Exploring the Complexities of Plastics and Innovations
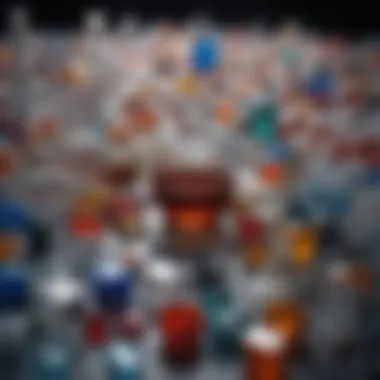
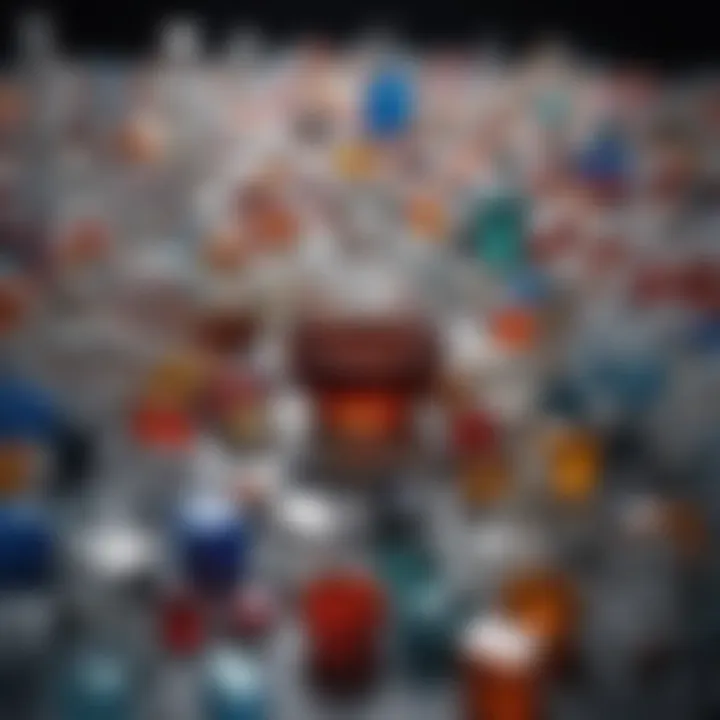
Intro
The realm of plastics represents a complex and ever-evolving field with significant implications for our daily lives and the environment. Plastics are not just materials; they embody a rich tapestry of scientific inquiry and innovation. From their diverse properties to their environmental impact, this article seeks to illuminate the multifaceted world of plastics.
Understanding plastics involves a closer look at their origins, properties, and uses. Plastics are synthetic materials made from polymers, which are long chains of molecules. Their versatility allows them to be molded into countless forms and shapes. However, this functionality comes at a cost. The widespread use of plastics has raised pressing environmental concerns, particularly regarding waste management and pollution.
The investigation of alternatives and recycling innovations is crucial. As research progresses, it highlights a growing necessity for sustainable practices to mitigate the adverse effects of plastics while capitalizing on their benefits.
This article aims to serve students, researchers, educators, and professionals seeking in-depth knowledge. It will synthesize critical advancements in research and illuminate ongoing efforts in recycling and alternatives, culminating in a comprehensive understanding of the current state of plastics across multiple disciplines.
Foreword to Plastics
The exploration of plastics encompasses a myriad of aspects, confronting both their utility and consequences. Plastics have become integral to countless applications across various sectors, from packaging to automotive solutions. Understanding how these materials are composed and their historical evolution is essential in contextualizing their role in modern society. The advancements in plastic technology have revolutionized many industries, offering both benefits and challenges that must be carefully evaluated.
In discussing plastics, it is crucial to address fundamental definitions and their complex makeup. This not only aids in comprehension but also fosters informed dialogue about their implications. Additionally, the historical context provides insight into how plastics emerged and transformed, highlighting milestones that have shaped current practices and innovations.
Definition and Composition
Plastics are synthetic materials made from polymersβlong chains of molecules created through chemical processes. These substances are derived primarily from fossil fuels, specifically petroleum. The composition of plastics varies widely, influenced by the intended application. Common types include polyethylene, polypropylene, polyvinyl chloride (PVC), and polystyrene. Each plastic type has distinct properties that dictate its usage, ranging from flexibility to durability.
The process of creating plastic generally involves polymerization, where monomers are chemically bonded to form these larger molecules. Additives such as colorants, stabilizers, and fillers may be incorporated to enhance performance characteristics. For instance, plastic products designed for outdoor use may contain UV stabilizers to prevent degradation from sunlight.
Historical Context
The history of plastics is relatively short yet impactful. The first synthetic plastic, Bakelite, was created in 1907 by Leo Baekeland, marking the beginning of a new era in material science. This groundbreaking development paved the way for the variety of plastics we see today.
Throughout the 20th century, the demand for plastics surged, driven by their versatility and cost-effectiveness. Major milestones include the introduction of nylon in the 1930s and the popularization of polyethylene in the 1950s. These materials quickly gained favor in manufacturing processes, leading to an array of consumer products that were once unimaginable. However, this rapid growth came with environmental repercussions, which are now at the forefront of discussions regarding sustainability and responsible plastic use.
Plasticsβ evolution mirrors society's changing needs and technological advancements. Their story is one of innovation and concern, requiring an ongoing dialogue about their future use and management in our world.
Types of Plastics
Understanding the types of plastics is essential in the discourse surrounding their applications and implications. Each type displays unique characteristics that influence how it is used in various contexts. Recognizing these differences allows for more informed choices in material selection, leading to better sustainability outcomes and optimized performance in intended uses.
Thermoplastics vs. Thermosets
Thermoplastics and thermosets represent the two primary categories of plastic materials, each with distinct properties.
Thermoplastics are types of plastics that soften when heated and can be reshaped upon cooling. This makes them highly versatile and amenable to recycling processes. Common examples include polyethylene and polypropylene. Their ability to be molded multiple times allows them to be used in a wide array of applications, from packaging materials to automotive components.
Advantages of Thermoplastics:
- Recyclable: Can be reshaped and reused, contributing to a circular economy.
- Cost-effective: Generally less expensive to produce than thermosets.
- Lightweight: They often weigh less than metal counterparts, which can assist in reducing transportation costs and energy consumption.
On the other hand, thermosets are plastics that undergo a curing process when heated. Once set, they cannot be remolded or reheated. This results in materials offering higher durability and thermal stability, which is valuable in industries such as aerospace and electronics. Phenolic and epoxy resins are prime examples of thermosets.
Considerations for Thermosets:
- Resilience: They exhibit superior resistance to high temperatures and chemicals.
- Stability: Once formed, they maintain their shape and integrity over time.
- Limited flexibility: Reworking these materials is impossible, which can be a drawback in recycling.
In summary, while thermoplastics allow flexibility and reusability, thermosets offer durability and stability. The choice between these types ultimately hinges on the specific requirements of an application.
Bioplastics and Their Applications
Bioplastics are derived from renewable sources, including plants and biomass, distinguishing them from conventional plastics that are petroleum-based. This category is gaining traction due to growing concerns about environmental sustainability. Unlike traditional plastics, which can take centuries to decompose, some bioplastics can break down in a shorter time frame through industrial composting processes.
The potential applications of bioplastics are vast. They can be utilized in industries such as packaging, agriculture, and consumer goods. For instance, polylactic acid (PLA) is commonly used in food packaging and disposable cutlery. This poses a less significant ecological footprint compared to its plastic counterparts.
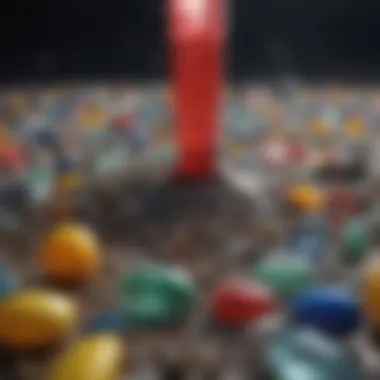
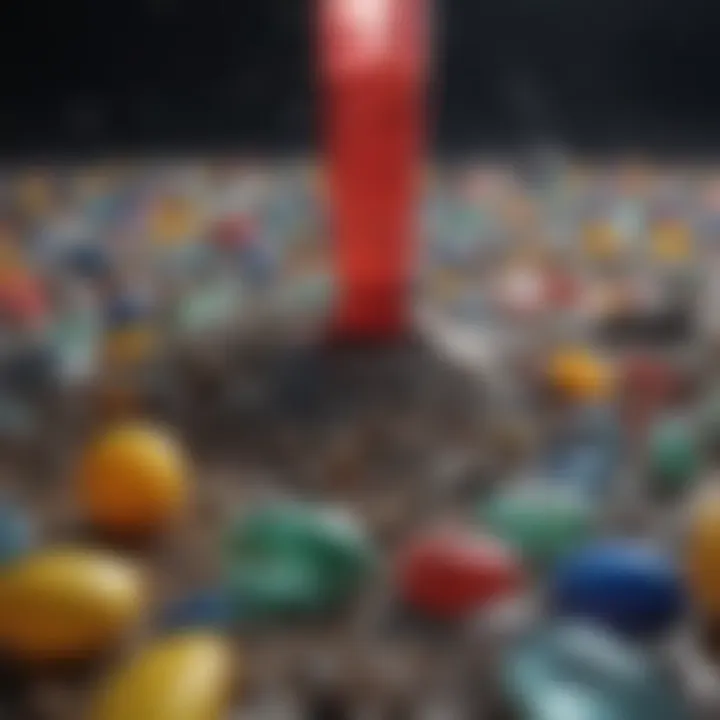
Benefits of Bioplastics:
- Sustainability: Use of renewable resources can reduce reliance on fossil fuels and lessen carbon emissions.
- Biodegradability: Many bioplastics are compostable, which can aid in reducing waste accumulation.
However, there are challenges associated with bioplastics. They can sometimes be more expensive to produce, which may hinder their widespread adoption. Furthermore, some bioplastics may still require specific conditions to degrade effectively, leading to confusion regarding disposal methods.
Properties of Plastic Materials
Understanding the properties of plastic materials is crucial for multiple reasons. These properties dictate not only the applications of plastics but also their performance, longevity, and environmental impact. Knowledge of mechanical, thermal, and chemical characteristics helps in selecting the right plastic for the intended use, thus enhancing efficiency and sustainability. This section investigates the key properties of plastics that make them unique and versatile.
Mechanical Properties
Mechanical properties of plastics encompass various attributes like tensile strength, elasticity, impact resistance, and hardness. Tensile strength measures the ability of a material to withstand stretching forces. This property is essential in engineering applications where strength is paramount.
- Tensile Strength: Plastics such as polycarbonate exhibit high tensile strength, making them suitable for demanding applications like safety glasses and electronic devices.
- Elasticity and Flexibility: Some plastics can stretch and return to original shape. Polyurethane is a prime example, known for its elasticity and shock absorbance.
- Impact Resistance: Many plastics resist breakage under sudden force, critical for packaging and safety applications.
These mechanical properties mean that plastics can be tailored specifically for applications that require resilience, flexibility or rigidity. Understanding which properties are vital for each application provides insight into product design and performance.
Thermal and Chemical Resistance
The thermal and chemical resistance of plastics is equally important for their lifespan and usability in various environments. Thermal resistance defines a plastic's ability to maintain its shape and properties when exposed to heat. Chemical resistance measures how well a plastic can withstand corrosive substances, which is vital in industrial applications.
- Thermal Stability: Polypropylene is renowned for its heat resistance, making it suitable for food containers that withstand microwaving.
- Chemical Resistance: Plastics like PTFE (Teflon) showcase exceptional resistance to a broad range of chemicals, commonly used in laboratory settings and industrial applications.
"Understanding the thermal and chemical resistances of plastics not only informs their practical applications but also guides safe handling and disposal methods."
Plastics in Daily Life
Plastics have become integral to our everyday existence, influencing how we live, work, and interact with the world. Their significance extends beyond mere convenience; they are foundational to a wide array of household and industrial applications, making them both ubiquitous and crucial. This exploration into plastics in daily life centers on how these materials shape our routines and the implications of their widespread usage.
Common Household Items
Many household items are made of plastic. These products range from storage containers to electronic devices and cleaning supplies. For example, containers like Tupperware are made of high-density polyethylene, which is durable and easy to clean. Plastic bottles are also common, as they lightweight and convenient for packaging beverages.
In addition, plastic is often used in kitchen utensils, such as spatulas and measuring cups, due to its ability to withstand high temperatures and resist breaking. Besides practicality, plastic materials can be cost-effective, making them accessible to many households.
However, the widespread use of plastic in these items also brings concerns. Environmental impact is a significant aspect, with single-use plastics causing pollution and waste management issues. Reducing reliance on disposable plastics in the household could benefit ecological health.
Industrial Applications
In the industrial sector, plastics play a significant role in manufacturing, construction, and packaging. For instance, in the automotive industry, plastics are favored for their lightweight properties, reducing fuel consumption while enhancing vehicle performance. Polypropylene and polyvinyl chloride are two common types of plastics utilized in various automotive components.
Moreover, plastics serve as protective materials for goods during transportation. Items like pallets and shrink wrap are made from plastics that help in preserving the integrity of products throughout the supply chain. This reduces waste and enhances efficiency in distribution.
Despite the advantages, the heavy reliance on plastic in industry raises critical questions about sustainability. As companies aim to improve their environmental footprints, many seek alternatives or ways to innovate their use of plastics. Implementing sustainable practices and materials could be key for the future of industrial applications.
"The reliance on plastic is a double-edged sword; while they offer numerous benefits, we must find means to reduce their environmental footprint."
In summary, plastics are essential in daily life, shaping how we manage our homes and industries. Understanding the balance between their utility and ecological responsibilities is crucial as we move forward.
Environmental Consequences of Plastics
The significance of understanding the environmental consequences of plastics cannot be overstated. Plastics have become ubiquitous in our lives, yet their effects on the environment are far-reaching and often detrimental. From their production, which inherently uses vast amounts of resources, to their disposal, which leads to pollution, plastic materials pose numerous challenges to ecosystems and human health. The focus on this topic is essential as it serves as a foundation for advocacy, policy change, and innovative solutions aimed at mitigating these impacts. Adopting a holistic approach in addressing these consequences will benefit society in the long term.
Pollution and Waste Management
Plastics contribute significantly to pollution across various environmental domains. A staggering volume of plastic waste enters oceans, rivers, and landscapes annually. This pollution is often the result of inadequate waste management systems, lack of recycling initiatives, and consumer habits of single-use plastics. The mismanagement of plastic waste can lead to several detrimental effects, including:
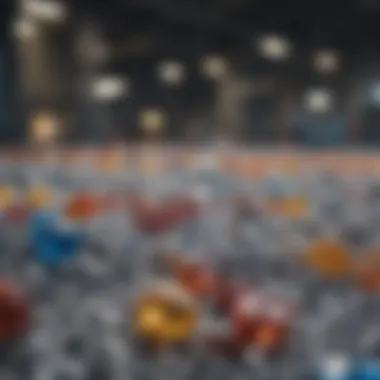
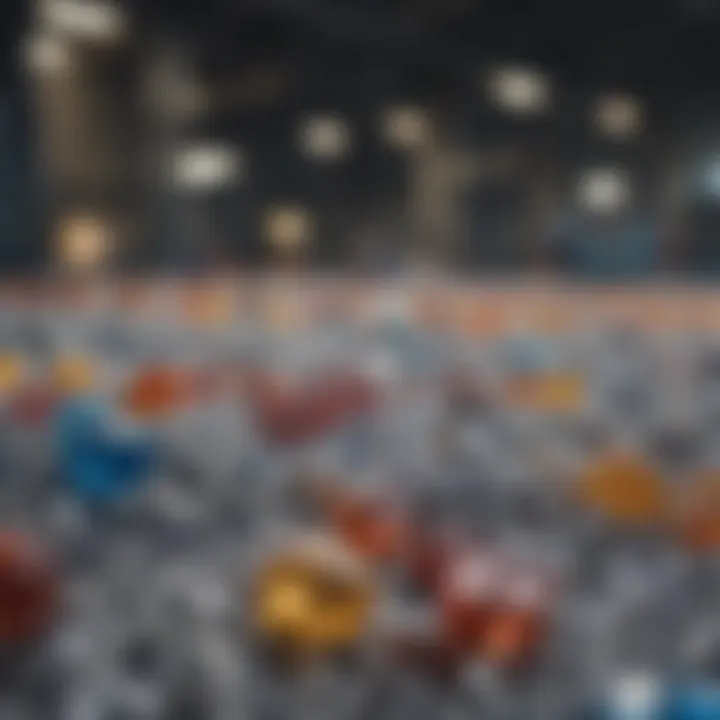
- Marine Life Threats: Many marine species ingest plastic debris, mistaking it for food. This can cause physical harm and reduce reproductive success, endangering entire species.
- Soil and Water Contamination: Over time, plastics break down into smaller microplastics that can leach harmful chemicals into the soil and water, affecting agricultural systems and drinking water supplies.
- Air Quality Issues: Burning plastic waste, common in many places, releases toxic substances into the atmosphere, posing health risks to those nearby and contributing to global air pollution.
To combat these issues, more effective waste management strategies need to be developed. A shift towards circular economy models, where plastics are reused and recycled, can drastically reduce the volume of waste generated. Enhanced public education regarding proper disposal and recycling practices is also crucial.
Microplastics and Ecological Impact
Microplastics, defined as plastic particles smaller than five millimeters, present a particular concern for ecosystems. Their pervasive nature means they have been detected in the most remote areas of the planet, including deep-sea environments and Arctic ice. Some critical aspects of microplasticsβ ecological impact include:
- Biodiversity Loss: Microplastics are ingested by a wide range of organisms from plankton to larger marine animals, leading to possible bioaccumulation throughout the food chain. This can disrupt entire ecosystems, altering species interactions and reducing biodiversity.
- Chemical Transfer: Microplastics can absorb pollutants from their environment, which may then be ingested by wildlife. This phenomenon poses risks to both animal and human health, as toxins may enter food supplies.
- Research Gaps: The long-term ecological consequences of microplastic exposure are still under investigation. More scientific research is needed to understand the full extent of their impact on various species and overall ecological health.
"Tackling the issue of microplastics requires collaboration among scientists, policymakers, and the public to develop effective management strategies."
The Science of Recycling Plastics
Recycling plastics plays a crucial role in managing waste and reducing the environmental burden associated with plastic production and disposal. As the global community begins to recognize the detrimental effects of plastic pollution, understanding the science behind recycling becomes vital. This section examines the methods currently employed in recycling plastics, the inherent challenges, and what they mean for our future.
Current Recycling Processes
Recycling plastics involves a series of complex steps. These steps enhance the recovery of valuable materials and minimize the need for virgin resources. Here are common processes used today:
- Collection and Sorting: This is the first step in the recycling process. Plastics are collected from various sources, including households, businesses, and recycling centers. Once collected, the materials are sorted based on resin types, which typically include PET, HDPE, PVC, LDPE, PP, and PS. Sorting is critical because different plastics have distinct properties and recycling methods.
- Washing and Cleaning: Contaminants such as food residue, labels, and dirt are removed from the sorted plastics. Effective cleaning is essential to ensure that the recycled material meets quality standards required for future applications.
- Shredding: Cleaned plastics are then shredded into smaller pieces. This attribute aids in uniform processing and enhances the efficiency of subsequent stages, such as melting.
- Melting and Extrusion: The shredded plastics are heated until they melt. The molten plastic is then pushed through a mold to create pellets. These pellets can be used to manufacture new plastic products.
- Quality Control: Residual contaminants are checked during quality control. This process ensures that the recycled plastics can be reused effectively and meet industry standards.
These processes highlight the technological advancements in plastic recycling. However, even with these improvements, challenges remain.
Challenges in Recycling
Despite the established recycling processes, various challenges hinder effective plastic recycling. Understanding these obstacles is essential for developing future strategies:
- Economic Factors: The cost of recycling plastic often exceeds the price of producing new plastic from virgin materials. This economic pressure leads many companies to favor cheaper, new plastics over recycled options.
- Material Contamination: Contamination during collection and sorting cause significant issues in recycling. Non-recyclable materials mixed with recyclable plastics can compromise the entire batch, leading to increased waste.
- Market Demand: There is limited demand for recycled plastics in some industries. Without a robust market for recycled materials, the incentives to invest in recycling technologies diminish.
- Variability in Consumer Behavior: Consumer practices regarding the disposal of plastics can vary widely. Some individuals may not properly recycle or dispose of plastic products which can lead to increased contamination and reduced rates of recycling.
"Addressing the challenges of recycling is as crucial as enhancing its processes. Effective solutions required a multifaceted approach, including regulatory incentives, public awareness, and technological advancements."
Through overcoming these challenges, the recycling of plastics can become more viable and innovative, contributing to the overall health of our environment. As we explore further advancements and solutions in the field, it becomes clear that understanding the science of recycling is integral to creating a sustainable future.
Innovations in Plastic Alternatives
Innovations in plastic alternatives represent a critical development in our ongoing quest for sustainability. As concerns about plastic pollution escalate, particularly in marine environments and urban landscapes, exploring viable alternatives becomes essential. This section breaks down recent advancements and research, with the objective of presenting a path toward reducing dependency on conventional plastics. The focus here is on how these innovations not only address ecological challenges but also stimulate new economic opportunities and technological advancements.
Research and Development of Alternatives
Research into plastic alternatives has accelerated in recent years, reflecting an enhanced awareness of environmental issues. Scientists and researchers are actively exploring various materials that can mimic the properties of traditional plastics without contributing to pollution.
- Biopolymers: These are derived from renewable resources like corn starch, sugarcane, or even seaweed. Unlike petroleum-based plastics, biopolymers are biodegradable, which can help mitigate waste problems. Some successful examples include polylactic acid (PLA) and polyhydroxyalkanoates (PHA).
- Mushroom-based materials: Researchers have developed innovative packaging solutions made from mycelium, the root structure of mushrooms. This organic material can be cultivated and shaped into forms that are not only lightweight but also biodegradable.
- Recycled materials: Ongoing advancements are being made in refining processes for using post-consumer plastics to create high-quality alternatives. This includes recycling technologies that can break down plastics into their base molecules, allowing them to be reused effectively in production.
The commitment to R&D in this area is evident. Many universities and corporations are establishing dedicated initiatives to study alternative materials, thereby potentially transforming multiple industries.
Emerging Materials and Their Potential
The emergence of new materials poses exciting possibilities for the future. These innovative solutions are being designed with sustainability at their core and aim to replace conventional plastic products.
- Cellulose-based materials: These materials are derived from plant fibers and present numerous benefits. They are biodegradable and come from abundant sources. Cellulose films, for example, can replace single-use plastics in packaging applications.
- Algae-based plastics: Algae have been recognized as a rich source of raw material for bioenergy and other products. Recent studies explore how to convert algae into bioplastics that would require fewer resources in terms of water and land for production, compared to traditional crops.
"The transition to alternative plastics is not just about the materials themselves; it embodies a fundamental change in how we think about consumption and waste in our society."
- Composite materials: This category includes formulations that combine natural fibers, like hemp or bamboo, with bioplastics. Not only do they possess a lower carbon footprint, but they also provide high strength and durability for various applications.
Regulatory Frameworks and Policies
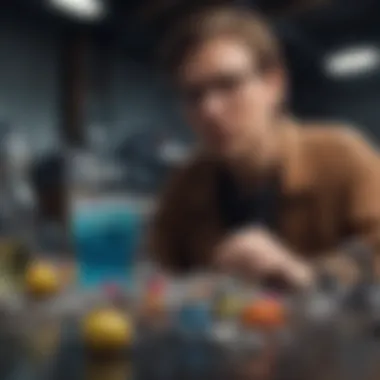
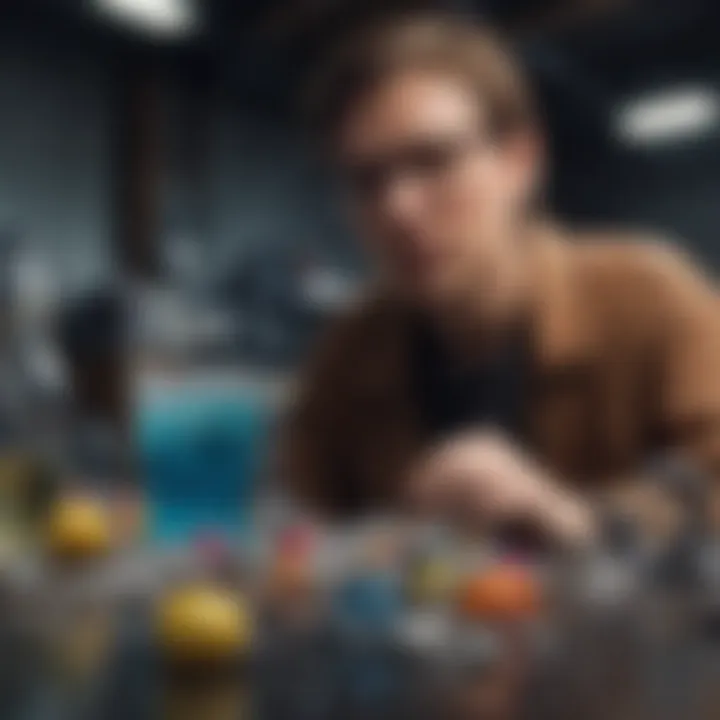
Understanding the regulatory frameworks and policies surrounding plastics is vital. These regulations shape how plastics are manufactured, used, and disposed of. They also have significant implications for public health and the environment. In this section, we examine local and global regulations that govern the plastic industry. A clear understanding of these policies can aid in developing new strategies that minimize adverse effects on the ecosystem while still supporting economic growth.
Local and Global Regulations
Local regulations often reflect the social, economic, and environmental priorities of a community. They can dictate the types of plastics allowed in certain applications, such as food packaging or single-use plastics. For example, many cities have enacted bans on plastic straws or bags to combat pollution. Local governing bodies may also impose requirements for plastic waste management, such as mandating recycling programs.
On the other hand, global regulations aim to standardize practices across borders. Organizations like the United Nations and the European Union have set frameworks for managing plastic pollution. The EUβs Directive on Single-Use Plastics, for example, significantly limits the use of certain disposable plastic products to reduce marine litter. These regulations often foster cooperation among countries and encourage the sharing of best practices.
"The regulation of plastics is not only a matter of environmental sustainability but also a critical component in public health and safety."
Impact of Policy Changes on Plastics Usage
Policy changes can have profound impacts on how plastics are utilized in various sectors. When stricter regulations are introduced, industries often must adapt. This can prompt innovations in material alternatives and recycling processes. For instance, if a government restricts the use of a certain type of plastic, businesses may invest in research for substitutes, leading to the development of biodegradable materials or enhanced recycling methods.
Conversely, lenient regulations can result in increased plastic usage that may lead to environmental degradation. Inconsistent regulations across different regions can further complicate this issue, making it challenging for businesses that operate internationally. An increase in regulations can also provoke discussions about economic implications, such as potential job losses in traditional plastic manufacturing sectors.
Regulatory frameworks thus play a critical role in shaping not just the production but also the lifecycle management of plastics. Adapting to these changes is essential for sustainability and innovation in the industry.
Future of Plastics
The future of plastics is a critical topic within the broader discussion of materials science and environmental sustainability. As the global production of plastic increases, so does the pressure to find effective methods for improving their lifecycle and reducing adverse environmental impacts. Understanding the future of plastics involves considering several facets, including advancements in technology, regulatory changes, and the urgent need for sustainable practices. It is vital for researchers, policymakers, and industry leaders to engage in this conversation to ensure that the direction taken contributes positively to our environment and society.
Trends in Research and Technology
Research on the future of plastics is rapidly evolving. Various trends reveal a promising shift towards innovation. One key development is the increase in bioplastics. These materials, derived from renewable resources such as corn starch or sugarcane, can potentially minimize reliance on fossil fuels. Researchers are also exploring methods to enhance the biodegradability of traditional plastics. New additives can make plastics degrade more quickly when exposed to specific environmental conditions.
Another significant trend is the improvement of recycling technologies. Advanced methods such as chemical recycling allow us to break down plastics into their basic components. This process can then produce high-quality raw materials that can be used to create new products. Furthermore, innovations in sorting technologies are revolutionizing the recycling process, making it more efficient and effective.
"Investing in research and development of new materials will shape the future of how we interact with plastics in our daily lives."
Additionally, there is a growing trend in additive manufacturing, commonly known as 3D printing. This technology not only allows for the efficient use of materials but also produces items on demand, potentially reducing waste. Universities and research institutions are at the forefront of these advancements, providing insights into better material properties and applications.
Sustainable Solutions for the Industry
Sustainability is no longer an option; it is a necessity for the future of the plastics industry. Companies are starting to adopt sustainable practices right from the design phase. Concepts like circular economy are gaining traction, promoting the idea that materials should be reused and recycled rather than discarded. There is a shift towards designing products with their end-of-life in mind, making it easier to recycle or repurpose them after use.
Several industry leaders have begun adopting eco-friendly raw materials. Companies like Unilever and Coca-Cola are committing to using recycled plastics in their packaging. The push for transparency in supply chains is growing, encouraging brands to disclose their material sources and sustainability practices.
Additionally, policies are being developed to encourage responsible plastic use. Initiatives, such as bans on single-use plastics in various regions, aim to encourage companies and consumers to consider more sustainable alternatives.
The future of plastics presents both challenges and opportunities. By embracing research, innovation, and sustainability, the industry can effectively address pressing environmental issues. As we move forward, collaboration among scientists, policymakers, and manufacturers is essential to drive meaningful change.
Finale
The conclusion of this article is pivotal in encapsulating the multi-faceted nature of plastics. It serves to synthesize the insights gained from the earlier sections and emphasize their relevance to scholars, industry professionals, and policymakers.
In summary, the discussion around plastics revolves around their extensive applications and significant environmental implications. By highlighting various aspects, from types of plastics to their recycling processes, we create a holistic view of their role in modern life. This overview does not merely catalog information but rather prompts a critical examination of the lifecycle of plastic materials.
Summary of Key Points
- Definition and Composition: The foundational elements that categorize plastics and their varying properties.
- Types of Plastics: The distinction between thermoplastics and thermosets, and the potential of bioplastics.
- Environmental Impact: An in-depth look at pollution, waste management, and the emerging concern about microplastics.
- Recycling Science: Current processes in recycling, alongside the challenges faced.
- Innovations: Ongoing research into plastic alternatives and new materials that could reshape industry standards.
- Regulatory Policies: The impact of global and local regulations on the usage of plastics and the response strategies from industries.
- Future Trends: Emerging technologies and sustainable solutions that can direct how plastics are handled in the future.
This summary highlights the interconnectedness of these elements and underscores the urgency for informed discussions on plastics.
Call to Action for Continued Research
The landscape of plastics necessitates further scrutiny. While significant advancements have occurred in the understanding and management of plastics, ongoing research is vital. Areas needing focus include:
- Decomposition Methods: Developing methods to efficiently break down plastics and mitigate their environmental impact.
- Innovative Recycling Techniques: Finding more effective ways to recycle a wider variety of plastics.
- Alternative Materials: Investigate innovative materials that can serve as substitutes for traditional plastics in various applications.
- Health Impacts: Studying the long-term health effects of plastic exposure and the constituents in bioplastics.
Continuous study will lead to better solutions, not just in managing current plastic waste but also in developing smarter, more sustainable alternatives.
By encouraging collaboration between researchers, industries, and policymakers, we can foster innovations that drive change.
Emphasizing an understanding of the multifaceted nature of plastics can lead to developing balanced solutions that align with ecological and societal needs.