Exploring CRISPR-Cas9 and HDR in Genetics
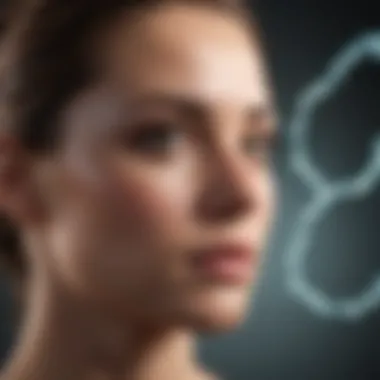
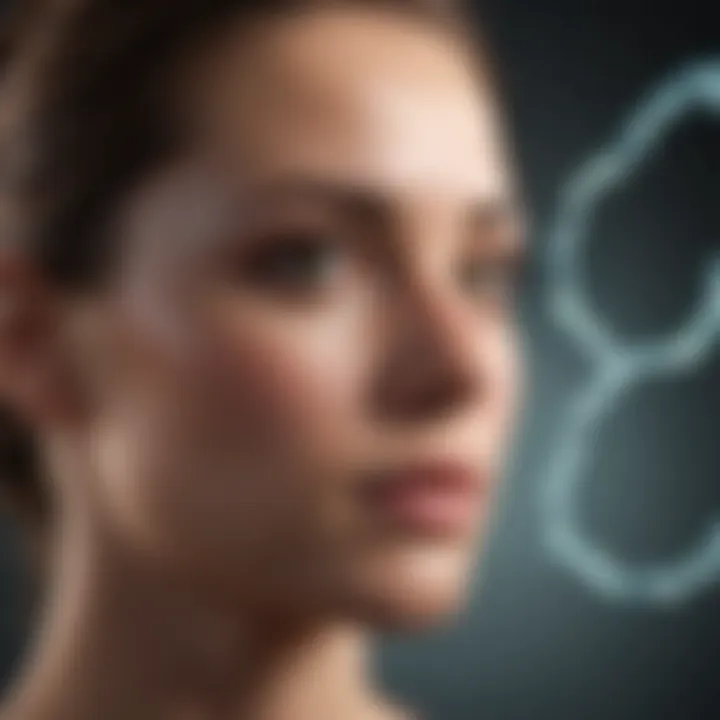
Intro
In the realm of genetic research, CRISPR-Cas9 and homology-directed repair (HDR) have emerged as pivotal technologies. Both play critical roles in advancing our understanding of genetics and opening new avenues in therapeutic practices. This article will thoroughly explore their mechanisms, applications, and the implications they carry in the modern scientific landscape.
CRISPR-Cas9, developed from the natural defense mechanisms of bacteria, allows for precise editing of DNA sequences. The technology is not only revolutionary due to its accuracy but also for its efficiency and ease of use compared to older genetic engineering tools. Together with HDR, which is a pathway for DNA repair that can precisely correct genetic errors, the duo presents a powerful method for creating targeted modifications in organisms.
As the field of genetics continues to evolve, it faces challenges. Ethical considerations are becoming more prominent, raising questions about the implications of manipulating genetic information. The potential for gene editing technologies to affect future generations adds a layer of complexity that demands careful thought. In evaluating CRISPR-Cas9 and HDR, it is vital to assess these technologies not only in terms of their capabilities but also their responsible use in society.
In the sections that follow, we will present a detailed overview of the current research in this area, highlight key findings, outline methodologies employed in studies, and address ethical considerations surrounding these powerful tools.
Preamble to Genetic Engineering
Genetic engineering has transformed biology, enabling precise modifications to genomes. This section introduces the foundational elements of genetic engineering, emphasizing the importance of understanding these principles in today's research landscape. The use of technologies for altering an organism's genetic material has profound implications in fields like medicine, agriculture, and environmental science. Genetic modification allows for the enhancement of desirable traits, understanding disease mechanisms, and even addressing ecological challenges.
The significance of genetic engineering is multifaceted. It offers solutions to pressing global issues, such as food security and human health. For instance, genetically modified organisms (GMOs) can be developed to resist pests or tolerate harsh environmental conditions, thus increasing agricultural yield. Similarly, the ability to edit genes in human cells can lead to breakthroughs in treating genetic disorders, opening pathways for personalized medicine.
However, alongside these benefits, genetic engineering presents ethical considerations. The potential for misapplication, the permanence of modifications, and long-term ecological impacts require careful examination. Therefore, a historical and scientific context is vital to navigate the complexities surrounding these technologies. As we delve into the specifics of CRISPR-Cas9 and homology-directed repair, it is crucial to appreciate the broader implications of genetic engineering that informs modern biological research.
Overview of Genetic Modification
Genetic modification involves changing the characteristics of an organism by altering its DNA. The techniques used in this field range from traditional breeding methods to modern genetic editing tools. Among these tools, CRISPR-Cas9 stands out due to its efficiency and precision. The ability to target specific sequences of DNA has revolutionized our approach to genetic research.
Genetic modification has various applications, including but not limited to:
- Agricultural Enhancements: Developing crops with better resistance to diseases, pests, or environmental conditions.
- Medical Therapies: Creating treatments for genetic disorders by correcting mutations at the DNA level.
- Biotechnological Innovations: Harnessing modified organisms for industrial processes, including biofuels and pharmaceuticals.
Moreover, ongoing research is continually expanding our knowledge base and enhancing our capabilities in genetic modification.
Historical Context of Genetic Techniques
To understand the current state of genetic engineering, it is important to look at its historical evolution. Early practices of genetic manipulation can be traced back to selective breeding in agriculture. However, the modern era of genetic techniques began in the late 20th century with the advent of recombinant DNA technology. This technique allowed scientists to combine DNA from different organisms, creating new genetic combinations.
Significant milestones include the development of polymerase chain reaction (PCR) in the 1980s, which enabled the amplification of specific DNA segments, and the mapping of the human genome, completed in 2003. These developments set the stage for the emergence of advanced tools like CRISPR-Cas9, which has become a focal point in modern genetic research due to its ease of use and versatility.
In the evolving landscape of genetic modification, understanding the history allows researchers, educators, and students to grasp the technological leaps made and the ethical discussions that have accompanied them. As we transition to exploring the intricacies of CRISPR-Cas9, this context will be essential for comprehending its transformative potential in genetics.
CRISPR-Cas9: A Breakthrough Technology
CRISPR-Cas9 signifies a paradigm shift in the realm of genetic engineering. This technology not only simplifies the process of gene editing but also increases the precision with which genetic modifications can be made. Its versatility allows researchers to target specific DNA sequences with unprecedented accuracy, making it a powerful tool in both basic research and applied sciences. The significance of CRISPR-Cas9 lies in its ability to facilitate advances in a variety of fields, such as agriculture, medicine, and biotechnology. By analyzing the mechanisms and applications of CRISPR-Cas9, one can appreciate its role in shaping the future of genetics.
Understanding CRISPR-Cas9 Mechanism
The CRISPR-Cas9 system operates by harnessing the natural defense mechanisms of bacteria against viruses. It employs a guide RNA that is programmed to match a specific target DNA sequence in an organism's genome. Once this guide RNA binds to the target, the Cas9 protein acts as molecular scissors, inducing a double-stranded break (DSB) in the DNA.
Upon creation of this DSB, the cell's repair mechanisms are triggered. This can lead to various outcomes based on whether the cell utilizes non-homologous end joining (NHEJ) or homology-directed repair (HDR). The first method often results in insertions or deletions, while HDR allows precise alterations through donor DNA templates. This duality presents an exciting range of possibilities for genetic modification and restoration of gene function, making the understanding of this mechanism crucial for advancing genetic therapies.
Components of CRISPR-Cas9
The CRISPR-Cas9 system comprises three main components:
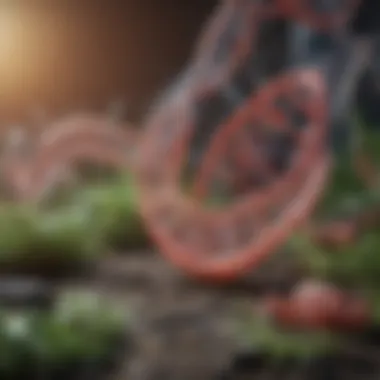
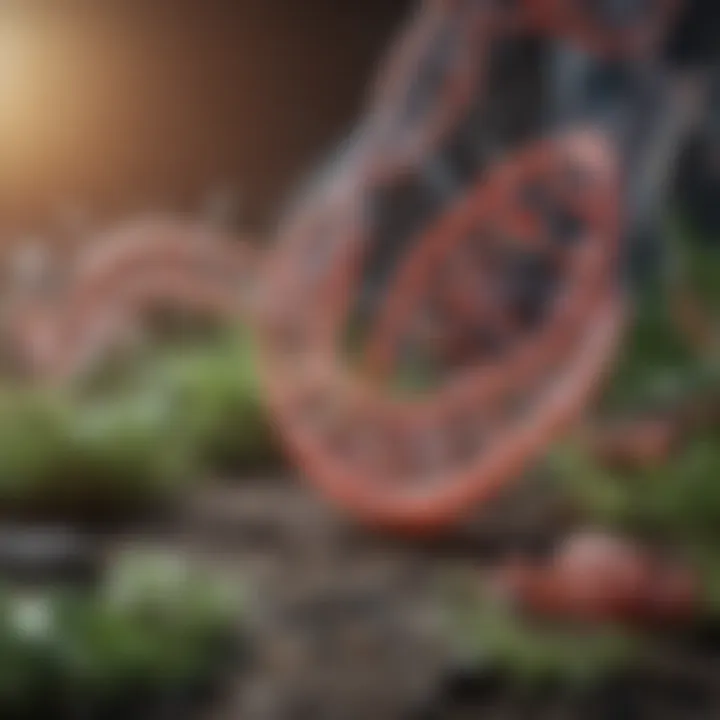
- Guide RNA (gRNA) - This RNA molecule pairs with the target DNA sequence. Its design is critical, as specificity determines the success of the gene editing.
- Cas9 Endonuclease - The enzyme component cuts the DNA. Its efficacy and precision can influence the outcomes of gene edits significantly.
- Donor DNA Template - This optional component is used during HDR. It provides the necessary genetic material for precise repairs or modifications.
These components work together seamlessly, making the CRISPR-Cas9 system efficient and adaptable for various applications in gene editing.
Applications in Gene Editing
The applications of CRISPR-Cas9 are vast and transformative. They include:
- Disease Research: Scientists use this technology to create models of diseases, enhancing understanding of the pathology.
- Therapeutic Development: Potential treatments for genetic disorders, such as sickle cell disease, are being explored through targeted edits.
- Agricultural Improvements: CRISPR is used to develop crop varieties with desirable traits, like drought resistance or pest tolerance.
- Gene Drives: This application aims to spread genetic modifications throughout populations, potentially controlling invasive species or eradicating diseases such as malaria.
The promise of CRISPR-Cas9 extends to the realm of synthetic biology, where it may help create novel organisms or biosystems with engineered properties.
Overall, CRISPR-Cas9 offers a toolkit that empowers researchers to explore genetic alterations that were once considered science fiction.
Homology-Directed Repair (HDR)
Homology-Directed Repair (HDR) is a fundamental process in modern genetics, acting as a crucial mechanism for DNA repair. Its importance in genetic engineering cannot be understated. Utilizing HDR, scientists can achieve intricate repairs within the genome, making it an invaluable tool for precise genetic modifications. Understanding HDR's intricacies allows researchers to effectively harness its capabilities in various applications like gene therapy and agricultural enhancements.
HDR stands out among other repair mechanisms due to its capability to utilize a homologous template for repair. This ensures not just randomness in DNA repair but a targeted restoration that maintains genomic integrity. Such precision opens avenues for correcting genetic disorders, enhancing crops, and even potentially curing diseases.
Mechanism of HDR
The HDR process involves a series of steps that are essential for its function. Initially, a double-strand break (DSB) occurs in the DNA, often induced by CRISPR-Cas9 technology. This break generates two DNA ends that require repair. Here are the core components involved in HDR:
- Recognition of the DSB: The cell's repair machinery identifies the break and processes the ends of the damaged DNA.
- Strand Invasion: Following recognition, a process called strand invasion occurs. Here, a single-stranded DNA segment, often provided as a template, invades one of the broken strands.
- DNA Synthesis: DNA polymerases synthesize new DNA using the template strand. This step allows for the insertion of desired genetic sequences into the genome.
- Resolution: Finally, the newly synthesized strands are resolved, restoring the integrity of the genome.
The efficiency of HDR can be influenced by several factors, including the design of donor templates, cell cycle stage, and the overall cellular environment. Researchers are continuously investigating ways to optimize these factors to enhance HDR efficiency in cellular systems.
Role of HDR in Genetic Repair
HDR plays a pivotal role in genetic repair and has profound implications for biotechnology. Among its most significant contributions is the correction of mutations that lead to genetic disorders. By utilizing precise templates, reproducible modifications can be made to the genome, addressing the root cause of inherited diseases. For example, a specific mutation in the Cystic Fibrosis Transmembrane Conductance Regulator (CFTR) gene could potentially be corrected using HDR technology.
Moreover, HDR acts as a powerful tool in the development of new therapies. By ensuring precision in gene editing, it mitigates risks associated with random integration events, which can disrupt other essential genes.
HDRβs precision makes it indispensable in the era of personalized medicine, where therapies can be tailored to individual genetic makeups.
In addition to therapeutic applications, HDR shows great promise in enhancing agricultural traits. Crop modification can produce plants with improved resistance to diseases and environmental stresses. This contributes to food security and sustainability in agriculture.
In summary, HDR is not merely a repair mechanism; it is a keystone in modern genetics. Its ability to achieve precise genetic edits positions it as a cornerstone for advancements across various fields, from medicine to agriculture, underscoring its significance in the ongoing evolution of genetic engineering.
Integration of CRISPR-Cas9 and HDR
The integration of CRISPR-Cas9 and homology-directed repair (HDR) represents a new epoch in modern genetics. This combination harnesses the precision of CRISPR technology alongside the inherent capabilities of HDR, significantly advancing gene editing potential. In this context, understanding how both tools work together is crucial for researchers and practitioners in the field.
CRISPR-Cas9 is responsible for creating double-strand breaks (DSBs) in DNA at targeted locations. This targeted precision is the backbone of its functionality, enabling specific genes to be cut with minimal off-target effects. Once these breaks occur, the cell undertakes its natural repair processes to mend the damaged DNA. This is where HDR comes into play. Unlike non-homologous end joining (NHEJ), which often results in random insertions or deletions, HDR utilizes a template to achieve precise repair. This allows for exact modifications in the genomic sequence.
The benefits of integrating CRISPR-Cas9 with HDR are numerous:
- Precision: HDR enables accurate insertion of new genetic material, reducing unintended changes.
- Efficiency: Combining these two methods can speed up the genetic editing process compared to using each technology alone.
- Versatility: The potential applications span various fields, including agriculture, biotechnology, and medicine.
However, there are considerations to keep in mind. The effectiveness of HDR relies on the phase of the cell cycle, meaning it works best during DNA replication when these processes are naturally active. Moreover, the competition between HDR and NHEJ can influence the success of a repair strategy. Researchers must account for this when designing experiments.
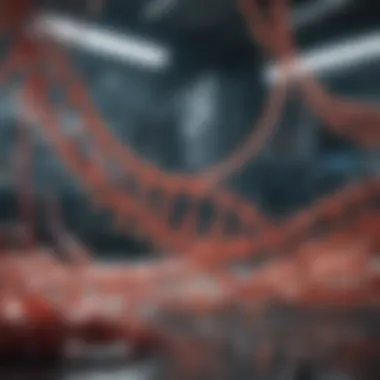
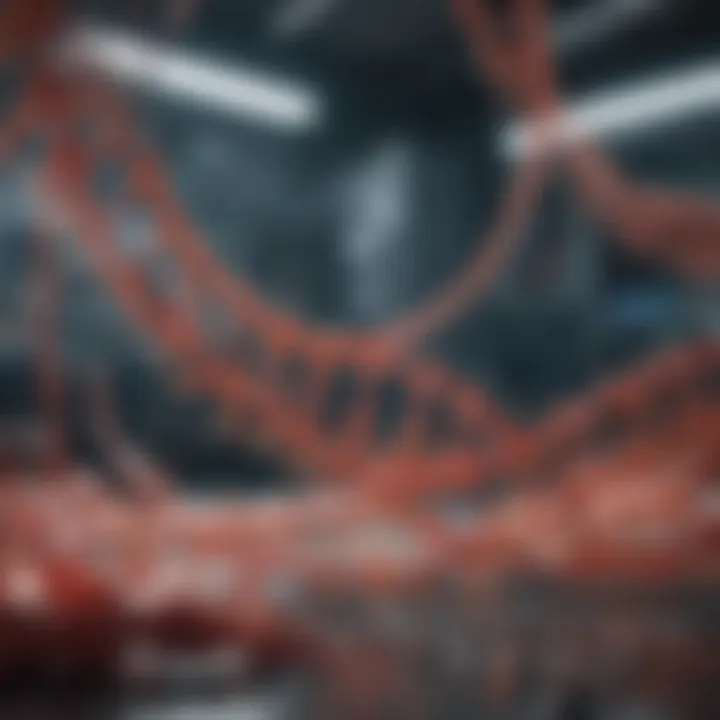
"The combination of CRISPR-Cas9 and HDR not only enhances genetic editing capabilities but also raises new questions about gene therapy possibilities."
In summary, the integration of CRISPR-Cas9 and HDR facilitates precise and efficient modifications in the genetic landscape, reinforcing the foundation for innovative research and therapeutic applications.
CRISPR-Cas9 Induced DSBs and Their Repair via HDR
The mechanism by which CRISPR-Cas9 induces double-strand breaks is pivotal for understanding its role in HDR. When the CRISPR system is introduced into a cell, the Cas9 enzyme, guided by RNA, binds to the target DNA sequence and creates a break. Following this, the cell can engage various repair pathways. The preference for HDR over NHEJ can be harnessed by providing a suitable repair template that matches the regions flanking the DSB. This allows researchers to insert or modify genetic sequences accurately.
To optimize repair through HDR, scientists often employ strategies to enrich the use of this pathway, such as synchronizing cell cycles or co-delivering factors that enhance HDR activity. By understanding how to manipulate the repair pathways effectively, future applications can become more reliable and predictable.
Potential for Precise Genetic Modifications
The potential for precise genetic modifications via the combined approach of CRISPR-Cas9 and HDR creates exciting opportunities in diverse fields. In medicine, this integration could lead to groundbreaking therapies for genetic disorders. The ability to replace or repair faulty genes with precision opens pathways to personalized medicine, enhancing treatment effectiveness while reducing side effects.
In agriculture, this technology can be applied to generate crops with desired traits, such as disease resistance or improved nutritional content, fostering food security in the face of global challenges.
Nevertheless, with this potential comes responsibility. Researchers must carefully assess the implications of such powerful tools. Dialogue about the ethical considerations, regulatory frameworks, and safety protocols is vital for responsible innovation.
Advantages and Limitations
Understanding the advantages and limitations of CRISPR-Cas9 and HDR is crucial in today's discussion of modern genetics. These tools represent significant advancements in genetic engineering, yet their application brings both opportunities and challenges. This section will provide a detailed overview of these dimensions, which is essential for researchers and practitioners in the field.
Benefits of CRISPR-Cas9 and HDR
The impact of CRISPR-Cas9 and HDR on genetic research is profound. One key benefit is the precision they offer. CRISPR-Cas9 allows for targeted editing of the genome, which increases the likelihood of successful modifications. This level of accuracy is essential for eliminating specific genetic disorders, making way for potential treatments that were unimaginable before.
Another advantage is the cost-effectiveness. Compared to traditional gene editing methods, like zinc-finger nucleases and TALENs, CRISPR-Cas9 requires fewer resources and simplifies the process of genetic manipulation. This reduction in costs enables laboratories with limited budgets to engage in cutting-edge research.
Furthermore, speed is a significant factor. Researchers can generate modifications more rapidly with CRISPR-Cas9 than with previous techniques. This acceleration is vital in fields like agriculture, where developing genetically modified crops to withstand climate change can occur more swiftly.
Challenges in Application
Despite the advantages, several challenges arise when applying CRISPR-Cas9 and HDR. One prominent issue is the technical complexity involved in the delivery mechanisms. Introducing these components into the target cells can be inefficient or inconsistent, leading to suboptimal results.
Moreover, researchers need a comprehensive understanding of the target genome. Inaccuracies in mapping can lead to unintended alterations, raising concerns over the reliability of genetic edits. The requirement for intricate design and validation steps can extend the timeline for research projects, complicating their implementation.
Off-Target Effects and Implications
Perhaps the most pressing limitation is the occurrence of off-target effects. These unintended modifications can lead to unintended consequences, which is a significant concern in therapeutic applications. The implications of these effects can be substantial, ranging from the introduction of new mutations to potentially harmful consequences in organisms.
"The precise targeting and editing afforded by CRISPR-Cas9 highlight its potential; however, the risk of off-target effects necessitates rigorous validation and ongoing research."
Researchers are actively exploring strategies to enhance precision, such as modifying guide RNA designs to minimize these risks. Yet, the inconsistency in results has raised ethical questions regarding the application of CRISPR technology in clinical settings.
Ethical and Regulatory Considerations
The rapid advancement of CRISPR-Cas9 technology and homology-directed repair (HDR) brings critical ethical and regulatory considerations to the forefront. This topic is vital in shaping how genetic engineering is perceived and applied in research and therapeutics. Ethical considerations address potential misuse of these technologies, while regulation plays a key role in ensuring safety and efficacy in applications.
Ethical Concerns in Genetic Editing
The ethical implications of genetic editing are profound. One major concern revolves around the concept of designer babies, where parents could potentially choose specific traits for their offspring. This raises questions about eugenics and the societal implications of such choices. The line between treatment and enhancement becomes blurred, leading to fears of inequality and genetic discrimination.
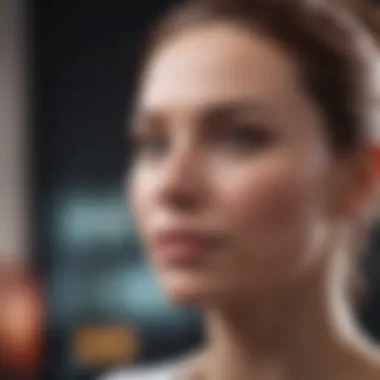
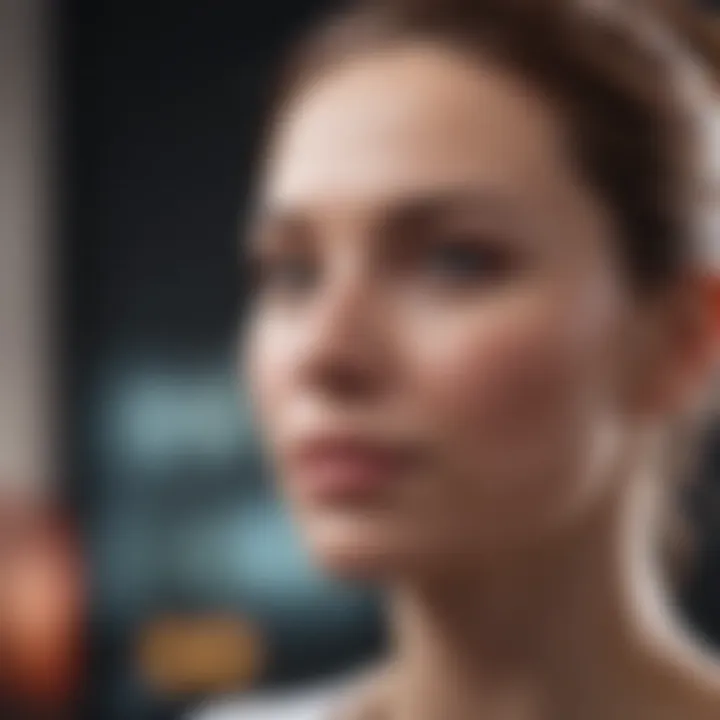
Moreover, the potential for unintended consequences cannot be overlooked. Off-target edits may result in unforeseen genetic issues, affecting not only the individual but their offspring as well. This inheritance of unintended edits raises significant ethical dilemmas about consent, especially when editing germline cells.
Another concern is the impact on biodiversity. As species are modified, there is potential for ecological imbalance, altering the genetic landscape of entire ecosystems.
"The ethical landscape of CRISPR technology is constantly evolving, demanding ongoing dialogue among scientists, ethicists, and policymakers."
Regulation of CRISPR Technologies
Regulatory frameworks are essential to manage the rapid integration of CRISPR technologies into society. There is a need for guidelines that delineate acceptable practices in research and clinical settings. Current regulations vary widely across countries, leading to a patchwork of oversight.
In the United States, the Food and Drug Administration (FDA) plays a role in evaluating gene editing therapies, while the National Institutes of Health (NIH) oversees many research projects. In contrast, countries like Germany have stricter laws that limit genetic modifications in humans.
Additionally, international collaboration is necessary to address the ethical and safety concerns globally. Organizations such as the World Health Organization (WHO) have started discussions on creating guidelines for gene editing technologies. This is crucial for fostering trust within the public and ensuring responsible innovation.
In summary, navigating the ethical and regulatory landscape of CRISPR and HDR is complex. Continuous dialogue among stakeholders, including scientists, ethicists, and public health authorities, is imperative to ensure that these powerful technologies are harnessed safely and ethically for the benefit of all.
Future Directions in CRISPR and HDR Research
The future of CRISPR-Cas9 and homology-directed repair (HDR) represents an evolving field in genetic research. Focus on these areas may yield significant breakthroughs, impacting various disciplines within the life sciences. The integration of CRISPR-Cas9 with HDR offers both practical and theoretical potential, guiding researchers towards novel therapeutic solutions for genetic disorders. Understanding these future directions could shed light on the innovations that lie ahead in addressing genetic conditions.
Innovations on the Horizon
New developments are continually emerging in CRISPR and HDR applications. Here are some innovative trends to watch for:
- Enhanced Precision: Researchers are focusing on improved specific targeting capabilities of CRISPR-Cas9. Techniques are emerging to minimize off-target effects, which is crucial for safety and efficacy in medical applications.
- Base Editing: This is a more refined method than traditional CRISPR. It allows conversion of one DNA base into another without introducing double-strand breaks. This could result in fewer unintended consequences during genome editing operations.
- Epigenome Editing: Manipulating the epigenome with CRISPR could provide non-invasive genetic modifications. This allows for reversible changes to gene expression without altering the DNA sequence itself, offering avenues to therapeutic strategies without permanent genetic alteration.
These innovations highlight a strong trajectory toward more sophisticated methods that enhance the potential applications of CRISPR-Cas9 and HDR in clinical settings.
Potential Impacts on Medicine and Therapy
The implications of CRISPR-Cas9 and HDR in medicine can be transformative. Their potential impacts include:
- Gene Therapy Advancements: Utilizing CRISPR-Cas9 with HDR may provide new techniques for correcting genetic mutations that cause diseases. This could lead to significant improvements in treating inherited disorders.
- Cancer Treatment: Researchers are exploring the use of CRISPR to edit immune cells, enhancing their ability to target and kill cancer cells. This approach may pave the way for personalized cancer therapies.
- Infectious Disease Management: CRISPR technology holds promise for combating viral diseases by targeting and disabling viral genomes within infected cells. This could lead to innovative strategies for controlling outbreaks.
As new research unfolds, it is essential to remain mindful of ethical and regulatory frameworks that ensure safe implementation of these technologies.
"Innovation in CRISPR and HDR holds the key to addressing some of the most pressing health challenges of our time, while navigating ethical implications remains a priority."
The End
The conclusion of this article emphasizes the significance of CRISPR-Cas9 and homology-directed repair (HDR) in modern genetics. These technologies are not merely advancements in genetic editing; they represent a paradigm shift that has the potential to redefine therapeutic approaches to a wide range of genetic disorders. Understanding both CRISPR-Cas9 and HDR enables researchers to harness their capabilities for more precise and effective genetic modifications.
Key benefits include their potential for precision and efficiency. With the ability to edit genes at specific locations in the genome, researchers can minimize off-target effects, enhancing the safety and effectiveness of therapeutic strategies. Furthermore, the integration of CRISPR-Cas9 with HDR can, theoretically, allow for not just the introduction of gene edits but also the correction of mutations that cause diseases.
However, important considerations remain. Ethical implications related to gene editing in humans necessitate a careful approach to both research and application. Regulatory frameworks are still under development to govern the responsible use of these technologies. Hence, scientists must balance innovation with ethical responsibility, ensuring that advancements do not outpace societal readiness.
"CRISPR technology and HDR are now recognized as essential tools, not just for genetic research but also for potential therapeutic applications that can tackle complex genetic diseases."
In summary, the conclusion articulates the intertwined future of CRISPR-Cas9 and HDR in the landscape of modern genetics. Their impact on the field is profound, and ongoing research will continue to uncover new possibilities and necessitate an ongoing dialogue about ethical and regulatory dimensions.
Summary of Key Points
- CRISPR-Cas9 and HDR Definition: CRISPR-Cas9 is a revolutionary tool for gene editing, while HDR is a natural DNA repair mechanism that enhances the precision of genetic modifications.
- Benefits of CRISPR-Cas9 and HDR: They allow for targeted edits with reduced off-target effects, paving the way for safer therapies.
- Challenges: Considerations related to ethics, regulation, and the need for continued research into their effects.
Final Thoughts on CRISPR-Cas9 and HDR
The journey of CRISPR-Cas9 and HDR in genetics is just beginning, and many more discoveries lie ahead. Researchers and the scientific community must remain vigilant about the implications of this technology as they work towards a future where genetic diseases can be accurately treated or even cured.