Exploring the Foundations of Gravity Generation
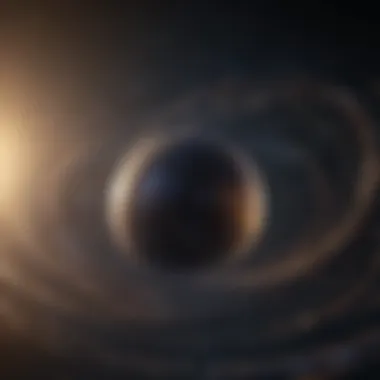
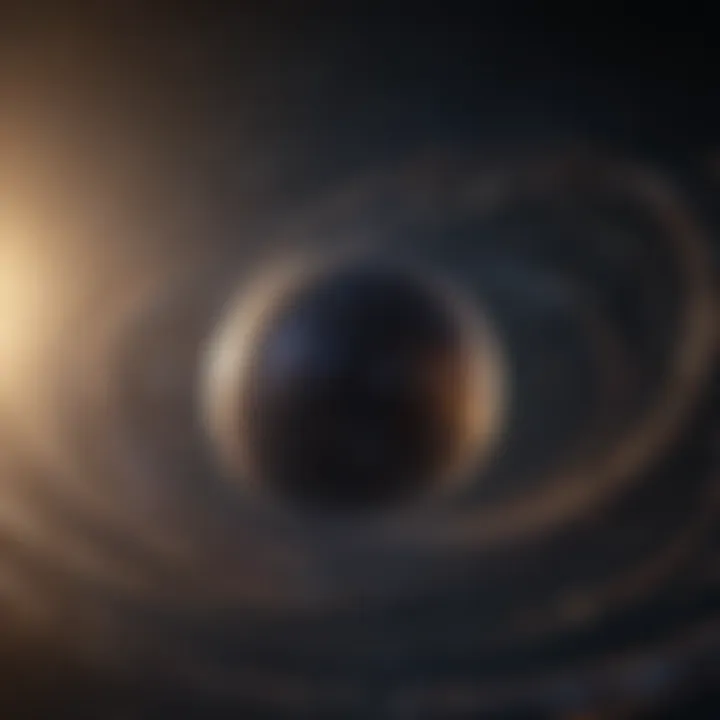
Intro
Gravity is a force that shapes our universe and affects everything from the tiniest particles to the massive celestial bodies. Understanding how gravity is generated is fundamental to physics and many scientific disciplines. The mechanisms behind gravity touch on concepts such as the theory of relativity, quantum mechanics, and cosmology. This article aims to provide a thorough overview of these concepts, their historical evolution, and the latest insights in research.
Research Overview
Summary of Key Findings
In exploring gravity generation mechanisms, several key findings emerge:
- Historical Context: The evolution of gravity theories from Newton's classical mechanics to Einstein's general relativity reveals a shift in understanding the universe's structure.
- Recent Advances: There are ongoing studies looking at quantum gravity, string theory, and experimental efforts to detect gravitational waves. These developments yield significant implications for our comprehension of the universe.
- Interdisciplinary Impact: Gravity plays a crucial role not only in physics but also in fields like astronomy, geophysics, and even understanding biological processes.
Research Objectives and Hypotheses
The primary objectives of this research include:
- To delineate the fundamental principles that govern the generation of gravity.
- To examine both classical theories and contemporary approaches, assessing their contributions and limitations.
- To explore future research directions that may provide new insights into gravity.
From these objectives, the following hypotheses arise:
- Gravity as Curvature: One hypothesis posits that gravity can be fully understood as the curvature of spacetime, consolidating both classical and quantum perspectives.
- Quantum Gravity: Another suggests the existence of a unified theory that reconciles general relativity with quantum mechanics, offering a more comprehensive understanding of gravitational forces.
Methodology
Study Design and Approach
This article draws upon a systematic literature review, synthesizing findings from historical texts, modern research articles, and key theoretical frameworks. By critically analyzing these sources, a clearer picture of gravity's mechanisms emerges.
Data Collection Techniques
Data is collected through multiple avenues, including:
- Reviews of academic journals on astrophysics, theoretical physics, and cosmology.
- Interviews or discussions with experts in gravitational theory and related fields.
- Analysis of recent experimental data from research institutes focusing on gravitational waves and other phenomena.
"The study of gravity not only deepens our understanding of the cosmos but also influences our conception of reality itself."
Given the complexity and significance of gravity, this exploration aims to provide a robust framework that can aid students, researchers, educators, and professionals alike in navigating this intricate subject.
Foreword to Gravity
The topic of gravity is fundamental in understanding not only physical science, but also the universe. It governs motion and structure, influencing everything from the orbits of planets to the behavior of objects on Earth. Without grasping gravity, one cannot fully appreciate the additional complexities that arise in higher-level physics, such as astrophysics and cosmology.
In this segment, we will delve deeply into gravityβs very definition and its rich history, focusing on key figures such as Sir Isaac Newton and Albert Einstein. Both contributed crucial theories that shaped our perception of gravity. The examination of these perspectives highlights the evolution of scientific thought and underlines the relationship between empirical evidence and theoretical frameworks.
Understanding the intricacies of gravity also illuminates its essential role in engineering disciplines and modern technology. From spacecraft navigation to medical applications, the principles derived from gravity research continuously impact our lives. Thus, a detailed exploration of gravity sets the stage for broader discussions against the backdrop of current scientific discovery.
Defining Gravity
Gravity can be defined simply as a natural phenomenon by which objects with mass are attracted to one another. The strength of this attraction depends on the masses involved and the distance between them. An integral part of the Earth's structure, gravity influences everything we experience on the planet.
Scientists describe gravity in terms of gravitational force, typically expressed in Newtons. This force dictates the motion of objects on Earth and throughout the cosmos. Understanding the basic principles of gravity is imperative for unraveling more complex scientific concepts.
Historical Perspectives
Newton's Law of Universal Gravitation
Sir Isaac Newton's contribution to gravity lies in his formulation of the Law of Universal Gravitation, published in 1687. This law posits that every mass attracts every other mass with a force that is proportional to their masses and inversely proportional to the square of the distance between them. One of the key characteristics of this law is its applicability across vast distances; it explains the motion of planets and predictably calculates the orbits of celestial bodies.
This law remains a cornerstone in physics for its simplicity and effectiveness in explaining gravitational phenomena. Its major benefit in this narrative is its intuitive structure, making it easily understandable and accessible. However, it does have limitations, especially in dealing with extremely strong gravitational fields or at relativistic speeds. For a detailed original source, consult Wikipedia.
Einstein's General Relativity
Albert Einstein's General Relativity took a different approach to understanding gravity, offering a more comprehensive model. Introduced in 1915, this theory posits that gravity is not merely a force, but a result of the curvature of space-time caused by mass. The defining characteristic of General Relativity is how it integrates gravity into the fabric of space-time, providing a more dynamic and flexible understanding.
This theory has become essential for advanced scientific study. Its calculations extend into predicting phenomena, such as gravitational waves, which have been empirically validated through modern experiments. The drawback, however, lies in its mathematical complexity compared to Newtonβs law, presenting a barrier to immediate comprehension for many. However, its impact on theoretical physics and cosmology is undeniable. For a more detailed exploration, refer to Britannica.
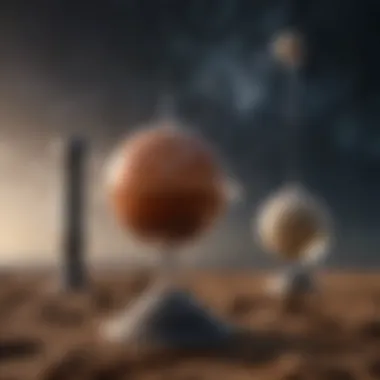
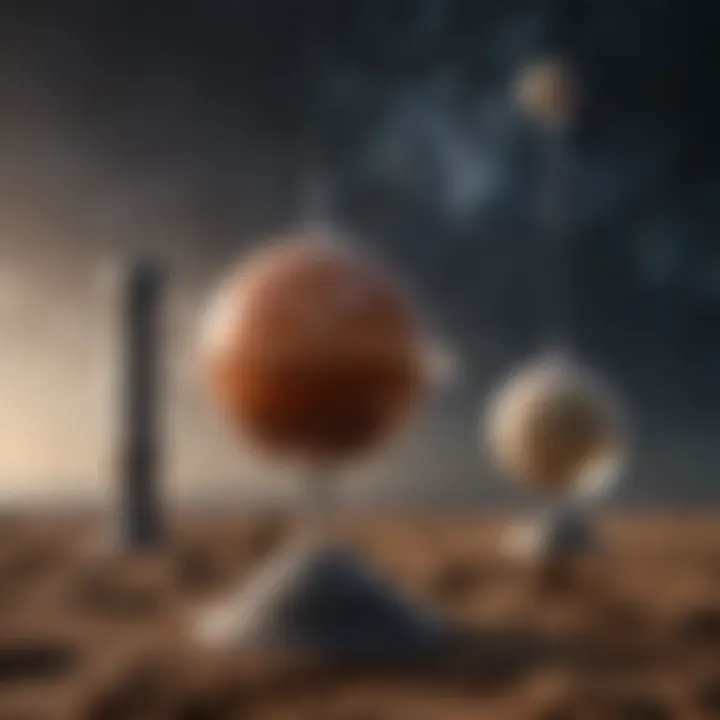
In this section of our article, we have set the groundwork for understanding gravity by analyzing its definition and historical background. The insights gained from Newton and Einstein provide a lens through which we can explore ongoing developments and challenges related to gravity research.
Theoretical Foundations
The theoretical foundations of gravity are pivotal for understanding its complex nature. They set the stage for how scientists interpret gravitational interactions in various contexts. This section discusses the historical theories and modern advancements that form the basis for contemporary gravity research.
These underpinning theories help clarify the relationship between mass and the gravitational forces they produce. Furthermore, they touch upon foundational principles that dictate the behavior of gravity across the cosmos. Grasping these theories provides insights into how gravity influences astrophysical phenomena and technological applications. Understanding this foundation also guides future research endeavors, bridging philosophical concepts with empirical science.
Newtonian Gravity
Newtonian gravity, proposed by Sir Isaac Newton, revolutionized our understanding of gravitational forces. Newton's law posits that every mass attracts every other mass through a force inversely proportional to the square of the distance between their centers. This concept simplified gravitational calculations, enabling precise predictions of planetary motions.
One primary advantage of Newtonian gravity is its simplicity and straightforward application. It provides robust predictions for many common scenarios. However, it does fall short in extreme conditions, particularly with massive bodies or at very high velocities.
General Relativity and Gravity
General Relativity, introduced by Albert Einstein, presented a shift in understanding gravity. This theory posits that gravity is not merely a force but rather a curvature of space-time caused by mass. This perspective allows for a more nuanced interpretation of gravity, particularly when exploring the dynamics around large celestial bodies.
Space-Time Curvature
Space-time curvature is a critical aspect of General Relativity. It fundamentally changes how we view gravity, shifting from a Newtonian attractive force to the geometry of space-time itself. In this framework, larger masses like stars and planets warp the fabric of space-time around them, resulting in the observed gravitational effects.
One key characteristic of space-time curvature is its ability to explain phenomena that Newtonian gravity cannot, such as the bending of light around massive objects. This feature makes it a valuable tool in understanding the universe, particularly in areas like cosmology. The advantages of this perspective include providing explanations for gravitational lensing and the expansion of the universe.
However, one disadvantage is the complexity of calculations involved, often requiring sophisticated mathematics and extensive computational resources.
Gravitational Waves
Gravitational waves are ripples in space-time generated by accelerating masses, as predicted by General Relativity. Their existence was confirmed by the LIGO observatory in 2015, marking a milestone in gravitational research. These waves provide new insights into the dynamics of massive cosmic events, such as colliding black holes or neutron star mergers.
The key characteristic of gravitational waves is their ability to travel across vast distances without losing strength. This allows scientists to observe events that are otherwise undetectable through traditional means. This is beneficial as it opens a new observational window into the universe, revealing details about otherwise hidden astrophysical phenomena. However, the detection and precise measurement of gravitational waves require advanced technology, which can limit accessibility for research.
Quantum Gravity Theories
Quantum gravity theories are attempts to reconcile General Relativity with quantum mechanics. They aim to provide a comprehensive framework for understanding gravity at the smallest scales.
String Theory
String theory proposes that the fundamental constituents of the universe are not point particles but rather one-dimensional "strings". This theory suggests that different vibrational modes of these strings correspond to different particles, including gravitons, the hypothetical carriers of gravitational force.
The unique feature of string theory is its potential to unify all fundamental forces should it be experimentally validated. As a theoretical foundation, it offers a compelling narrative for integrating gravity with quantum physics. However, one major drawback is that it is currently not testable through direct experiments, leaving it in a speculative domain.
Causal Set Theory
Causal set theory offers an alternative approach by proposing that spacetime is fundamentally discrete rather than continuous. It posits a set of points arranged in a causal order, presenting a new way to think about gravitational phenomena on a quantum level.
This feature allows researchers to explore the implications of quantum mechanics on gravity directly. It is a beneficial choice in this article because it presents a radical departure from classical theories, opening discussions on the nature of time and space. Yet, this theory is still in its infancy and faces challenges regarding how to connect its ideas with established physics.
Understanding these theoretical foundations is essential for grasping how gravity interacts with the universe in multiple dimensions. By examining these models, we discover the limitations and advantages inherent in our theories, guiding us toward future advancements.
Experimental Evidence
Experimental evidence serves as the bedrock upon which scientific theories are validated or refuted. In the context of gravity, it becomes paramount as we strive to comprehend its intricate mechanisms. This section delves into the empirical approaches that have been undertaken to test theoretical frameworks surrounding gravity. By highlighting specific examples and practices, we can appreciate the robustness of experimental evidence.
Testing General Relativity
Testing General Relativity requires scrutiny through various experimental methodologies. General Relativity, proposed by Albert Einstein, is significant for how it radically altered our concept of gravity, presenting it not as a force, but as the curvature of spacetime. Through rigorous experimentation, we seek to confirm or challenge Einstein's postulations.
Observational Tests
Observational tests form a key pillar in evaluating General Relativity. They rely on astronomy and astrophysical phenomena to provide insights. For instance, the bending of light around massive objects has been observed; this is congruent with Einstein's predictions regarding light trajectories. The key characteristic of observational tests is their basis in large-scale cosmic events, making them not only informative but also compelling in real-world applications.
The unique feature of observational tests lies in their ability to provide tangible evidence drawn from vast cosmic distances. While they have proven beneficial, they are sometimes limited due to the scale and nature of cosmic events that may not occur frequently. This means that while observational tests yield substantial insights, they also risk a degree of uncertainty linked to the infrequency of observable events.
Laboratory Experiments
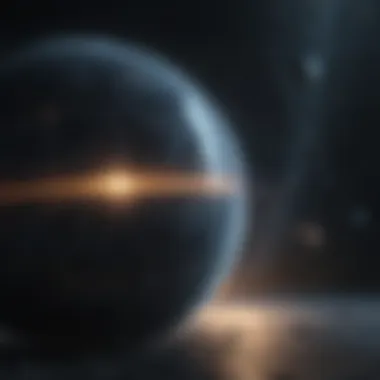
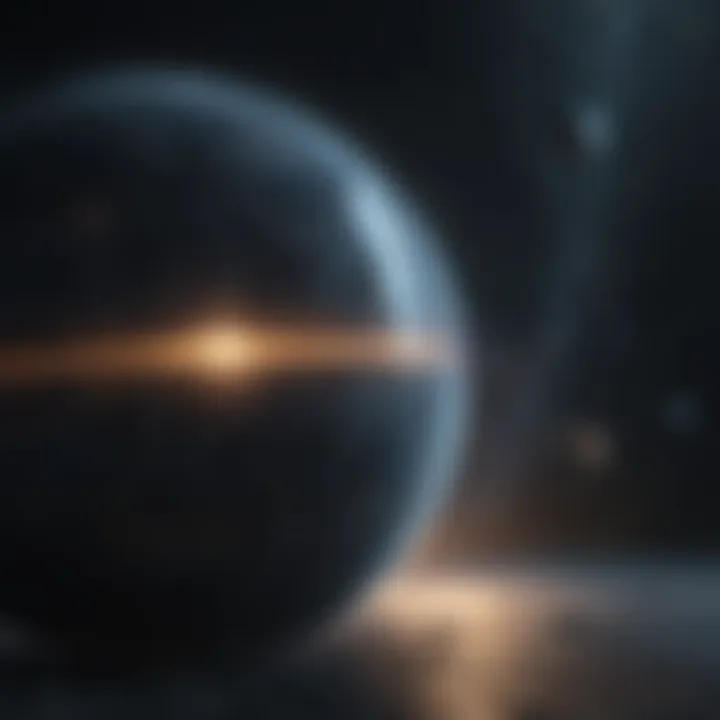
Laboratory experiments offer controlled environments to test the principles of gravity more directly. These experiments can manipulate variables and provide data that may be difficult to obtain through observational methods. A primary characteristic of laboratory experiments is their ability to produce repeatable and consistent results, which is a hallmark of scientific methodology.
The hallmark of these experiments is the capacity to explore gravity's effects on small scales or under controlled conditions. This approach fosters precision and allows for rigorous exploration of gravitational forces without the vast complexities encountered in cosmic tests. However, it can also face limitations in its applicability to large-scale phenomena, which can differ significantly from small system behaviors.
Gravitational Waves Detection
Gravitational waves detection is an exciting frontier that underscores the modern aspects of gravitational research. Detected by advanced observatories like LIGO, these waves are ripples in spacetime caused by massive cosmic events, such as black hole mergers. The detection of these waves provides vital implications for our understanding of gravitational dynamics and supports the existence of General Relativity's predictions.
Gravitational waves not only bolster the theoretical framework of gravity but also enhance our ability to study isolated events across the universe. Observing these waves enhances our comprehension of the universe's evolution and its fundamental forces, simultaneously illuminating areas like dark matter and energy. The advances in detection technology and the insightful data gathered present new roads for research and understanding of gravity, showcasing the importance of experimental evidence in pushing the boundaries of our knowledge.
Gravity in Cosmology
Gravity plays a central role in shaping our universe. It influences the motion of galaxies, the existence of planets, and even the path light travels. Understanding gravity in the context of cosmology helps us grasp critical cosmic phenomena, such as the formation of structures, cosmic evolution, and the elusive nature of dark matter. These elements have significant implications for our understanding of the universe and its future development.
Role of Gravity in the Universe
Formation of Structure
The formation of structure in the universe is a fundamental process that confirms gravity's significance. It begins with small density fluctuations in the early universe. Gravity draws matter together, leading to the clumping that forms stars, galaxies, and clusters. This gravitational attraction is essential because it explains how large-scale structures evolved from earlier simpler conditions.
One key characteristic of this process is how gravity influences the balance between expansion and collapse. If gravity were weaker, structures would not form as efficiently. The beneficial aspect of studying formation of structure lies in its potential to explain the stability and arrangements of galaxies today.
However, a unique feature of this construction process is its reliance on the presence of dark matter. While we cannot see dark matter, its gravitational effects are evident in the way galaxies cluster. The advantages are many; understanding this relationship offers insights into both gravity's behavior and the unknown aspects of the cosmos.
Cosmic Evolution
Cosmic evolution examines how the universe has changed over time, with gravity being a key player in this story. It governs the large-scale motion of galaxies and helps us understand the lifecycle of celestial bodies. Through gravity, we learn about the genesis of elements in stars, the birth and death of stars, and the formation of planets.
A crucial characteristic of cosmic evolution is its reliance on fundamental physical laws, including those that describe gravity's influence over vast distances. This is particularly beneficial because it provides a framework for interpreting observational data. The study of cosmic evolution highlights the interplay between gravity and other forces in shaping the universe's evolution. It poses intriguing questions regarding the fate of the universe.
While studying cosmic evolution can be advantageous, it also presents challenges. Gravitational interactions can lead to chaotic behaviors that complicate our understanding. Overly simplified models may not accurately predict cosmic events.
Dark Matter and Gravity
Dark matter represents one of the most pressing mysteries in modern cosmology. It does not interact electromagnetically, which makes it invisible to telescopes but still exerts gravitational effects. Gravitational interactions help reveal the presence of dark matter through galaxy rotation curves and gravitational lensing.
The relationship between dark matter and gravity is vital for theories about the universe's structure. Understanding dark matter helps flesh out models of cosmic evolution. With increasing research in this area, it becomes clearer how dark matter influences galaxy formation and clustering.
The challenge remains in identifying the nature of dark matter itself. Current observations and theoretical frameworks guide researchers toward a more comprehensive understanding. The interaction between dark matter and gravity could illuminate many aspects of both particle physics and astrophysics, making this topic rich for exploration.
Engineering Gravity
Engineering gravity is a fascinating and crucial topic within the field of gravitational research. It blends concepts from both theoretical physics and practical engineering to explore how gravity can be manipulated or even synthesized for various applications. The significance of this topic lies in its potential to transform how we understand movement in space and expand technologies on Earth. As we continue to investigate new methods for generating and controlling gravitational effects, the implications for science and technology become more exotic and intriguing.
Theoretical Mechanisms
Artificial Gravitational Fields
Artificial gravitational fields refer to the creation of gravity-like forces through various means, primarily in controlled environments. These fields can simulate gravitational effects thus allowing researchers to study phenomena usually governed by gravity under different conditions. One of the key characteristics of artificial gravitational fields is their flexibility; they can be generated in laboratories or space stations, giving scientists a strategic advantage to conduct experiments without the confines of Earthβs gravity.
A distinct advantage is that these fields enable the examination of biological and physical processes in a gravity-free environment. However, the challenges include maintaining stability of these fields and the costs associated with such technologies. Nonetheless, the exploration of artificial gravitational fields remains a popular and promising area in understanding gravity generation.
Controlled Gravity Manipulation
Controlled gravity manipulation involves the direct assessment and alteration of gravitational forces, which can greatly contribute to advancements in various scientific fields, including space exploration and medical research. The ability to manipulate gravity gives scientists an avenue to modify environments and conditions for experiments in ways that were previously unimaginable. This characteristic makes it indispensable in the engineering of gravity because it allows for precise control over gravitational stimuli during testing.
It presents unique features such as potential applications in space travel where adjusting gravity can help reduce the physiological impacts of prolonged exposure to microgravity on astronauts. Despite these advantages, ethical considerations and the technological challenges in achieving practical applications of controlled gravity manipulation can create obstacles that researchers must navigate carefully.
Practical Applications
Spacecraft Maneuverability
The advent of technologies that leverage gravity manipulation has made spacecraft maneuverability one of the most vital practical applications in this domain. This involves adjustments in gravitational forces to enhance navigation and control of spacecraft in various interaction zones within gravity wells.
Key aspects of this concept include the optimization of fuel usage and the increase in operational safety. It is significant in long-distance space missions to maintain control over spacecraft trajectories and dynamics. The unique responsiveness of modified gravitational influences can lead to efficient trajectories and reduce the travel time between distant celestial bodies.
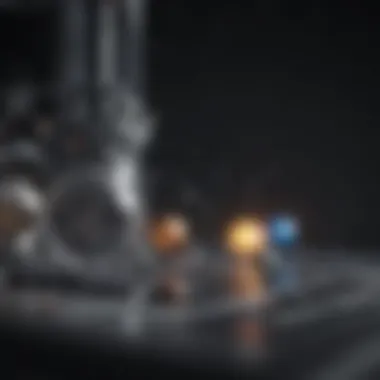
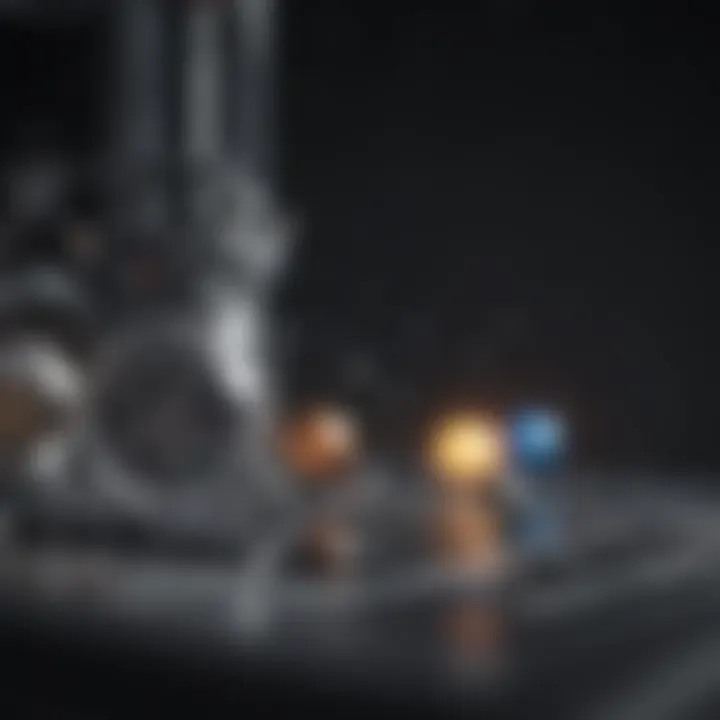
However, the downside lies in the need for sophisticated engineering systems that require substantial resources and careful planning to implement effectively.
Medical Technologies
In the medical field, the application of principles of gravity engineering has opened new avenues for patient care and rehabilitation. Technologies that utilize artificial gravitational fields can help in understanding muscle and bone deterioration in microgravity, which is particularly beneficial for astronauts during long durations in space.
One key characteristic is its potential to enhance physical therapies and surgical procedures. Through controlled environments, doctors can apply gravitational forces to assist in recovery processes. The unique feature of these technologies ensures targeted therapy, allowing for more personalized medical treatment. However, the limitations often coincide with the availability of these high-end technologies, which may not be accessible in all medical facilities.
Engineering gravity is at the intersection of theoretical understanding and practical application. As we probe deeper into the mechanisms of generating gravity, the future holds immense possibilities that can redefine concepts of movement and force in both cosmic and terrestrial frameworks.
Challenges in Gravity Research
The exploration of gravity is more than just understanding its effects on objects; it embodies one of the deepest questions in physics. The challenge lies in the quest to develop a coherent framework that unifies various theories of gravity. This unification is essential, as it aims to reconcile observations made at different scales and under different conditions. Significant progress has been made, but questions remain, making this field rich with ongoing inquiry and exploration.
The Quest for a Unified Theory
The search for a unified theory of gravity is a fundamental challenge that researchers face. Currently, general relativity and quantum mechanics stand as two pillars of modern physics. However, they operate under distinct principles. General relativity describes gravity as a curvature of spacetime caused by mass, while quantum mechanics governs the behavior of the smallest particles. These theories conflict when applied to extreme conditions, such as inside black holes or at the beginning of the universe.
Despite their individual successes, a framework is needed to bridge these theories. Concepts like string theory attempt to provide a unified perspective but remain largely speculative. The implications of successfully achieving a unification go far beyond academic discussions. A coherent theory would enhance our understanding of the universe, leading to advancements in technologies and potentially answering profound questions about existence itself.
Technological Limitations
The advancement of gravity research is significantly hampered by technological limitations. Many theoretical proposals exist, but validating them requires sophisticated equipment and methodologies. Current instruments, such as gravitational wave detectors, provide valuable data but have limitations in sensitivity. The detection of weaker gravitational waves and understanding their properties requires continuous improvements in technology.
Moreover, conducting experiments that simulate extreme gravitational conditions can be prohibitively difficult. Environments like those near black holes or during high-energy particle collisions are hard to replicate in a laboratory setting. This means that much of our understanding remains theoretical, waiting for the technological advancements necessary to test these theories directly.
"The ingenuity and creativity of our technological advancements are crucial to pushing the boundaries of gravity research forward."
In summary, the challenges in gravity research are numerous and complex. The quest for a unified theory highlights the need for deep integration of existing principles, while technological limitations serve as a barrier to empirical validation. Overcoming these challenges will require collaboration across disciplines and sustained focus on innovative experimental designs.
Future Directions
The exploration of gravity generation mechanisms is paramount for advancing our understanding of the universe. Future research in this field can yield significant insights, pushing the boundaries of both theoretical and practical applications. Key areas of focus include advancements in experimental techniques and interdisciplinary approaches, which can foster collaboration across various scientific domains.
Advancements in Experimental Techniques
Innovative experimental methods are crucial for testing existing theories of gravity and developing new ones. Researchers are exploring cutting-edge tools like atom interferometers and laser-based sensors which can measure gravitational effects with extreme precision. These advancements not only enhance our ability to conduct experiments but also broaden our understanding of phenomena such as gravitational waves and black holes.
For instance, the enhancement in measurement resolution allows scientists to explore phenomena that were previously theoretical. This can lead to the discovery of new gravitational behaviors or even deviations from established models. The exploration of these techniques may bridge gaps in our knowledge and provide answers to longstanding questions about the fundamental nature of gravity.
Interdisciplinary Approaches
Collaboration between Physics and Engineering
The collaboration between physics and engineering plays a pivotal role in advancing our understanding of gravity. This partnership is characterized by the practical application of theoretical concepts. By integrating theoretical physics with engineering solutions, researchers can develop novel technologies aimed at manipulating gravitational forces.
A unique aspect of this collaboration is the design of experimental setups that integrate advanced materials and technology. This integration can lead to significant breakthroughs in areas such as gravity-based propulsion systems or improved gravitational wave detectors. Each field brings its strengths, resulting in a synergistic effect that enhances research capabilities.
Implications for Earth Sciences
The implications for Earth sciences from gravity studies are profound. Understanding how gravity functions is crucial for comprehending various geological processes such as plate tectonics and gravitational anomalies. By studying these interactions, researchers gain insight into Earth's internal structure and dynamics.
A key feature of this integration is its practical application in resource exploration and environmental monitoring. For example, gravity measurements can aid in locating natural resources or assessing the stability of geological formations. This interdisciplinary approach highlights how the understanding of gravity can be beneficial for both scientific inquiry and practical applications in Earth sciences.
The End
In examining the mechanisms of generating gravity, it becomes evident that this field of study is not just a matter of theoretical exploration, but one of profound practical implications as well. The understanding of gravity is a foundation upon which much of current physics builds. The goals of this article culminate in emphasizing the centrality of gravity in both cosmological contexts and human technology.
Summarizing Key Insights
Throughout this article, key insights have emerged that illustrate the complexity and multifaceted nature of gravity:
- Historical Context: The evolution of the understanding of gravity from Newton's universal law to Einstein's theory showcases the paradigm shifts in scientific thinking.
- Theoretical Frameworks: The comparison between Newtonian gravity and general relativity highlights their applicability and limits. Quantum gravity approaches invite innovative thoughts on reconciling the two major theories.
- Experimental Evidence: The success in testing general relativity and detecting gravitational waves illustrates the empirical backbone that supports current theoretical advancements.
- Engineering and Practical Applications: Recognizing gravityβs role in technology, such as in spacecraft maneuverability and medical technologies, shows its relevance to everyday life and future innovations.
These insights collectively underscore that gravity is not merely a force but a critical component of the universe and our understanding of it.
Call for Continued Research
The necessity for ongoing research in gravity and related fields is apparent. Several areas warrant attention:
- Unified Theories: Investigating a comprehensive framework that integrates general relativity with quantum mechanics remains a tantalizing challenge, holding potential for groundbreaking discoveries.
- Technological Progress: Advances in experimental tools can lead to more precise measurements of gravitational effects, shedding light on phenomena like dark matter.
- Interdisciplinary Collaboration: Cross-integration with engineering, astronomy, and earth sciences may yield fresh perspectives and applications. The complexities of gravitational phenomena can benefit from diverse expertise and approaches.