Exploring the Potential of DSSO Crosslinker in Biochemistry
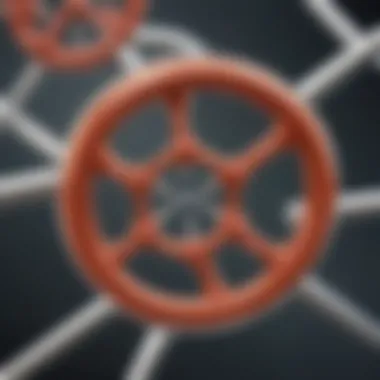
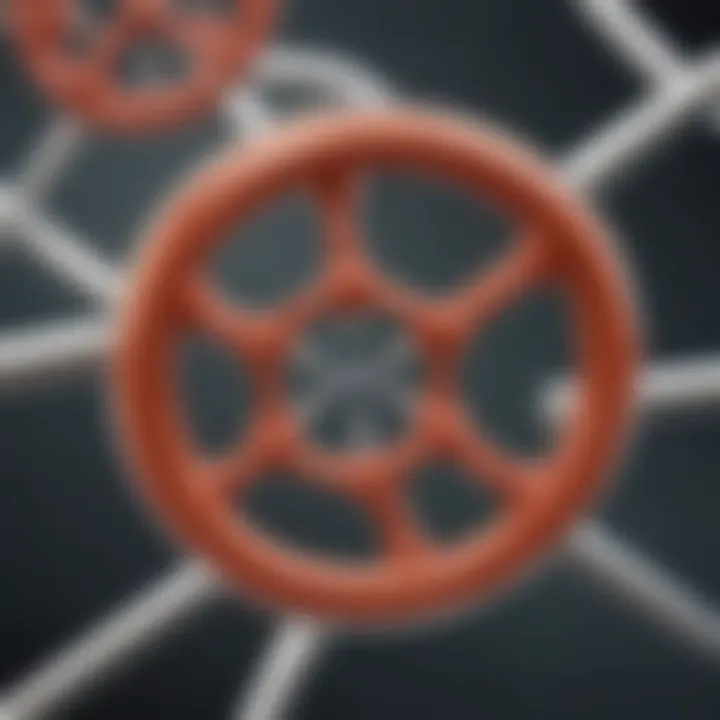
Intro
The dsso crosslinker is gaining recognition in the realm of biochemical research. This compound holds significant promise in the study of proteins and their interactions. Understanding its chemical properties is vital for scientists seeking to unlock the complex dynamics of molecular biology. As researchers delve into how dsso impacts biochemical processes, the potential for groundbreaking discoveries emerges.
In this article, we will explore the various dimensions of the dsso crosslinker. We will cover its key characteristics, applications in experimental designs, and its contribution to advancing our knowledge of protein interactions. The objective is to provide a clear and concise resource for students, researchers, and educators interested in the nuances of this compound and its implications in the field.
Research Overview
Summary of Key Findings
The study of dsso crosslinker reveals its unique ability to stabilize protein structures. It enhances the understanding of how proteins interact within cellular environments. Key findings suggest that dsso effectively couples with specific amino acid residues, leading to more reliable data in protein analysis.
Moreover, the dsso crosslinker shows promise in various applications, such as mass spectrometry and structural biology. Researchers have noted its effectiveness in providing insights into protein conformational changes and binding kinetics.
Research Objectives and Hypotheses
The primary objective of research into dsso crosslinker is to assess its impact on the accuracy and reliability of biochemical analysis. Specific hypotheses include:
- ****: dsso enhances the stability of protein-protein interactions compared to traditional methods.
- ****: Utilizing dsso in protein studies increases data reproducibility and quality.
- ****: dsso can be applied effectively across different types of protein classes, leading to broader applications in biochemical research.
Methodology
Study Design and Approach
The examination of dsso crosslinker involved a systematic approach. Scientists designed experiments to evaluate the effectiveness of dsso in various biochemical contexts. These contexts included analyzing protein interactions through crosslinking, especially under different environmental conditions. Protocols were established to ensure consistency and reproducibility.
Data Collection Techniques
Data collection utilized advanced techniques, including:
- Mass Spectrometry: For analyzing crosslinked products and determining molecular weights.
- Chromatography: To isolate proteins and assess their stability post-crosslinking.
- Immunoblotting: For validating the presence of specific protein interactions.
Together, these methods provided a robust framework to evaluate the utility and effectiveness of dsso crosslinker in various scenarios.
The dsso crosslinker is transforming the landscape of biochemical research by offering more reliable tools for analyzing complex protein interactions.
Prelude to dsso Crosslinkers
The dsso (disuccinimidyl suberate) crosslinker plays a pivotal role in the field of biochemical research, particularly regarding the analysis of protein interactions and the structural comprehension of biological complexes. Understanding dsso crosslinkers is crucial for any researcher engaged in the molecular biology arena. These crosslinkers are essential in enhancing the fidelity of measurement in various biochemical assays, allowing for a nuanced exploration of cellular mechanisms.
Definition and Overview
Dsso crosslinkers are a class of chemical compounds that facilitate the formation of stable covalent bonds between proteins. Specifically, dsso is a bifunctional crosslinker; it contains two reactive groups that can bond with amine functional groups found in proteins. This feature makes dsso an effective tool for stabilizing transient or weak protein-protein interactions, an aspect that is often a significant challenge in biochemical studies. The versatile nature of dsso allows it to be used in various applications, including immunoprecipitation, mass spectrometry, and structural biology studies.
The chemical structure of dsso is designed to create linkages that can withstand different experimental conditions while maintaining the native conformation of proteins, thus preserving the biological relevance of the interactions being studied. This inherent property of dsso makes it a preferred choice among researchers, especially when precise measurements are integral to experimental success.
Historical Context
The development of crosslinking agents can be traced back several decades as scientists sought better methods to study complex protein interactions. Early crosslinkers had limitations, often suffering from issues of specificity and stability. The introduction of dsso in the 1990s marked a significant turning point. Researchers began to recognize the advantages provided by its unique structure and reactivity profile.
The extensive use of dsso can be attributed to its ability to covalently link proteins without altering their native states significantly. Over the years, it has been utilized in countless studies that aimed to unravel intricate signaling pathways and protein functions within living cells. Its adoption has grown alongside advancements in related technologies, further strengthening its position in biochemical research.
As scientists continue to explore the capabilities and applications of dsso crosslinkers, the focus on refining methodologies and understanding the underlying biochemical dynamics remains strong. The historical progression leading to the current understanding of dsso illustrates its critical relevance in modern biochemical techniques.
Chemical Properties of dsso Crosslinkers
Understanding the chemical properties of dsso crosslinkers is essential in biochemical research. These properties dictate how dsso interacts with biomolecules, influencing the effectiveness of experimental protocols. Key elements include molecular structure, reactivity, and stability. Each aspect helps researchers determine the best conditions for using dsso crosslinkers to obtain reliable results.
Molecular Structure
The molecular structure of dsso crosslinkers plays a crucial role in their function. It consists primarily of a unique 1,2-diaminoethane core, which provides the necessary framework for crosslinking. This structure facilitates interactions with amine groups in proteins and nucleic acids. The presence of functional groups such as aryl azides enhances specificity in covalent bond formation.
Moreover, the hydrophilic and hydrophobic regions in the structure affect solubility in various buffers. Greater solubility allows for better incorporation into biological systems and enhances the potential for successful crosslinking.
Reactivity and Stability
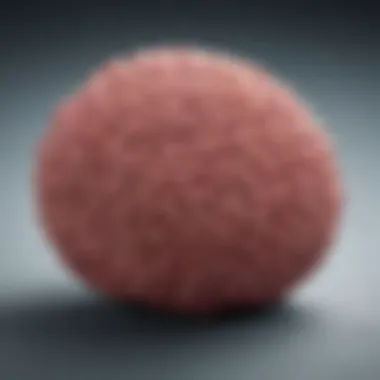
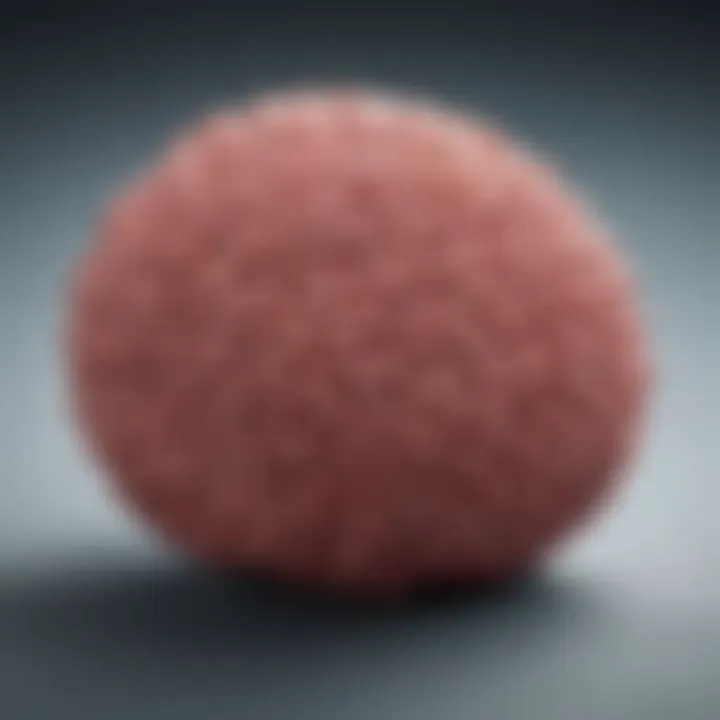
Reactivity and stability are highly significant factors when considering the application of dsso crosslinkers. The reactivity is primarily governed by the chemical functionality present in the dsso structure. The crosslinker's ability to form stable covalent bonds with target proteins or complexes under physiological conditions is vital. Precise control over reaction conditions, such as pH and temperature, can influence the outcome of crosslinking experiments.
Stability is equally vital as it ensures that the crosslinker remains intact throughout the experimental procedure. Factors such as light exposure, temperature variations, and buffer composition can affect its stability. In practical terms, researchers must optimize these conditions to maintain reactivity while minimizing degradation.
"The choice of crosslinkers can significantly influence the resolution and interpretation of protein interactions."
In summary, the molecular structure, reactivity, and stability of dsso crosslinkers are interrelated components essential for effective use in biochemical research. Investigating these properties allows scientists to tailor their experiments more precisely, maximizing the informative value of their findings.
Applications in Biochemical Research
The application of dsso crosslinkers in biochemical research is significant for advancing our understanding of molecular interactions. These unique compounds allow researchers to study the intricate relationships between proteins and other biomolecules. The ability to crosslink proteins opens numerous avenues in research, providing insights that are not easily achievable through other methodologies. Key applications include investigating protein-protein interactions, structural analysis of complexes, and cellular localization studies. Each of these applications offers distinct benefits and requires careful considerations.
Protein-Protein Interactions
dsso crosslinkers are especially useful for studying protein-protein interactions. They facilitate the formation of stable complexes between interacting proteins, which can then be analyzed. This stability is crucial, as it allows researchers to capture interactions that are typically transient and difficult to observe. By using dsso, scientists can identify specific binding partners and determine the nature of their interactions. This information is vital for understanding various cellular processes and mechanisms.
Moreover, this method has implications beyond basic research. It can aid in drug development by pinpointing potential targets within specific pathways. Understanding how proteins interact informs therapeutic strategies, potentially leading to more effective treatments for diseases.
Structural Analysis of Complexes
In structural biology, knowing the three-dimensional arrangement of proteins is fundamental. dsso crosslinkers enhance the ability to analyze protein complexes by providing structural constraints. When proteins are crosslinked and isolated, researchers can use techniques such as mass spectrometry or X-ray crystallography to determine their structure.
The accurate structural information obtained helps elucidate the function of the proteins involved. This is especially important when assessing the impact of mutations or post-translational modifications on protein function.
Cellular Localization Studies
Cellular localization studies benefit significantly from the application of dsso crosslinkers. By crosslinking proteins in situ, researchers can gain insights into where each molecule resides within the cell. This knowledge is crucial for understanding the roles proteins play in various cellular compartments and how their localization affects cellular function.
Real-time observations of protein placement are challenging; however, dsso allows for snapshots of dynamic systems. By understanding the spatial distribution of proteins, researchers can unravel the complexities of signaling pathways and cellular responses.
Methodologies Utilizing dsso Crosslinkers
The utilization of dsso crosslinkers in research methodologies is critical for advancing our understanding of biochemical interactions. By employing these crosslinkers, researchers can gain insights into the intricate networks of proteins and nucleic acids, providing a fuller picture of cellular functions. This section delves into the important aspects of experimental design, sample preparation, and analytical methods when using dsso crosslinkers, highlighting their impact on the quality of results and their interpretability.
Experimental Design Considerations
Designing experiments with dsso crosslinkers requires careful planning. One crucial element is the concentration of the crosslinker used, as this can significantly influence the binding efficiency and specificity. High concentrations might lead to non-specific crosslinking, whereas low concentrations may result in incomplete crosslinking. Therefore, it is essential to optimize concentrations based on the specific application and type of biomolecule involved.
Moreover, the choice of buffer conditions can affect the activity of dsso crosslinkers. It is advisable to select buffers that maintain a physiological pH and ionic strength to preserve the structural integrity of the target proteins during crosslinking. The timing of the crosslinking reaction is equally important; too short or too long can alter the experimental outcomes.
In summary, rigorous planning and understanding of experimental variables are vital for effective use of dsso crosslinkers.
Sample Preparation Techniques
The preparation of samples for crosslinking involves several steps that must be meticulously executed. First, proteins of interest should be purified to eliminate contaminants that might interfere with the crosslinking process. This purity ensures that the resulting data reflect the intended interactions without extraneous variables.
Additionally, the conditions under which proteins are incubated with dsso crosslinkers should be controlled. It may include varying temperature, incubation time, and the presence of reducing agents. For instance, some proteins may require denaturation before crosslinking to expose reactive sites. Typically, a controlled environment helps in achieving reproducible and reliable results.
Proper sample handling post-crosslinking is also crucial. This involves quenching the reaction promptly to prevent further undesired crosslinking and then formatting the samples for subsequent analyses, such as mass spectrometry.
Analytical Methods for Verification
Verifying the results obtained from experiments using dsso crosslinkers involves various analytical techniques. Mass spectrometry is often the preferred method due to its sensitivity and ability to analyze complex mixtures. It can identify crosslinked peptides and provide insights into the interaction networks of proteins.
In addition to mass spectrometry, techniques such as Western blotting and immunoprecipitation can also play significant roles in confirming crosslinking efficiency and specificity. These methods allow for the assessment of specific protein interactions before and after crosslinking, providing a clear comparison.
Next-generation sequencing may also be employed, particularly when studying nucleic acid-protein interactions. This method allows for comprehensive mapping of binding sites and understanding the extent of the crosslinking.
Proper verification of crosslinking outcomes is fundamental to the credibility of biochemical research.
Advantages of Using dsso Crosslinkers
The dsso crosslinkers bring significant benefits to biochemical research. Their unique properties contribute to a range of applications that improve data accuracy and reliability. Understanding these advantages helps researchers optimize their experimental designs and enhance the validity of their findings. This section will delve into two primary benefits: enhanced resolution of protein structures and increased sensitivity in detection.
Enhanced Resolution of Protein Structures
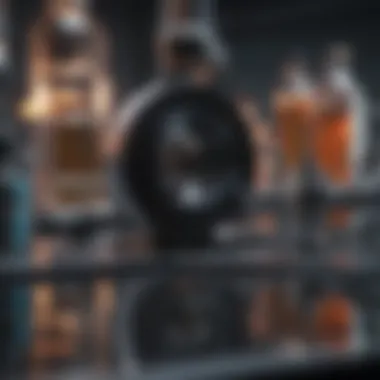
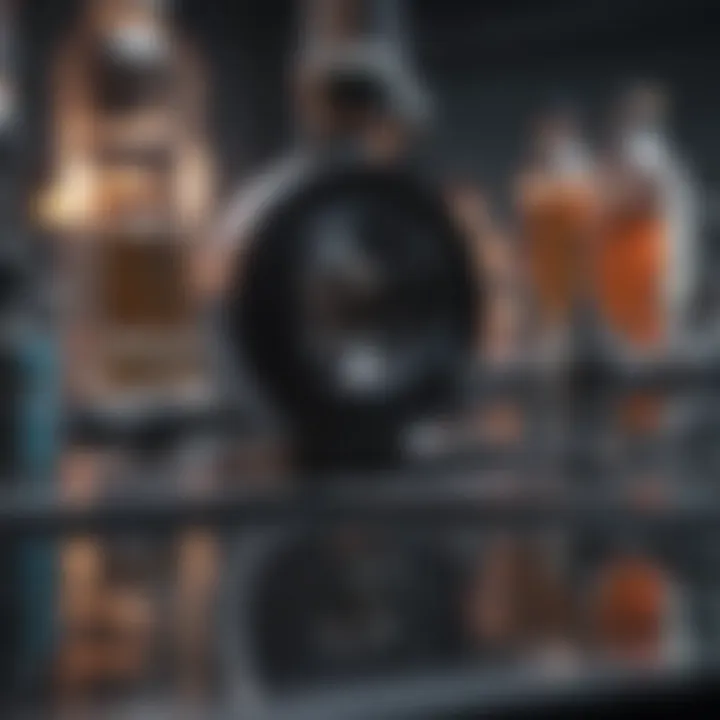
One of the standout advantages of using dsso crosslinkers is their ability to enhance the resolution of protein structures. When proteins interact, specifically designed crosslinkers bind different parts of these molecules together. This binding stabilizes the protein complexes, which makes it easier to resolve their three-dimensional configurations. In biochemical studies, accurate structural data are vital for understanding function.
The use of dsso crosslinkers allows for higher-resolution insights into protein conformation without significant alteration in their native state. Because of their specific reactivity and the formation of covalent bonds, researchers can obtain clearer data in methods such as X-ray crystallography and nuclear magnetic resonance (NMR) spectroscopy. The ability to capture transient interactions also cannot be overlooked, as it opens doors to studying complexes that might otherwise be too fleeting to analyze.
Increased Sensitivity in Detection
In addition to resolution, dsso crosslinkers significantly increase sensitivity in detection methods. The crosslinking efficiencies lead to stable complexes which makes them easier to track, even in complex biological samples. Researchers can detect lower abundance proteins and transient interactions, which are essential for deciphering signaling pathways, metabolic processes, and cellular mechanisms.
This heightened sensitivity is particularly advantageous in mass spectrometry, where even subtle changes in molecular weight or structure can yield vital information about protein interactions and modifications. It also aids in the identification of post-translational modifications that play critical roles in protein function. Therefore, incorporating dsso crosslinkers into experimental workflows can provide richer datasets, illuminating areas of research that may have remained obscure.
"The enhanced resolution and increased sensitivity offered by dsso crosslinkers position them as invaluable tools in the evolving landscape of biochemical research."
In summary, the dsso crosslinkers offer remarkable advantages that improve the clarity and detail of protein studies, expanding the horizons of what researchers can achieve. Their contributions towards enhanced structural resolution and superior detection sensitivity elevate the quality of data significantly, making them a superior choice in biochemical methodologies.
Limitations and Challenges
Understanding the limitations and challenges associated with dsso crosslinkers is crucial for researchers striving to maximize the potential of this compound in biochemical studies. While dsso crosslinkers represent significant advancements in the ability to study protein interactions and structures, they are not without complications. Researchers must consider the implications of non-specific crosslinking and potential sample degradation, both of which can adversely affect experimental outcomes.
Non-Specific Crosslinking
One major concern with using dsso crosslinkers is the tendency for non-specific crosslinking. This occurs when the crosslinker bonds proteins that are not intended to be linked. The result can obscure the intended interactions and yield misleading data. Non-specific interactions can arise from the structural attributes of proteins, as well as the experimental environment. It is vital to establish stringent conditions when applying dsso to minimize these non-selective reactions.
- Protein Concentration: High concentrations may increase the chances of non-specific interactions.
- Reaction Time: Extended reaction periods can also lead to unwanted crosslinking.
- Temperature: Keeping the reaction at optimal temperatures is essential to control crosslinking specificity.
Even though these issues can be addressed with proper methodology, they necessitate careful experimental design and validation.
Potential Sample Degradation
Another significant challenge in employing dsso crosslinkers involves potential sample degradation. This can occur due to the chemical nature of the dsso compound and the conditions under which experiments are conducted. Proteins can denature or degrade during the crosslinking process or subsequent analysis.
To mitigate these risks, consider the following:
- Buffer Systems: Employ stable buffer solutions that protect protein integrity during crosslinking and analysis.
- Reaction Conditions: Optimize pH and temperature to maintain protein stability.
- Timely Analysis: Minimizing the time between crosslinking and analysis can prevent degradation of sensitive samples.
Researchers must be vigilant in monitoring for signs of sample degradation to ensure that results are reliable and representative of true biological interactions.
"Understanding the limitations of dsso crosslinkers is essential for deriving accurate and meaningful data from biochemical research."
Future Directions in Crosslinking Research
The exploration of future directions in crosslinking research is pivotal for advancing our understanding of biochemical processes. The ongoing evolution of dsso crosslinkers brings forth numerous possibilities that may reshape the landscape of molecular biology. Increased innovation in this area can lead to significant improvements in how researchers study interactions at the molecular level, subsequently impacting drug discovery, disease understanding, and biotechnology applications.
Innovative Crosslinker Designs
Designing novel crosslinkers caters to specific needs in biochemical assays. Current dsso variants primarily serve general crosslinking purposes. Future designs should focus on enhancing selectivity for specific protein targets. By developing crosslinkers with greater specificity, it is possible to minimize non-specific interactions, which can confound experimental results.
- Targeted Modifications: Future designs may incorporate functionalities that enable selective binding to particular amino acid residues, thus reducing background noise in protein interaction studies.
- Dual-Action Crosslinkers: Concepts such as bifunctional crosslinkers that can bind multiple protein targets may enhance the ability to study complex protein networks.
- Responsive Crosslinkers: Designing crosslinkers that respond to environmental stimuli could provide dynamic insights into protein interactions under varying conditions.
Exploring these designs could potentially yield crosslinkers that are better suited to the complexities of live-cell environments, allowing researchers to capture real-time molecular interactions.
Technological Advances in Crosslinking Techniques
Technological advancements are essential for the full realization of dsso crosslinkers' potential. New tools, methodologies, and imaging techniques will likely enhance the effectiveness of crosslinkers in experimental setups. Significant areas for growth include the following:
- Mass Spectrometry Innovations: Enhanced techniques in mass spectrometry may improve the detection and characterization of crosslinked products, giving deeper insights into protein interactions.
- Imaging Techniques: Advancements in fluorescence microscopy and electron microscopy can aid in visualizing crosslinked proteins within living cells, thus providing clearer context for functional studies.
- Automation and High-Throughput Screening: Integrating automation into crosslinking protocols can optimize workflow efficiency and enable larger scale screening of potential crosslinkers, fostering rapid discoveries.
- Bioinformatics Integration: Utilizing computational models can help predict the outcomes of crosslinking experiments, refining the experimental designs based on computational simulations.
These technological strides present opportunities for researchers to delve deeper into biochemical interactions and protein structures, overcoming existing limitations in the field.
Comparative Analysis with Other Crosslinkers
The comparative analysis of dsso crosslinkers with other available options in the scientific community is crucial in understanding their unique advantages and disadvantages. It helps researchers choose the most suitable crosslinker based on their specific requirements. This analysis draws attention to fundamental characteristics such as reactivity, stability, specificity, and the scope of applications.
dsso vs. Traditional Crosslinkers
dsso crosslinkers present several distinctions when compared to traditional crosslinkers like glutaraldehyde and formaldehyde. One of the main advantages is the improved specificity of dsso. Traditional options often lead to non-specific crosslinking, which poses significant challenges in experiments, resulting in complicated data interpretation.
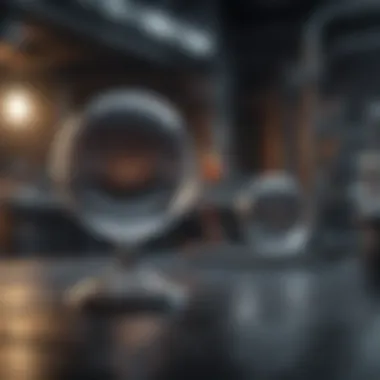
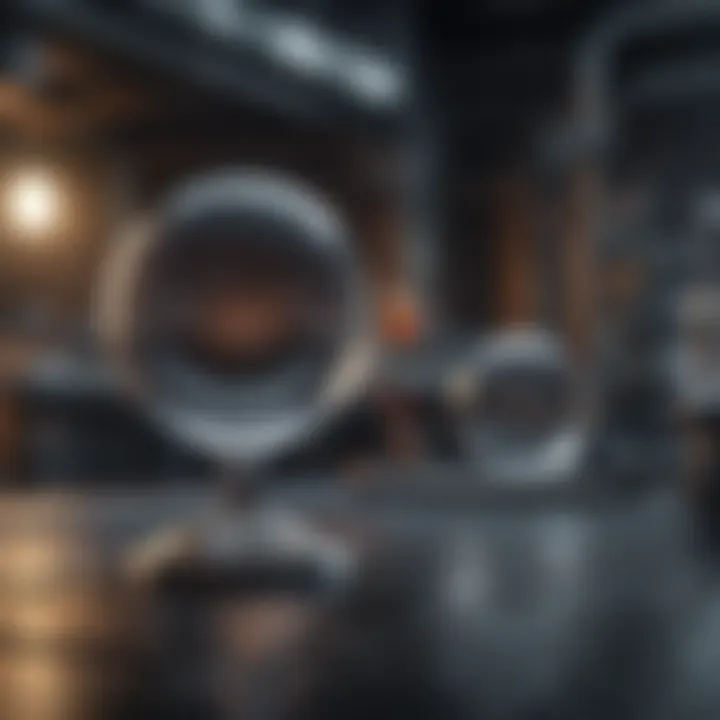
On the other hand, dsso crosslinkers are designed to minimize this issue, enabling a more accurate representation of protein interactions. Additionally, dsso tends to have a better tolerance for diverse experimental conditions, which makes it a compelling choice for various biochemical studies.
Furthermore, the introduction of dsso crosslinkers has highlighted the importance of selectivity in crosslinking methods, moving towards more refined techniques to study protein complexes. This specificity is particularly valuable in structural biology, where accurate models are crucial.
In terms of cost and accessibility, traditional crosslinkers can be more readily available. However, when considering the economic implications of potential misinterpretations from inaccurate data with traditional methods, the investment in dsso may counterbalance initial costs.
Hybrid Crosslinking Approaches
Hybrid crosslinking strategies, combining dsso with other crosslinkers, have emerged as innovative methodologies in biochemical research. These approaches leverage the benefits of multiple crosslinkers to enhance results while reducing associated limitations. For instance, using dsso alongside a traditional crosslinker could lead to improved detection of distinct protein interactions while ensuring better sample preservation.
Moreover, hybrid methods can optimize the crosslinking process, allowing for a wider range of experimental applications. The combination can help in achieving a comprehensive understanding of complex biological systems, depicting not only the structure but also the dynamic nature of protein interactions.
The development of hybrid strategies signifies a shift toward multilayered analysis in biochemical research. Researchers are exploring varied combinations to tailor their experimental setups for maximum efficiency and accuracy.
The ongoing evolution in crosslinker methodologies, including dsso and hybrid approaches, is critical for advancing our understanding of molecular interactions in biological systems.
Case Studies Utilizing dsso Crosslinkers
The study of dsso crosslinkers is greatly enhanced when practical case studies illustrate their application in real-world biochemical research. These case studies provide invaluable insights into how dsso crosslinkers facilitate the understanding of complex biochemical interactions and molecular structures. By examining specific instances of dsso use, researchers can glean important knowledge and design considerations for future experiments.
Insights from Recent Research
Recent research highlights the diverse applications of dsso crosslinkers across various biological systems. For instance, a study involving the dsso crosslinker in the analysis of protein-protein interactions in living cells demonstrated how these crosslinkers could yield higher resolution in the resultant data. Researchers found that using dsso allowed for effective retention of protein complexes, enabling them to map interaction networks more comprehensively. In particular, one study focused on the interactions of signaling proteins, revealing intricate networks that were previously hidden using traditional methods.
Moreover, dssoβs unique chemical properties offer researchers a specific set of advantages. The crosslinker's ability to react selectively with nearby amino acids has been shown to produce clearer results, making it easier to interpret data. A bioinformatics approach in one case study correlated the affinity data obtained from dsso-linked proteins with known interaction partners, enhancing the understanding of dynamic signaling in cellular environments.
Practical Applications in Biotechnology
The practical applications of dsso crosslinkers extend beyond basic research into the realm of biotechnology. For example, in the development of new therapeutic strategies, dsso crosslinkers have been employed to stabilize therapeutic protein complexes. This stabilization improves the efficacy of drug delivery systems and increases the half-life of biopharmaceuticals in circulation. Companies involved in monoclonal antibody development utilize dsso to ensure that their products maintain structural integrity during various formulation steps.
Several other fields within biotechnology also benefit from the use of dsso crosslinkers. These include:
- Vaccine development: Crosslinking helps maintain the conformation of antigens, enhancing immune response.
- Drug discovery: dsso enables better identification of biologically relevant targets in complex mixtures, streamlining the process of lead compound identification.
- Synthetic biology: Researchers apply dsso for assembling complex molecular machines from proteins, allowing for novel pathways in genetic engineering.
"The deployment of dsso crosslinkers is setting new benchmarks in biochemistry and biotechnology, fostering innovation that pushes the boundaries of what is achievable."
Impact on Scientific Collaboration
The dsso crosslinker plays a pivotal role in advancing scientific collaborations, particularly in the domain of biochemical research. As researchers increasingly recognize the importance of interdisciplinary approaches, dsso crosslinker serves as a bridge that facilitates interactions among diverse scientific fields. Its unique properties enable scientists from biology, chemistry, and physics to work together more effectively, resulting in a more comprehensive understanding of complex biochemical processes.
Facilitating Interdisciplinary Research
dsso crosslinkers enhance the ability of researchers to study protein interactions and molecular structures in a collaborative environment. By allowing the linking of various components in biochemical studies, dsso crosslinkers foster the integration of methodologies from different disciplines. For instance, a biochemist might partner with a physicist to explore structural aspects of proteins while employing unique techniques facilitated by the dsso crosslinker. This kind of collaboration improves the overall quality of research by combining expertise and perspectives from various scientific domains.
Moreover, the use of dsso crosslinkers often leads to more reproducible results in interdisciplinary studies. Enhanced resolution and specificity in tracking protein interactions significantly contribute to reliable outcomes, making it easier for teams to reach consensus on findings.
Encouraging Global Scientific Discourse
The implications of dsso crosslinkers extend beyond individual research teams; they encourage global scientific discourse. As more studies successfully utilize dsso crosslinkers, communication about methodologies and results becomes vital across industries and countries. Researchers sharing their insights and findings contribute to a larger conversation about biochemical research, promoting knowledge transfer and collaboration beyond geographical boundaries.
The ability to employ dsso crosslinkers creatively in experiments makes research more compelling and accessible to a wider audience. Researchers are more inclined to publish their findings and share data, resulting in an increase in communal knowledge. This trend emphasizes the importance of open science and collaboration, ultimately leading to accelerated advancements in the biochemical field.
In sum, the dsso crosslinker not only enhances individual research projects but also significantly impacts scientific collaboration on a broader scale. Its properties encourage a multidisciplinary approach, while its usage fosters global communication and knowledge sharing within the scientific community.
Endings
Concluding an article about the dsso crosslinker requires a thorough understanding of its implications in biochemical research. The dsso crosslinker serves as a vital tool for researchers seeking insights into molecular interactions and protein structures. Through comprehensive analysis and recent advancements, it has significantly impacted various areas within biochemistry.
Summary of Key Findings
The investigation into dsso crosslinkers reveals several key findings:
- Chemical Properties: The molecular structure of dsso crosslinkers allows for effective stabilization of protein interactions, enhancing the accuracy of biochemical assays.
- Applications: Their use in protein-protein interactions, structural analysis, and cellular localization has confirmed their importance in elucidating complex biological processes.
- Methodologies: Proper experimental designs and analytical methods using dsso crosslinkers have improved the reliability of data obtained from biochemical research.
- Advantages and Limitations: While dsso crosslinkers offer enhanced resolution and sensitivity, challenges such as non-specific crosslinking can complicate interpretations.
- Future Directions: The ongoing development of innovative crosslinker designs promises to address current limitations and expand the scope of applications in scientific studies.
Final Thoughts
The dsso crosslinker stands out in biochemical research for its potential to drive advancements in understanding molecular dynamics. Its contributions are not just limited to present applications but extend towards shaping future methodologies. Researchers must carefully consider both the advantages and limitations of dsso crosslinkers in their studies. With ongoing innovations, the field can expect new insights into protein interactions and structural biology. Ongoing scientific dialogue is essential to fully realize the potential of this powerful crosslinking tool.
"The will to comprehend and improve our molecular understanding shapes the future of biochemistry."
In summary, dsso crosslinkers are integral to advancing knowledge in various biochemical applications. Their roles continue to expand, indicating a promising future where new technologies will only increase their significance.