GFP mRNA Transfection: Insights and Innovations
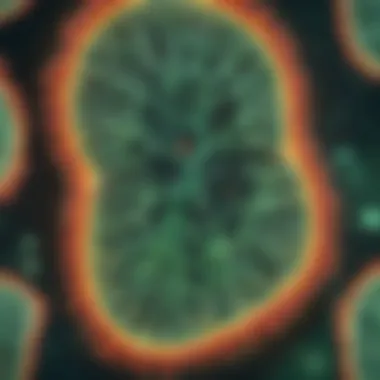
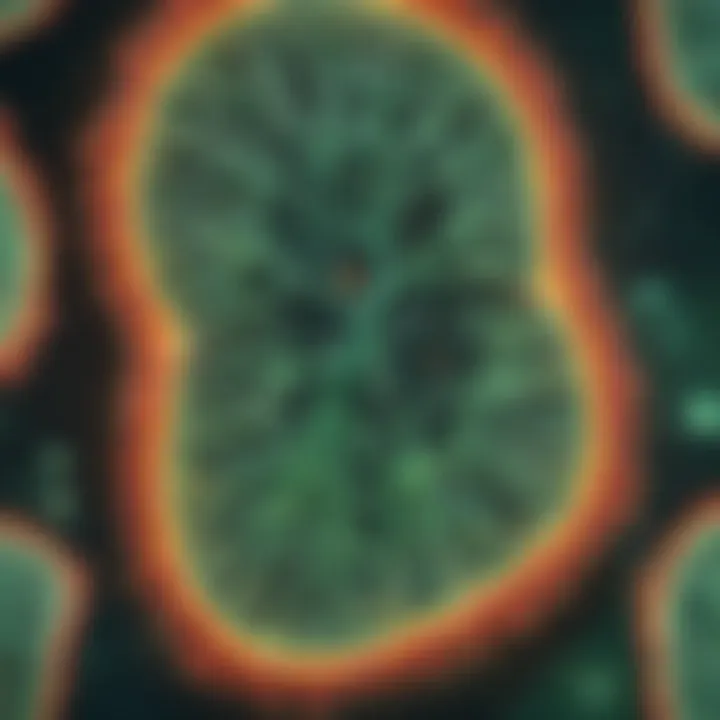
Intro
GFP mRNA transfection represents a pivotal method in modern molecular biology, bridging the gap between gene expression studies and real-world applications. By harnessing the power of messenger RNA, researchers can facilitate the production of Green Fluorescent Protein, a vital tool in various biological experiments. This process not only enhances understanding of cellular mechanisms but also paves the way for innovative therapeutic strategies. With a rapidly evolving landscape in transfection technology, educational platforms are essential in keeping students and researchers informed about the latest trends and breakthroughs.
Research Overview
Summary of Key Findings
The exploration of GFP mRNA transfection unveils multiple significant aspects:
- High Efficiency: Techniques have evolved to allow for superior delivery efficiencies, resulting in a more robust expression of the target gene.
- Versatile Applications: From basic research in cell biology to potential applications in gene therapy, GFP mRNA transfection plays a crucial role in many fields.
- Technical Challenges: Despite advancements, challenges remain—particularly concerning delivery systems, cellular uptake, and endosomal escape.
This combination of efficiency, versatility, and complexity characterizes contemporary research in this domain.
Research Objectives and Hypotheses
The key objectives of this investigation are:
- To elucidate the transcription and translation dynamics of mRNA once transfected into host cells.
- To analyze the challenges and limitations associated with current transfection methods.
- To explore novel transfection technologies and their potential impact on gene expression analysis and therapeutic interventions.
With these objectives in place, the research hypothesis posits that improvements in transfection techniques will significantly enhance both gene expression levels and therapeutic outcomes in clinical settings.
Methodology
Study Design and Approach
This research employs a comparative analysis of various transfection methods, including liposomal, electroporation, and viral-mediated delivery. A blend of experimental trials and computational models will outline the efficiency and effectiveness of each technique across different cell types. By maintaining a systematic approach, the study aims to dissect the mechanisms at play during the transfection process.
Data Collection Techniques
Data collection will involve:
- Quantitative Analysis: Measuring fluorometric outputs using specialized software to gauge GFP expression levels.
- Microscopy Imaging: Utilizing fluorescence microscopy to visualize transfected cells and assess the distribution of GFP.
- Molecular Assays: Conducting assays such as RT-qPCR to quantify mRNA levels post-transfection.
By integrating these diverse approaches, the study seeks to construct a comprehensive picture of GFP mRNA transfection mechanisms, paving the way for future innovations and improvements in both research and therapeutic contexts.
"Understanding transfection technology is no longer a luxury but a necessity for any serious endeavor in molecular biology."
Prologue to mRNA Transfection
mRNA transfection is a transformative process in molecular biology, providing researchers a tool to manipulate gene expression in cells. Understanding its nuances is essential for anyone involved in genetic research or therapeutic interventions. The implications of effectively delivering mRNA into cells can be monumental, influencing everything from basic research to advanced therapies.
The ability to introduce mRNA into a cell gives scientists a powerful way to appreciate the mechanics of gene expression and protein synthesis. This is particularly useful in studying diseases or creating models for drug testing. By shining a light on mRNA delivery techniques, we can better grasp the possibilities and limitations present in the field.
Defining mRNA Transfection
At its core, mRNA transfection can be described simply as the introduction of messenger RNA into a cell. Unlike DNA, which often integrates into a cell's genome, mRNA transfection allows for temporary expression of a protein. This brings a unique flexibility to research since the effects of gene expression can be analyzed without modifying the fundamental DNA blueprint.
So, how exactly does this work? When researchers transfect a cell with mRNA, it translates the RNA into a corresponding protein. This process holds many implications, particularly in studying gene function, protein interactions, or in drug development, allowing for continual adaptation of the biological inquiry.
Historical Perspectives on Transfection Techniques
The journey of transfection began in the realms of virology where scientists were first intrigued by how viruses could introduce their genetic material into host cells. The early techniques, such as calcium phosphate precipitation, were rudimentary yet groundbreaking, setting the stage for future advancements.
As the decades rolled on, techniques evolved significantly. Liposome-mediated transfection emerged as a more efficient method, offering a less toxic way to introduce nucleic acids into cells. These liposomes act like tiny delivery vehicles, surrounding the mRNA, making it easier for the cell membrane to facilitate entry.
Now, we find ourselves in an era where methods have reached a level of sophistication that allows for high-throughput screening, and targeted delivery systems. Recent innovations include electroporation and the use of viral vectors, which represent the latest frontiers in transfection technology.
Bringing history into the mix gives us perspective on how far we've come and where we are headed. It’s like tracing the footprints of scientific progress; each step reveals lessons learned and new paths laid before us.
With these advancements in hand, the stage is set to explore even more innovative solutions in mRNA transfection, with the hope of achieving unprecedented control over gene expression.
The Role of GFP in Molecular Biology
Green Fluorescent Protein, or GFP, plays a pivotal role in molecular biology, functioning not just as a simple marker but as a multifaceted tool in biological research. Its unique luminescence enables researchers to visualize cellular structures and processes in real-time, serving as an indicator of gene expression and protein localization. By incorporating GFP into experimental designs, scientists can garner insights that would be nearly impossible to achieve using traditional biochemical methods.
The significance of GFP is particularly pronounced in live-cell imaging. Unlike fixed cells, which can only provide a snapshot of cellular activity, live imaging with GFP allows for continuous monitoring. This has profound implications for understanding dynamic processes such as cell division, migration, and even signal transduction pathways. The ability to track these events in vivo adds a new dimension to our comprehension of cellular biology.
Additionally, the ease of genetic manipulation with GFP has made it a go-to tool for creating transgenic organisms. Whether it’s in mice for developmental biology studies or in plants for agricultural research, GFP-tagged genes offer researchers a way to visualize expression patterns across different developmental stages and environmental conditions.
Understanding the mechanisms by which GFP is integrated into molecular systems provides not only an appreciation of its utility but also highlights the considerations that accompany its use. The fluorescence spectrum of GFP can be influenced by environmental factors. Consequently, while GFP is robust, its performance can be variable, requiring researchers to take precautions to ensure consistent results.
"The incorporation of GFP into various experimental designs is not just a curiosity; it represents a leap towards more nuanced and comprehensive biological understanding."
Understanding Green Fluorescent Protein
GFP was originally discovered within the jellyfish Aequorea victoria, where it contributes to the organism's natural bioluminescence. The structure of GFP comprises a barrel-shaped chromophore that, when excited by specific wavelengths of light, emits a striking green fluorescence. This unique property has been harnessed in molecular biology, making it a versatile tool in both basic and applied sciences.
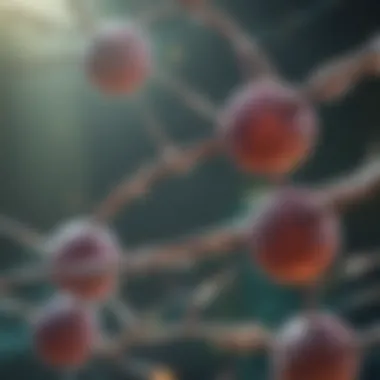
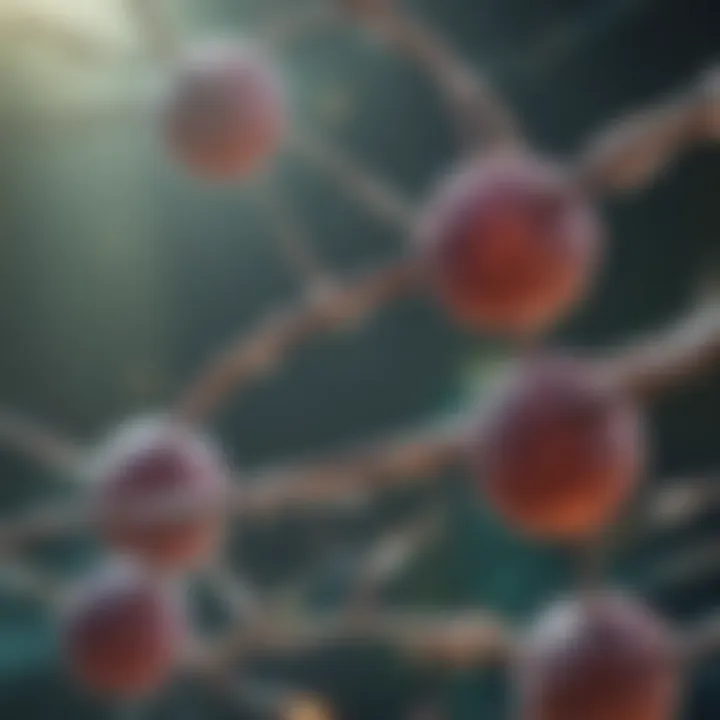
One notable aspect of GFP is its ability to function autonomously. Unlike some fluorescent tags that require conjugation with other molecules, GFP can be expressed directly from the DNA constructs. This simplicity facilitates its use in various experimental setups, reducing the likelihood of complications that may arise from tagging or conjugation processes.
Furthermore, advances in GFP technology have led to the development of enhanced variants. These newer versions, such as Enhanced Green Fluorescent Protein (eGFP) and mNeonGreen, feature improved brightness and photostability. Such enhancements are crucial for long-term imaging studies, where prolonged exposure to light can lead to photobleaching.
Applications of GFP in Research
The versatility of GFP has led to its incorporation in a wide array of research applications. One primary area is studying protein localization within cells. By tagging proteins of interest with GFP, scientists can observe where these proteins operate within the cellular architecture. This insight is invaluable; understanding the precise locations of proteins helps unravel their functions and interactions.
GFP is also a key player in gene expression studies. For example, scientists can use GFP reporter assays to assess promoter activity. By linking GFP expression to specific promoters, researchers can easily evaluate the regulatory mechanisms underlying gene activation. This method provides a visual and quantitative approach to monitor gene expression in real-time.
In additional fields, the use of GFP has extended into the realm of developmental biology. In studying organ development, scientists often utilize GFP to label specific cell populations. This allows for tracking the fate of these cells over time, shedding light on complex developmental processes.
In enlightening the future of medical research, GFP is increasingly employed in gene therapy investigations. Using GFP to validate successful gene delivery provides crucial insights into therapeutic effectiveness, offering hope for innovative treatment protocols targeting genetic disorders.
Overall, the contributions of GFP to molecular biology research are too significant to overlook. From unraveling the intricacies of cellular processes to revolutionizing methodologies in gene expression analysis, its versatility continues to empower scientific exploration in myriad directions.
Thus, any discussion about GFP mRNA transfection is incomplete without acknowledging the substantial implications of this remarkable protein in a multitude of research contexts. As techniques and applications evolve, so too will our understanding of the fundamental biological processes that GFP helps illuminate.
Mechanisms of mRNA Transfection
Understanding the mechanisms behind mRNA transfection is vital for several reasons. First, it helps unravel the intricate processes that facilitate the successful delivery of mRNA into target cells. This understanding underscores the efficiency of gene expression studies and the potential therapeutic impacts. More specifically, knowledge in this area points to the various cellular uptake pathways and how mRNA is translated into functional proteins, providing insights into optimizing transfection techniques across different cell types.
Cellular Uptake Mechanisms
The first step in mRNA transfection involves cellular uptake. Various mechanisms allow for the entry of mRNA into the cell, and these pathways can significantly influence transfection efficiency. A few main strategies come to mind:
- Endocytosis: This is when cells engulf extracellular material, forming vesicles. Different forms of endocytosis can occur, including clathrin-mediated and caveolin-mediated endocytosis, each with a unique role in cellular uptake.
- Direct Membrane Penetration: Under certain conditions, particularly when employing specific transfection agents, mRNA may cross the lipid bilayer directly. This method is less common but can be advantageous in some experimental setups.
- Microinjection: This technique involves the manual introduction of mRNA into cells using fine glass needles, ensuring high delivery accuracy. Though it’s not scalable for large numbers of cells, it provides excellent control over delivery.
For mRNA transfection to be effective, maintaining cellular health during these uptake processes is crucial. Cells are adept at sorting what they need, and thus understanding these pathways allows researchers to design strategies that enhance mRNA delivery without overwhelming cellular systems.
Translation of mRNA into Protein
Once the mRNA successfully penetrates the cellular structure, the next critical stage is translation into protein. Here’s a look at the core elements involved:
- Ribosome Interaction: After entering the cytoplasm, free ribosomes quickly recognize and bind to the mRNA. The ribosomes then initiate translation, synthesizing proteins based on the codon sequence encoded in the mRNA.
- Initiation Factors: Proteins that assist in several stages of translation are known as initiation factors. They help ribosomes find the start codon on the mRNA and ensure that the new polypeptide chain is created accurately.
- Post-Translational Modifications: Once synthesized, proteins may undergo various post-translational modifications that can alter their function, stability, or localization within the cell. This aspect ties back to the proper design of the mRNA, ensuring these modifications can take place effectively.
A clear view of these translation mechanisms emphasizes the importance of designing mRNA with both structural and sequence features that facilitate efficient protein synthesis. This interplay between delivery and expression showcases why both cellular uptake mechanisms and translation processes are crucial focuses in the realm of mRNA transfection.
Understanding the dynamics of mRNA uptake and translation equips researchers with the tools to enhance the effectiveness of gene therapies and other applications.
By grasping these multifaceted mechanisms, one can not only appreciate the complexity of mRNA transfection but also navigate the challenges it presents in both research and therapeutic contexts.
Techniques for Efficient mRNA Delivery
Delivering mRNA efficiently is crucial in ensuring that therapeutic and experimental outcomes meet desired goals. Not only does it enhance gene expression, but it also diminishes the energy wasted on unsuccessful transfection attempts. Efficient mRNA delivery methods can make or break a variety of research domains, especially in molecular biology where the mission is to understand cellular mechanisms and develop therapies. Here, we delve into three primary techniques that have gained traction in recent years:
- Liposome-Mediated Transfection
- Electroporation Methods
- Viral Vectors in mRNA Delivery
Each of these methods has its own set of benefits and considerations that researchers must navigate to optimize transfection efficiency.
Liposome-Mediated Transfection
Liposome-mediated transfection utilizes lipid-based vesicles to encapsulate mRNA, aiding its passage across cell membranes. The lipid bilayer of liposomes mimics cellular membranes, allowing for an easier fusion process.
- Benefits:
- Considerations:
- Biocompatibility: Liposomes are often well-tolerated by cells.
- Controlled Release: The encapsulated mRNA can be released in a controlled manner, helping maintain the desired concentration over time.
- Versatility: This method can be tailored for different cell types and experimental conditions, increasing its utility.
- Loading Capacity: Not all liposomes can carry large RNA molecules effectively.
- Stability: Liposomal formulations may experience instability during storage, leading to challenges in reproducibility.
Electroporation Methods
Electroporation has gained prominence in recent years. It involves applying an electric field to cells, making their membranes permeable to mRNA. By creating transient pores in the cell membrane, this technique allows for a high uptake of mRNA, making it effective for many cell types.
- How It Works: By applying a series of electric pulses, the procedure temporarily alters the permeability of the cell membrane. The mRNA can then enter without significant harm to the cell.
- Benefits:
- Considerations:
- High Efficiency: Typically yields higher transfection rates compared to other techniques.
- Scalability: Suitable for both small-scale experiments and large-cell populations; can be scaled up as required.
- Cell Viability: Care must be taken, as high voltages or poorly timed pulses can lead to cell death.
- Equipment Requirements: Specialized equipment is needed to generate the electric pulses, which could be a barrier for some labs.
Viral Vectors in mRNA Delivery
Viral vectors utilize modified viruses to deliver mRNA into host cells. This method takes advantage of the natural mechanisms that viruses use to infect cells, thereby ensuring effective entry and expression.
- Types of Viral Vectors:
- Benefits:
- Considerations:
- Lentiviral Vectors: Can integrate into host DNA, leading to long-term expression.
- Adenoviral Vectors: Provide high transfection efficiency but generally don’t integrate into the genome.
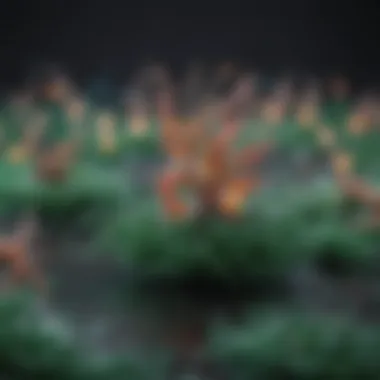
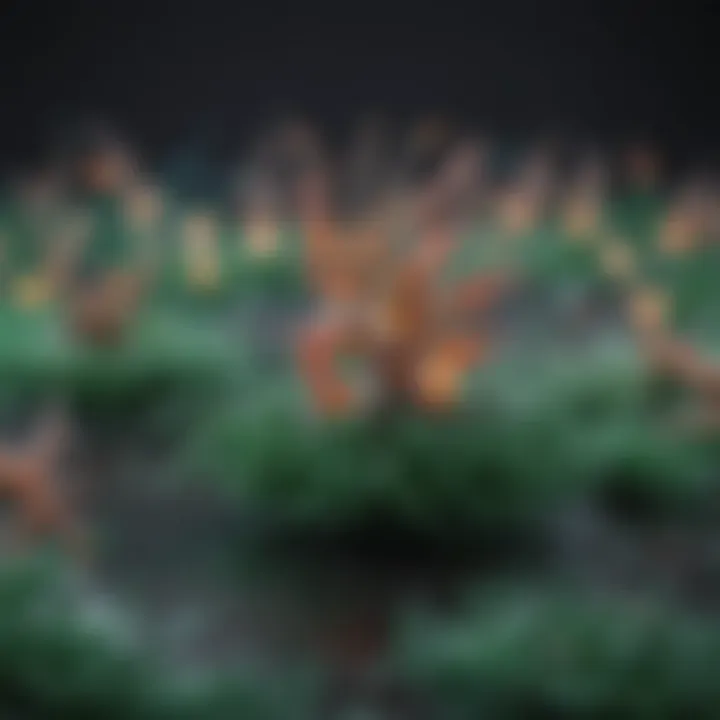
- Efficiency: Viral vectors can achieve high levels of transfection across a wide range of cell types.
- Stability: Once inside, the mRNA is often less susceptible to degradation compared to other methods.
- Immunogenicity: Potential immune responses can be triggered, which could limit the effectiveness.
- Ethical Concerns: Use of virus-derived methods may raise ethical questions and require careful handling due to biohazard considerations.
These mRNA delivery techniques offer various pathways towards enhancing transfection efficiency. The choice of method often depends on the specific requirements of the experiment, the cell type involved, and the desired outcome. Researchers must carefully weigh these factors to find a solution that fits their particular project.
"Selecting the appropriate technique for mRNA delivery is akin to choosing the right tool for a job; the right choice saves time and effort while optimizing results."
As exploration in the realm of molecular biology continues, these techniques will likely evolve further, opening doors to new discoveries and improvements in therapeutic contexts.
Factors Influencing Transfection Efficiency
Transfection efficiency is a critical element in the realm of molecular biology, particularly when it involves mRNA delivery. Understanding the factors that affect this efficiency can significantly enhance experimental outcomes. Whether it’s for research purposes or therapeutic applications, grasping how various elements interplay in transfection can lead to the development of more reliable and effective methodologies.
Cell Type Variability
When it comes to mRNA transfection, not all cells are created equal. The inherent variability among different cell types plays a huge role in how efficiently mRNA can be delivered and expressed. For example, epithelial cells often exhibit differing uptake mechanisms compared to neurons or stem cells. This variance raises the question: how does one optimize delivery for diverse cellular backgrounds?
- Uptake Efficiency: Cell membrane composition and receptor availability can drastically influence how much mRNA gets inside. Cells with a higher number of endocytic receptors may take up mRNA more readily compared to those with fewer receptors.
- Culture Conditions: Factors such as the density of the cells, the medium they are grown in, and even their passage number can affect transfection results. Optimizing these variables can lead to better uptake and expression of mRNA.
In certain cases, a generalized approach to transfection might not suffice. For researchers focusing on specific cell lines, tailoring the transfection technique—be it via lipofection, electroporation, or using viral vectors—can yield better results. This highlights the importance of preliminary experiments to identify the ideal conditions for the target cell type, ensuring that the method and medium align cohesively.
mRNA Design and Modifications
Designing mRNA for optimal transfection isn’t a one-size-fits-all approach. The structure and modifications of mRNA can either enhance or hinder its stability, cellular uptake, and translation efficiency. Several aspects come into play, making it essential for researchers to consider the nature of their mRNA when planning transfections.
- 5’ Capping and Polyadenylation: The inclusion of a 5' cap and a poly-A tail are common modifications that not only help in stability but are also crucial for the translation process. Modified caps can increase mRNA’s resistance to degradation and misleading host immune responses.
- Codon Optimization: Adapting the mRNA sequence to favor codons that are more frequently used in the host cells can improve translation efficiency. This is particularly relevant when the goal is to express a protein at higher levels quickly.
- Chemical Modifications: Making chemical alterations, like using modified nucleotides, can enhance the mRNA's longevity inside the cells. These adjustments also reduce immunogenicity, which is vital for achieving sustained expression without triggering unwanted immune responses.
In summary, the factors influencing transfection efficiency are multidimensional—ranging from the variability of cell types, culture conditions, to the design of the mRNA itself. It’s not just about getting the mRNA inside the cells; it involves a careful orchestration of many elements to ensure that the mRNA is not only delivered but also successfully translated into functional proteins.
Applications of GFP mRNA Transfection
GFP mRNA transfection has emerged as a vital tool in molecular biology, transforming how researchers approach various biological questions. The ability to transiently express protein markers, such as Green Fluorescent Protein, allows scientists to visualize processes within living cells in real time. This technique has widespread implications across multiple fields of research, providing a unique lens through which to study cellular dynamics, gene expression, and therapeutic interventions.
Studying Protein Localization
One major application of GFP mRNA transfection is in the study of protein localization. By tagging proteins of interest with GFP, researchers can track the movement and distribution of these proteins within cells. This is crucial for understanding complex cellular functions and pathways. For example, if a scientist is investigating a signaling protein linked to cancer, transfecting cells with GFP-tagged mRNA of that protein enables them to observe where it localizes in the cell under different conditions.
- Real-time monitoring: This provides real-time insights into protein dynamics, helping to clarify how proteins interact with each other and their cellular environment.
- Spatial distribution: Knowing the spatial distribution of proteins can reveal the mechanisms underlying various cellular processes, from metabolic pathways to the cell cycle.
The localization studies can lead to significant breakthroughs in understanding disease mechanisms, as abnormal localization often correlates with pathological states.
Investigating Gene Function
Another critical application of GFP mRNA transfection is gene function analysis. This process allows researchers to elucidate the roles of specific genes in cellular processes. By introducing mRNA that codes for GFP-fusion proteins, scientists can inhibit or facilitate the expression of accommodating genes and subsequently observe the effects.
- Gain-of-function studies: Transfecting cells with GFP mRNA enables the observation of cell responses, providing insights into gene activation or silencing and further clarifying their functional roles.
- Phenotypic assays: Researchers can design phenotypic assays to associate the presence of certain proteins with specific cellular behaviors, such as apoptosis or differentiation.
These insights help paint a clearer picture of gene function, facilitating the discovery of novel genetic interactions and contributing to our understanding of genetic diseases.
Therapeutic Uses and Future Directions
The therapeutic potential of GFP mRNA transfection is another exciting frontier. With gene therapy gaining traction, the possibility of delivering mRNA encoding therapeutic proteins has significant implications for treating conditions such as genetic disorders and cancers.
- Personalized medicine: This method could pave the way for personalized therapeutic strategies, where specific mRNA constructs are designed for individual patients based on their genetic profiles.
- Vaccine development: The role of mRNA in vaccines, notably the COVID-19 vaccines, emphasizes the potential for mRNA technology in rapidly developing effective treatments against various pathogens.
Looking forward, integrating GFP mRNA transfection with emerging technologies, such as CRISPR-Cas9, could revolutionize how we perceive and treat complex diseases. The continuous advancements in mRNA delivery systems and our understanding of cellular responses will only enhance the applicability of this technique, thus broadening its scope across various research disciplines.
In summary, GFP mRNA transfection serves as a powerful methodology that is reshaping our understanding of genetics, protein dynamics, and therapeutic approaches. Researchers are steadily unearthing its potential, creating exciting opportunities for innovation in molecular biology.
Challenges in mRNA Transfection
The escalation of mRNA transfection techniques has amplified the scientific community's interest in the efficiency and viability of such methodologies. However, the path forward isn't without its boulders. Addressing the challenges of mRNA transfection not only aids in refining techniques but also underscores the significance of careful consideration as researchers work toward optimizing gene delivery systems. These challenges encompass a variety of aspects, including immunogenic responses and the stability of mRNA itself.
Immunogenic Responses
A primary hurdle in mRNA transfection is the potential for immunogenic responses. The immune system, designed to detect and eliminate foreign invaders, can mistakenly recognize exogenous mRNA as a threat. When the body reacts, it can lead to inflammation or even autoimmune responses, which complicates the intended benefits of mRNA therapy.
Moreover, the immune response isn't uniform across different cell types. For instance, dendritic cells often exhibit a robust reaction to mRNA, affecting their role in antigen presentation. This variability can complicate the design of effective transfection strategies. Some strategies to mitigate immune activation include the use of chemically modified nucleotides, which can be less recognizable to the immune system while still functioning effectively.
"Understanding the balance between immunogenicity and efficacy is crucial for advancing mRNA transfection methods."
Stability and Degradation of mRNA
The stability of mRNA post-transfection poses a significant challenge, often dictating the success of protein expression. mRNA is naturally susceptible to degradation by ribonucleases, enzymes that chop up RNA into smaller pieces. Therefore, ensuring that the delivered mRNA remains intact long enough to produce the desired protein is essential.
Some common strategies to enhance mRNA stability include:
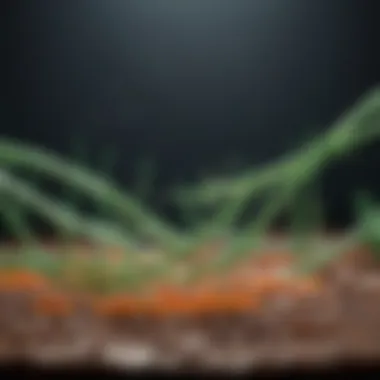
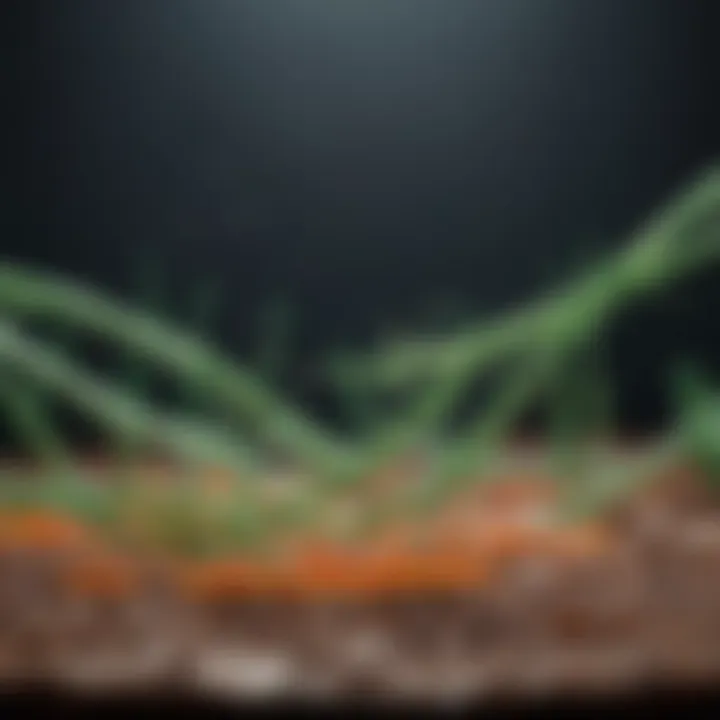
- Incorporation of a modified cap structure, which can protect the mRNA and enhance its translation.
- Using poly(A) tails that are longer in length to improve stability in the cytoplasm.
- Encapsulation within nanoparticles, which can shield the mRNA from degradation while facilitating cellular uptake.
Additionally, the design of mRNA itself plays a vital role in determining its stability and translational efficiency. By optimizing codon usage, researchers can lower the chances of ribonuclease engagement, leading to higher yields of the desired protein product.
In summary, while mRNA transfection holds immense potential in various fields, addressing these challenges is crucial. A better understanding of immunogenic responses and the stabilization of mRNA will enhance the efficacy of gene delivery systems and accelerate research efforts.
Recent Advancements in Transfection Technologies
In the rapidly evolving world of molecular biology, staying updated on advancements in transfection technologies is paramount. Researchers strive to find ways to deliver genetic material effectively while minimizing potential drawbacks. The advancement in transfection technologies specifically enhances methods used to introduce GFP mRNA into various cell types, promoting better expression of proteins crucial for research and therapeutic discoveries.
The efficacy of transfection techniques has significant implications not only for basic research but also for clinical applications. Recent advancements focus on improving delivery mechanisms, boosting cellular uptake, and ultimately leading to more consistent and higher yields of protein expression. Here are some key points to consider:
- Increased Efficiency: New techniques aim to increase the efficiency of mRNA entry into cells, facilitating greater uptake that can lead to enhanced protein yield.
- Reduced Toxicity: The refinement of delivery systems aims to lower cytotoxic effects, providing gentler solutions for sensitive cells.
- Specific Delivery: Innovations enable more targeted approaches to ensure that mRNA reaches only the intended cells, minimizing off-target effects and potential negative responses.
More refined technologies improve the backbone of transfection methods that researchers rely upon. Two notable advancements are the development of nanoparticle systems and the integration of CRISPR-Cas technology.
Nanoparticle Systems
Nanoparticle systems have emerged as a game-changer in facilitating mRNA transfection. The versatility of these systems allows for controlled delivery of mRNA into cells, enhancing both efficiency and specificity. In essence, nanoparticles, such as lipids, polymers, or even metallic nanoparticles, are capable of encapsulating mRNA and fostering its transport into target cells.
Some benefits of using nanoparticles include:
- Size and Surface Modifications: The small size of nanoparticles allows them to navigate biological barriers more easily. Customizable surfaces can be chemically altered to enhance cellular interaction.
- Controlled Release: Nanoparticles can be designed to release mRNA in response to specific stimuli, such as changes in pH or temperature, ensuring sustained expression strategies.
- Versatile Types: Researchers can choose from various formulations, from lipid nanoparticles like those seen in Covid-19 vaccines, to biodegradable polymers.
All in all, these properties facilitate higher degrees of control over mRNA delivery, setting the stage for impactful therapeutic approaches and research applications.
CRISPR-Cas Technology Integration
The integration of CRISPR-Cas technology into mRNA transfection strategies marks a vital advancement, enabling precise genetic modifications. This system not only allows researchers to edit genomes but also enhances the delivery of mRNA for purposes such as gene knock-in or knock-out experiments. The CRISPR-Cas tools provide a double-edged sword by helping to understand gene function while simultaneously allowing for potential therapeutic developments.
Considerable points about integrating CRISPR-Cas technology into mRNA transfection methods include:
- Targeted Gene Editing: By utilizing the CRISPR system, mRNA can be guided to specific genomic locations allowing for precise interventions.
- Synergistic Effects: Together with mRNA, CRISPR offers dual functionality—one to express proteins for study and another to modify the host genome’s response to those proteins.
- Improving Specificity and Reduce Off-Target Effects: The CRISPR system’s ability to target sequences with high precision means researchers can expect more reliable results with minimization of unintended modifications.
From understanding how cellular pathways function to developing potential therapies for genetic diseases, these advancements in transfection technologies represent a promising frontier. They offer a wealth of possibilities, building confidence that the next decade can yield significant breakthroughs in molecular biology.
Regulatory Considerations in mRNA Research
The realm of mRNA research has expanded rapidly, particularly with the development of GFP mRNA transfection techniques. As the field advances, so does the necessity for a structured regulatory framework to ensure that the methods employed are not only effective but also safe. Regulatory considerations are crucial since they bridge the gap between scientific innovation and public welfare. In this section, we will explore the ethical implications and the need for compliance in the context of mRNA use in research and therapeutics.
Ethical Implications of mRNA Use
When it comes to mRNA, the stakes are high, and ethical considerations cannot be put on the back burner. Researchers must navigate a minefield of ethical dilemmas, particularly regarding potential applications in gene editing and therapy. Key issues include:
- Informed Consent: It is paramount that researchers provide clear, comprehensible information regarding the mRNA products and their potential effects to all participants involved in studies.
- Privacy Concerns: Handling genetic information linked to mRNA transfection can lead to dilemmas about data security and participant privacy. Researchers must establish robust protocols to safeguard sensitive data.
- Dual-use Dilemmas: The potential for mRNA techniques to be used for both beneficial and harmful purposes raises questions about how to prevent misuse.
As we tread further into the realm of mRNA applications, the ethical landscape will likely continue to evolve, necessitating ongoing discussions and adjustments in practices. The balance of scientific progress against ethical considerations is delicate; ensuring that the promises of mRNA research can be realized without compromising moral standards is vital.
Compliance with Regulatory Frameworks
Just as a ship needs a sturdy compass to navigate through turbulent seas, mRNA research depends on adherence to regulatory frameworks to steer safe and ethical outcomes. Various agencies play a crucial role in overseeing mRNA-related research and its implications. Key points include:
- Governing Bodies: Agencies like the U.S. Food and Drug Administration (FDA) and the European Medicines Agency (EMA) establish guidelines that researchers must follow to ensure safety and efficacy in mRNA applications.
- Clinical Trials Oversight: Regulatory structures enforce strict protocols for clinical trials, ensuring that mRNA therapies are only tested under well-defined and controlled conditions.
- Monitoring Adverse Effects: Post-marketing surveillance is critical. Once a treatment is in the public domain, compliance standards require constant monitoring for potential adverse effects.
- Accountability: Researchers and companies involved in mRNA applications must also be prepared for audits and inspections by regulatory bodies, ensuring that ethical standards and safety protocols are consistently upheld.
In summary, navigating the regulatory landscape of mRNA research is no small feat. It demands a careful balance of innovation and compliance, ensuring that the progression in this exciting field moves forward with a grounded sense of responsibility.
"With great power comes great responsibility." This age-old adage rings profoundly true in the realm of mRNA research, where the pursuit of knowledge must never outpace the ethical considerations that guard public well-being.
Future Perspectives on GFP mRNA Transfection
The exploration of GFP mRNA transfection holds significant promise for the future of molecular biology. As research techniques continue to evolve, the implications of mRNA transfections stretch beyond mere laboratory applications; they impact the broader spheres of therapeutics and genetic engineering. Understanding these future perspectives is crucial for harnessing the full potential of this innovative approach. As we stand on the threshold of these advancements, several elements must be considered: from the efficiency of mRNA delivery to ethical implications in gene therapies and the search for novel applications.
Future innovations could improve how we transfect cells using GFP mRNA, making the process more robust and adaptable to various research needs. This will not only facilitate successful transfections but also ensure that results are reproducible and reliable. The primary benefit here lies in the potential for higher precision in genetic manipulation, and consequently, more profound insights into cellular functions.
Additionally, as methodologies become less invasive and more effective, the therapeutic implications could lead to groundbreaking treatments for conditions previously deemed challenging to address. The ability to deliver mRNA efficiently could allow for on-demand protein production, opening the door to rapid responses in disease treatment.
Innovations on the Horizon
Future innovations in GFP mRNA transfection encompass a range of exciting technological advancements. One pivotal area of focus is the development of more sophisticated delivery systems. For example, researchers are exploring the use of bioconjugates—chains of biomolecules that are capable of delivering mRNA in a manner that's less repsponsive to immunity issues. This could result in more effective transfection techniques.
Another innovative approach lies in the realm of artificial intelligence and machine learning. These technologies could optimize transfection protocols by analyzing vast amounts of data to identify the ideal conditions for each experiment. With AI's capabilities, it’s feasible to predict successful outcomes in mRNA interactions, leading to tailored transfection strategies that enhance efficacy.
"With the advent of AI, we could soon explore transfection approaches that specifically cater to rare or challenging cell types."
Moreover, the integration of nanoparticles is gaining traction. These minuscule carriers can package mRNA in a way that boosts cellular uptake while minimizing degradation before it reaches the target site. Nanoparticle systems may work like finely tuned instruments, ensuring that mRNA reaches its destination in optimal condition. This promises a tremendous leap forward in the efficiency and effectiveness of mRNA transfection protocols.
Potential Cross-Disciplinary Applications
GFP mRNA transfection stands to impact various fields beyond molecular biology. The cross-disciplinary applications of this technology are vast. In medicine, for instance, the advancements in transfection efficiency could lead to significant leaps in gene therapy protocols. The ability to introduce genes responsible for producing therapeutic proteins can potentially revolutionize treatment strategies for genetic conditions and diseases such as cancer.
Furthermore, in the field of agriculture, gene editing combined with efficient mRNA transfection may enhance crop resilience to diseases and environmental stresses. Plants engineered to express specific genes could flourish even in challenging conditions, ultimately leading to increased food security.
In biotechnology, GFP mRNA transfection can be harnessed for synthetic biology projects, where microbes can be tailored for specific industrial applications such as biofuel production or waste remediation. The implications here are vast and could result in sustainable practices that have a far-reaching positive impact on the environment.
Thus, as GFP mRNA transfection technology advances, it warrants attention from various sectors, emphasizing the interconnectedness of scientific disciplines. By fostering a collaborative spirit, these innovations may lead to breakthroughs that not just influence a single field but enhance numerous aspects of life, where science and society converge.