaborate sample preparation.
In an era where precision and detail are paramount, this technique provides researchers with the tools to observe samples in their natural state, leading to more accurate analyses. Such advancements are crucial in fields like biology and materials science, where understanding three-dimensional configurations and dynamics can lead to breakthroughs in research and product development.
Throughout this article, the importance of holography microscopy will be elucidated, focusing on its foundational principles and diverse applications. The discussion will also encompass a comparison with conventional microscopy techniques, highlighting how holography redefines our capacity for visualization.
Definition and Historical Context
Holography, originating from the Greek words for "whole" and "writing," fundamentally refers to the technique of capturing light fields to form a three-dimensional image of an object. Initially developed in the mid-20th century by physicist Dennis Gabor, holography was primarily used for data storage and security purposes but quickly found its footing in scientific applications. By the 1960s, the advent of coherent laser light allowed for greater clarity and precision in recording holograms, setting in motion a series of innovations that would lead to the application of holography in microscopy.
As researchers began to explore the potential of holography in capturing fine details of microscopic samples, it became clear that this technology could extend beyond mere visualization. The ability to record light interference patterns made it possible to create images that not only exhibit spatial relationships but also capture dynamic processes occurring within living cells. Today, the evolution of holography microscopy continues to unfold, with advances in optics and computing propelling its capabilities.
Comparison with Conventional Microscopy Techniques
When contrasting holography microscopy with traditional microscopy methods, several distinct advantages emerge. Traditional techniques, such as light and electron microscopy, often rely on complex staining methods that can alter or destroy delicate specimens. The overarching goal in conventional microscopy is to enhance contrast and resolution, frequently at the expense of the sample's integrity.
In stark contrast, holography microscopy allows for high-resolution imaging without modification of the specimen. This non-invasive nature means that researchers can observe biological samples in real-time, unveiling the inner workings of cellular structures or processes like mitosis without disrupting their environment.
Here are some key differences:
- Imaging Technique: Traditional methods often require fixing and staining, while holography captures the light field as it interacts with the sample directly.
- Three-Dimensional Visualization: Unlike conventional techniques, holography provides a naturally three-dimensional view, enabling a fuller understanding of spatial relationships.
- Dynamic Monitoring: The capacity to monitor live samples over time is significantly enhanced in holography, which is invaluable in dynamic research settings.
In summary, while traditional microscopy techniques have their own merits, holography microscopy opens new avenues for research that emphasize preservation and real-time observation. As we delve further into the principles behind this technology, the implications for various scientific disciplines will become increasingly evident.
"The advantage of holography lies in its ability to uncover realities that remain hidden through conventional methods."
As we advance through the sections, we will explore the principles driving holography microscopy and examine its profound impact across multiple fields.
Principles of Holography
Understanding the principles of holography is essential in grasping how holography microscopy elevates our imaging capabilities beyond traditional microscopy. This section elucidates the core concepts that form the backbone of holography. The benefits of applying holographic techniques in various fields are immense, allowing for a more nuanced observation of samples without the need for complex sample preparation.
Basic Concepts of Holography
Holography is more than a fancy technique; it’s a paradigm shift in the way we capture and visualize light. At its heart, it revolves around the interference of light waves. When a coherent light source, such as a laser, strikes an object, it scatters in various directions. This scattered light can then be captured on a recording medium, typically photographic film. What marks the uniqueness of holography is that it records both the intensity and the phase of the light waves, allowing for a three-dimensional representation of the object when viewed.
One fundamental aspect of holography is the object beam and the reference beam. The object beam is the light reflected from the object, while the reference beam is not modified by the object at all. When these two beams meet, they create an interference pattern that encodes the information about the object’s shape and texture in an extremely detailed manner.
Acquisition of Holograms
The process of acquiring holograms is deceptively straightforward. It begins with selecting a coherent light source, which is crucial for obtaining clear and precise holograms. The setup usually involves a laser beam that is split into two paths: one directed towards the sample and the other toward a photo-sensitive surface.
Most importantly, the alignment of the beams is critical. Any misalignment can lead to distorted images. Holography microscopy often utilizes advanced techniques like digital holography, where digital sensors replace traditional films. This transition has made the acquisition process not just faster, but also more versatile. By utilizing digital sensors, researchers can capture dynamic processes in real-time, something traditional methods can hardly manage.
The ability to record objects' three-dimensional information in real-time is a revolutionary stride, setting holography apart from its conventional counterparts.
Reconstruction of Holographic Images
Reconstructing the images from the captured holograms is where the magic happens. This process can be visualized as shining a laser on the recorded hologram. The phase and amplitude information captured in the interference pattern guide the light to reconstruct the original object’s image. What's fascinating here is that this reconstruction doesn’t just yield a flat image; it allows for multi-dimensional viewing.
Holographic images can be viewed from various angles, giving a true sense of depth. This is invaluable, especially in biological studies and material science where understanding the intricate details on a 3D level can lead to new insights and discoveries. You could say that reconstructing holograms is akin to opening a window to a whole new world, where each glance reveals more than just what meets the eye.
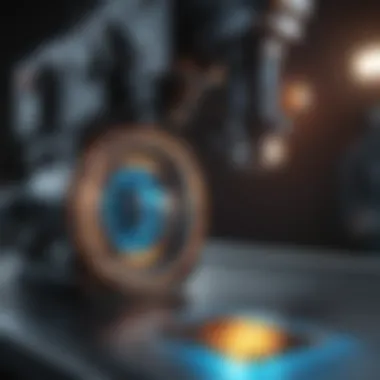
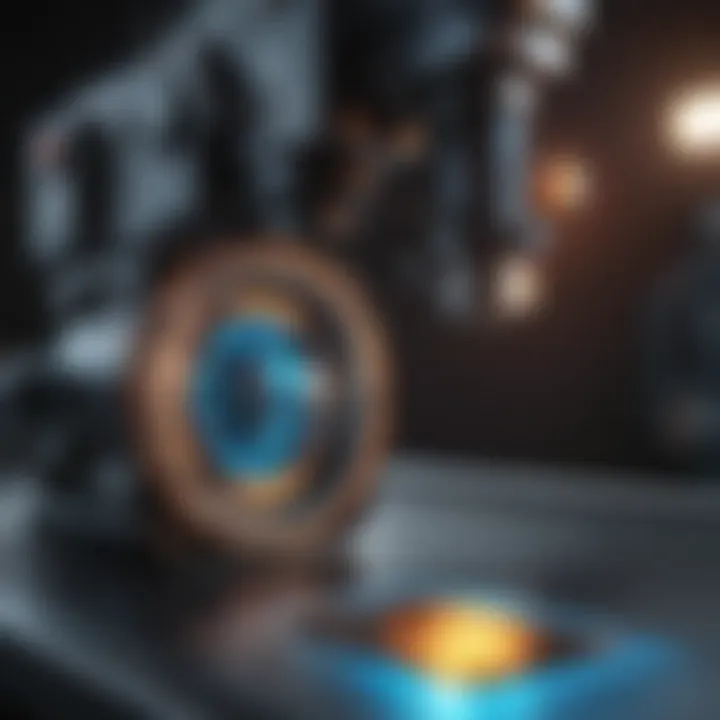
In summary, the principles of holography are not simply a collection of procedures but rather, they represent a transformative journey into advanced imaging. With a firm grasp on these concepts, we can appreciate how holography microscopy paves the way for unparalleled advancement in scientific research and practical applications.
Technology Behind Holography Microscopy
Understanding the technology behind holography microscopy offers a gateway to grasping how this technique elevates imaging capabilities in various scientific realms. At its core, the technology serves as a bridge that connects intricate optical components with sophisticated computational methods. This synergy enables researchers and professionals to delve deeper into their subjects of study, whether that be in the biological sciences, material science, or medical diagnostics. By leveraging advanced optical principles, holography microscopy doesn't just enhance visibility; it redefines the very essence of imaging methodologies.
Components of a Holography Microscope
Laser Sources
Laser sources are pivotal in holography microscopy due to their unique ability to produce coherent light. This coherence is crucial for effective interference patterns needed to generate holograms. A key characteristic of lasers is their monochromaticity, which means they emit light of a single wavelength. This distinct property makes lasers a popular choice among practitioners; it allows for precise measurements and clearer images.
A standout feature of lasers is their stability. Unlike traditional light sources, lasers maintain their intensity and wavelength over time. The advantages of using laser sources are manifold: they contribute to low noise levels in imaging and enhance the resolution of detailed structures. However, one must consider their high costs and the technical know-how needed for setup and operation.
Wavefront Sensors
Wavefront sensors play an essential role in holography microscopy, specifically in measuring the phase perturbations in light waves as they interact with microscopic samples. These sensors can detect even minimal changes in the light’s wavefront, enabling the creation of high-fidelity holograms. A significant feature is their speed; they provide real-time data on wavefront distortions. This characteristic is invaluable for dynamic monitoring of samples under observation.
Wavefront sensors are favored because they allow for non-invasive imaging techniques. A benefit of using these sensors is their ability to reveal details about nanostructures that may go unnoticed with conventional methods. However, a disadvantage is that they require calibration and can sometimes be sensitive to environmental factors, which may influence accuracy.
Optical Detectors
Optical detectors are the eyes of the holography microscope, responsible for capturing the light information transformed by the holographic process. These detectors convert light signals into electrical signals for analysis. A key aspect that makes optical detectors stand out is their sensitivity. Different types of detectors, such as CCDs and CMOS sensors, offer various benefits and trade-offs, making selection critical.
The unique feature of optical detectors is their adaptability to diverse imaging conditions. They are highly efficient for low-light environments, which is often where holography thrives. But there are caveats; sensor noise and dynamic range can pose challenges which sometimes limit the scope of imaging in certain conditions.
Software for Image Processing
The advancement of software for image processing has been a game changer for holography microscopy. This software handles the massive amounts of data captured during imaging processes, employing algorithms to enhance and reconstruct images. The analytical capabilities of such software allow researchers to visualize complex structures in unprecedented detail.
By integrating artificial intelligence and machine learning, image processing software continuously learns from the data, refining its ability to deliver sharper and more relevant images. The importance of this software in processing complex holographic data cannot be overstated; it allows for real-time analysis that can significantly accelerate research timelines. Moreover, its user-friendly interfaces enable even those with limited technical expertise to harness the power of holography microscopy effectively.
Applications of Holography Microscopy
The realm of holography microscopy has burgeoned, illuminating various scientific fields with its unique imaging capabilities. Applications of this advanced microscopy technique are wide-ranging, but they tend to be particularly significant in biological sciences, material science, and medical diagnostics. Each field reaps distinct benefits, enabling researchers and professionals to delve deeper into their respective studies. This section aims to highlight those applications, emphasizing the key characteristics and unique features that make holography microscopy a powerful tool in modern research and development.
Biological Sciences
Cell Imaging
Cell imaging stands as a cornerstone in the exploration of biological organisms. The primary aspect of cell imaging is its ability to provide clear and non-invasive images of live cells, enabling scientists to observe cellular processes in real time. This is crucial, as it permits the study of dynamic movements and interactions within cells, such as mitosis or cellular response to stimuli.
One key characteristic of cell imaging via holography is its high axial resolution; it captures fine details without the need for traditional staining techniques, which can alter cellular structure.
- Benefits of Cell Imaging:
- Non-destructive to samples, promoting natural cellular behavior.
- High resolution allows for microparticles detection within cells.
However, the technique is not without its challenges. The interpretation of holographic images may require advanced processing technology to convert raw data into meaningful visual representations.
Understanding Cellular Structures
The understanding of cellular structures takes this a step further; it focuses not only on visualizing but also elucidating the organization and function of organelles within cells. This addition of depth allows scientists to analyze cellular abnormalities, contributing to major advancements in fields like cancer research. The ability to differentiate between various organelles, such as mitochondria or endoplasmic reticulum, highlights why this aspect is pivotal in this article.
- Key Features:
- Offers insight into the spatial configuration of cellular components.
- Enhanced ability to determine cell health by observing organelle integrity.
Yet, the disadvantage lies in the sometimes steep learning curve to effectively utilize the software required for accurate image reconstruction and analysis.
Material Science
Surface Analysis
Holography microscopy brings powerful surface analysis capabilities, allowing scientists to determine material properties without lifting a finger. By examining the surfaces of materials at nano-scale resolution, researchers can unlock secrets about roughness, composition, and defects. The key characteristic of this approach is that it provides instantaneous feedback, making surface analysis a rapid process.
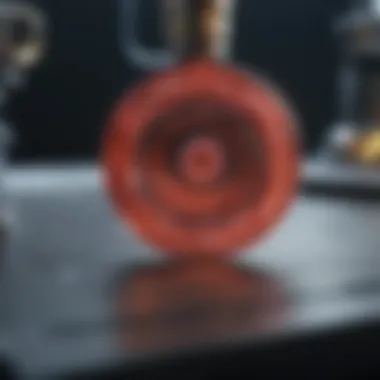
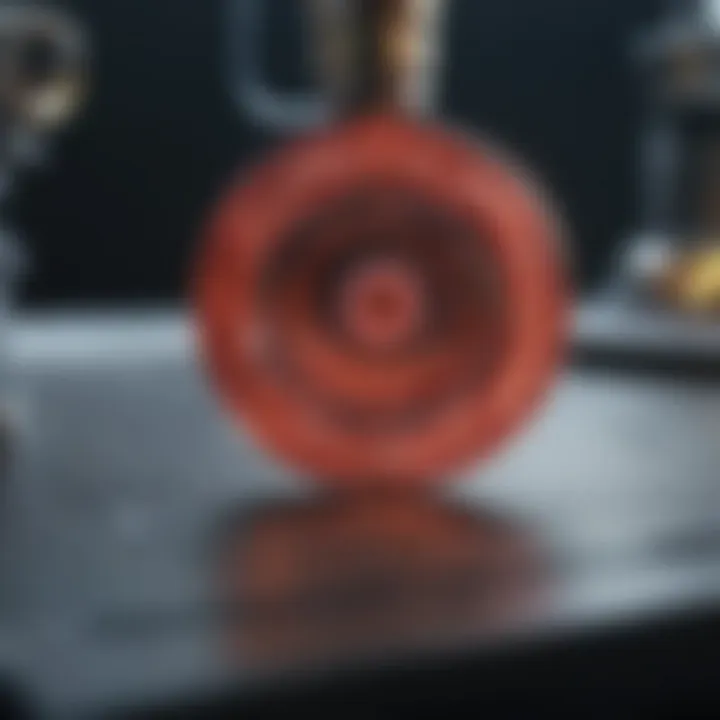
- Benefits of Surface Analysis:
- Non-invasive and quick analysis of various material types.
- Real-time assessment aids in quality control processes.
However, the precision of this method can be influenced by environmental factors like temperature fluctuations, potentially skewing results.
Nanostructure Investigation
Diving into nanostructure investigation brings new insights about materials at the atomic level. This aspect sheds light on the interplay between nanostructures and their macroscopic properties, paving the way for advancements in nanotechnology and optoelectronics. To that end, the key feature of nanostructure investigation is its capability to measure alterations in refractive indices, facilitating the monitoring of structural changes over time.
- Highlights of Nanostructure Investigation:
- Crucial for developing new materials with tailored properties.
- Provides pivotal data for the improvement of nano-engineered structures.
Despite the advantages, the complexity of fabricating and interpreting nanostructures often presents barriers to widespread application.
Medical Diagnostics
In the fast-paced world of medical diagnostics, holography microscopy is carving out a niche for itself. This technique's ability to analyze biological samples swiftly and accurately can offer timely insights. The ultimate goal here is improving patient outcomes through precise testing and monitoring.
Holography’s non-invasive approach ensures that samples are preserved while still delivering crucial information about cellular anomalies, such as abnormalities indicative of diseases. This swift, efficient method is a breath of fresh air compared to the more traditional, lengthy procedures that often delay diagnosis.
In summation, the applications of holography microscopy span across various disciplines, offering unique advantages and pushing the boundaries of what we understand about biological and material sciences, while enhancing the diagnostic strategies within healthcare. As it continues to evolve, we are likely to witness many more groundbreaking developments fueled by these advanced imaging techniques.
Impact of Holography on Current Research
Holography has made waves across various research domains, reshaping the landscape of how scientists visualize and understand intricate structures and phenomena. This shift towards holography, with its ability to capture three-dimensional volume data, marks a significant departure from traditional imaging techniques. Its impact can be divided into two key areas: enhancements in imaging capabilities and the exploration of new scientific frontiers.
In today's fast-paced research environment, the need for clear, high-resolution images is paramount. Holographic techniques cater to this necessity, unveiling details previously obscured. This not only aids in better comprehension of material properties but also enhances the discovery of known and unknown structures.
Enhancements in Imaging Capabilities
Holography microscopy presents a transformative approach to imaging, elevating traditional methods to an entirely new level. By employing coherent light, such as that from lasers, this technique enables researchers to visualize structures in more detail than ever before. The ability to create a holographic image means that scientists can study samples without the need for staining or alteration, preserving their natural state.
- High Spatial Resolution:
- Dynamic Imaging:
- Three-Dimensional Visualization:
- Holography delivers intricate details with remarkable clarity, essential in fields like cell biology and material science. Researchers can discern features on a nanoscale, which can alter their understanding of cellular processes or material behaviors.
- Unlike conventional microscopy, which often captures static images, holography can capture the movement and changes within specimens over time. This dynamic imaging capability is invaluable, especially in studying live cells or reactions in materials.
- Holographic images are not just two-dimensional projections; they can depict a three-dimensional view of the specimen. This depth perception allows for more comprehensive analysis compared to flat images.
"Holography holds the potential not just to visualize but to revolutionize the very core of scientific investigations."
Thus, the enhancements provided by holographic methods are not merely incremental but rather a seismic shift that aids researchers in achieving new discoveries and innovations in their fields.
New Frontiers in Scientific Inquiry
The integration of holography into research is also paving pathways to new scientific inquiries. It acts as a catalyst for exploration, prompting questions and investigations that were previously deemed challenging or even impossible. This technology encourages a more interdisciplinary approach to research, weaving together principles from physics, biology, and engineering to create a more holistic understanding of scientific phenomena.
- Exploration of Complex Biological Systems:
- Insights into Material Properties:
- Fusion with Emerging Technologies:
- Holography facilitates the study of complex interactions within biological systems in a more integrated manner. It allows scientists to observe how cells interact with their environment or how they respond to stimuli without any disruptive influence from traditional imaging methods.
- The ability to analyze materials at different scales opens doors to innovative applications in fields such as nanotechnology and metrology. Understanding how materials behave under various conditions can lead to substantial advancements in product design and material engineering.
- By amalgamating holography with emerging technologies like AI and machine learning, researchers can develop more refined analytical tools that enhance predictive modeling and characterization of complex systems.
In summary, the impact of holography on current research is profound. It provides enhancements in imaging capabilities that improve how scientists visualize the microscopic world while also pushing the boundaries of traditional inquiry. This evolution in microscopy not only augments scientific understanding but also inspires novel approaches to problem-solving across different domains.
Challenges and Limitations
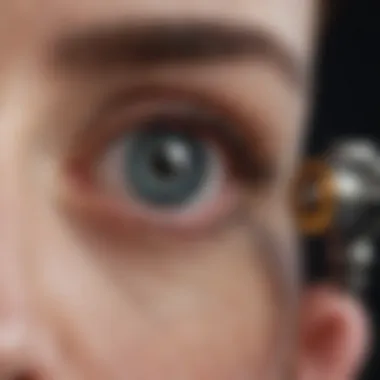
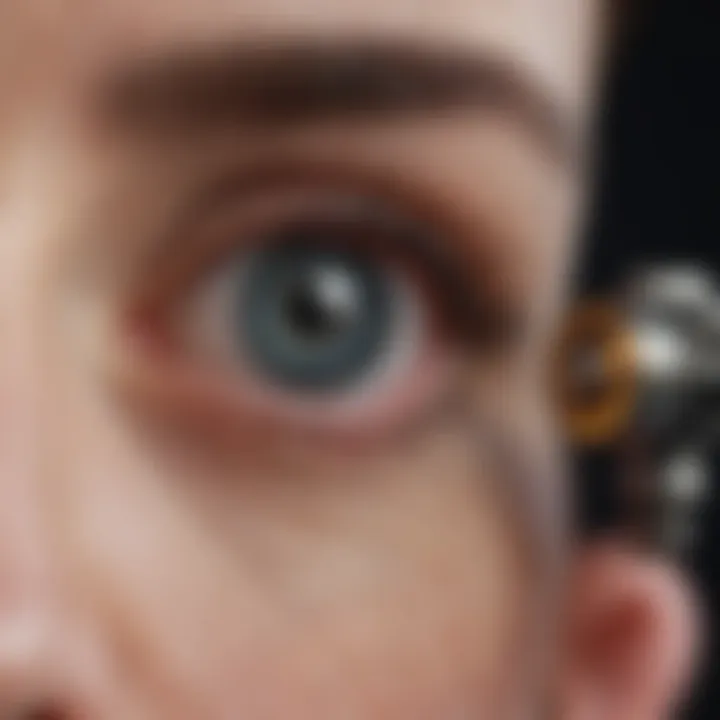
Understanding the challenges and limitations of holography microscopy is crucial for grasping the overall narrative of its emergence and ongoing evolution in scientific inquiry. While this advanced imaging technology carries with it a host of benefits, such as improved imaging capabilities and enhanced analytical precision, it is not without its drawbacks. Delving into these challenges provides a more nuanced view for students, researchers, educators, and industry professionals who seek to leverage holography microscopy in their work.
Technical Hurdles
One of the most pressing technical hurdles faced in the realm of holography microscopy involves the need for specialized equipment. Unlike traditional microscopes that rely on simple optical components, holography systems require sophisticated setups that can include high-quality lasers, sensitive sensors, and advanced image processing software. These components must work in unison to ensure that the holographic images are accurate and of high resolution.
For instance, the reliance on lasers for illumination means that any imperfections in the laser source can lead to distortions in the captured holograms. Furthermore, fluctuations in environmental conditions such as temperature and vibrations can impact the stability of the holographic setup, thereby introducing noise into the measurements. Researchers must frequently recalibrate their systems to account for these inconsistencies, which can be both time-consuming and resource-intensive.
It's also worth noting that holographic reconstruction, the process by which 3D images are formed from holograms, can be computationally demanding. The techniques used for reconstruction may require significant processing power, posing challenges particularly for those working in field settings or with limited access to high-performance computing resources.
Cost and Accessibility Issues
Cost and accessibility issues represent another significant barrier to the widespread adoption of holography microscopy. The initial investment in holography equipment can range from tens of thousands to millions of dollars, depending on the complexity of the system. This steep price tag limits access primarily to well-funded research institutions and commercial enterprises, leaving smaller labs and emerging researchers at a disadvantage.
While prices may gradually decrease as technology progresses and becomes more commonplace, the associated costs of ongoing maintenance and specialized training also cannot be overlooked. Technical specialists adept at operating and maintaining holography systems may not be readily available, thus further complicating the adoption process.
Moreover, the learning curve associated with holography microscopy can also deter researchers from venturing into its use. Many scientists are accustomed to traditional microscopy techniques and may resist shifting their methodologies, fearing not only the complexity of holography but also its perceived lack of proven reliability in comparison to established methods.
In summary, while the promise of holography microscopy is significant for advancing our understanding of biological and material sciences, it is essential to address the various challenges and limitations that accompany this technology. By tackling these hurdles head-on, the scientific community can work towards making holography microscopy more accessible and efficient, ultimately unlocking new avenues for research and discovery.
Future Directions in Holography Microscopy
The journey of holography microscopy is far from over. As we stand on the threshold of unprecedented advancements, the future beckons with a promise of transformative innovations and applications. The field is not only about refining existing techniques but also about exploring uncharted territories where holography can be applied to a myriad of disciplines. The potential for growth lies in embracing new technologies, expanding its reach beyond conventional realms, and leveraging interdisciplinary approaches.
A major focus in this future landscape will be on access and affordability, as breakthroughs in technology often come with a suite of hurdles including cost. The accessibility of such cutting-edge technologies must be prioritized to empower researchers and practitioners alike, democratizing access to these powerful tools for scientific exploration.
Innovations on the Horizon
As the technology behind holography microscopy continues to evolve, we can expect several innovations to emerge. New laser sources and camera technologies are being developed, permitting deeper imaging capabilities and higher resolution than ever before. Miniaturization of tools will also play a huge role, enabling portable holographic microscopes that can be used in a more ad-hoc or field-based context. Being able to visualize samples in real-time with high fidelity in non-laboratory settings could open new avenues for research, particularly in environmental biology or remote medical diagnostics.
Moreover, improvements in digital holographic techniques promise enhanced image reconstruction algorithms that will allow faster and more accurate processing of holographic images. This may lead to more immediate applications in areas such as live-cell imaging, where observing dynamic processes in real-time is crucial.
Integration with Other Technologies
The integration of holography microscopy with other technologies is another promising frontier. This coupled approach could result in synergetic systems capable of providing richer, more comprehensive data in various research contexts.
AI and Machine Learning
One of the most exciting aspects is the involvement of AI and machine learning. These technologies can automate the image analysis process—a task that can be time-consuming and complex when done manually. Key characteristics of AI, particularly its ability to learn from large datasets, make it a powerful ally for refining imaging techniques.
By employing algorithms trained on diverse datasets, AI can identify patterns and anomalies in the holographic data that may be imperceptible to human eyes. This brings significant benefits: it increases the efficiency of data processing and potentially enhances the accuracy of findings. A unique feature here is the adaptability of AI models which can be tailored to specific research needs, but there are some drawbacks too. The black-box nature of some AI models can make it hard for researchers to understand their decision-making process, raising questions about transparency.
Multi-Modal Imaging Systems
Alongside AI, the use of multi-modal imaging systems will gain traction. These systems combine holography with techniques like fluorescence, or electron microscopy, providing a more holistic view of sample specimens. The key characteristic of these systems is their ability to leverage the strengths of different imaging modalities to overcome the limitations of each individually.
For instance, while conventional fluorescence offers high specificity, it may lack in-depth structural details. By integrating holographic imaging, researchers may visualize cellular structures in three dimensions while also gaining functional insights through fluorescence. However, developing these integrated systems may be complex and could lead to higher maintenance demands. Furthermore, researchers must be trained to effectively operate and interpret the results from multifaceted systems—suggesting a prerequisite for extensive cross-domain knowledge.
In summary, the future of holography microscopy is rife with potential as innovations and integrations with cutting-edge technologies unfold. The spotlight will not only be on enhancing imaging capabilities but also on pushing the boundaries of scientific inquiry to heights yet imagined.
End
The role of holography microscopy in modern scientific exploration can't be overstated. This cutting-edge technology bridges the gap between traditional microscopy methods and the need for more refined, accurate imaging techniques. By embracing holography, researchers can achieve deeper insights into complex biological and material systems, pushing the boundaries of what we know about the microscopic world.
Recapitulation of Key Insights
In our journey through the article, we’ve discussed several pivotal points:
- Innovative Techniques: Holography microscopy harnesses the unique properties of light and wavefronts, allowing for high-resolution imaging without the fiddliness of traditional lenses.
- Diverse Applications: From the realm of biological sciences, where it aids in cell imaging and understanding cellular structures, to material science, where it plays a crucial role in surface analysis and nanostructure investigations, its versatility stands out.
- Technological Advances: The components of holography microscopes, like laser sources and optical detectors, along with sophisticated software, have evolved significantly, offering sharper and more detailed images.
- Integration with Modern Tech: The future lies in the integration of holography with technologies such as AI and machine learning, indicating a path toward even more innovative approaches to imaging.
Final Thoughts on the Role of Holography Microscopy
Holography microscopy is not merely a trend; it is a paradigm shift in how scientists approach imaging. One can’t help but appreciate its potential, especially considering how these capabilities can resolve long-standing challenges in both research and diagnostics.
As we look ahead, the importance of these advancements cannot be ignored. They equip researchers and clinicians alike with tools that enhance understanding and efficacy in diagnosis. With ongoing evolution, holography might just become the gold standard in microscopy, leading to breakthroughs that will change our perception of reality at the microscopic level.
This tech isn't just changing how we see; it's revolutionizing what we can discover.
Thus, holography microscopy stands at the intersection of art and science, offering a window into our world that continues to evolve and inspire.