Exploring Carbon Capture Science: Mechanisms and Challenges
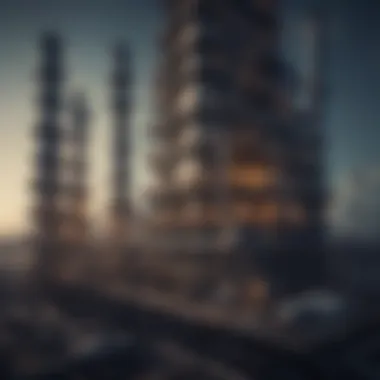
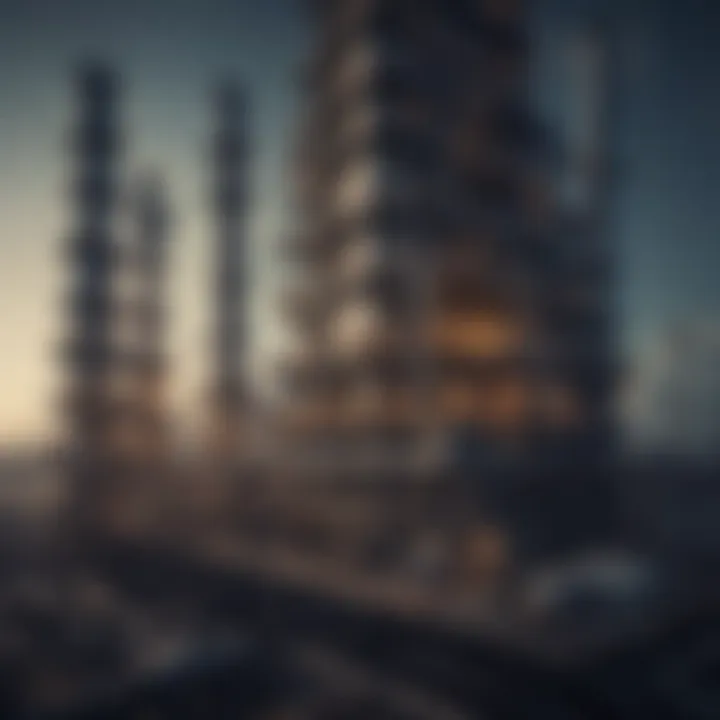
Intro
Carbon capture science stands at a pivotal intersection of technology, environmental policy, and sustainability. Amidst growing concerns over climate change, understanding how to effectively remove carbon dioxide from the atmosphere is increasingly essential. This article aims to unravel the complexity of carbon capture, detailing the various mechanisms, addressing the challenges that come with them, and evaluating the wider implications for our environment and future climate strategies.
The journey into carbon capture commences with a review of its key technologies and methodologies. From direct air capture to more traditional methods like afforestation, each approach contributes uniquely to mitigating carbon concentrations in the atmosphere. However, no innovation comes without its own set of challenges. Implementing these technologies in a manner that is both economically viable and scalable poses significant hurdles.
By analyzing current research and innovations, we provide readers with a deep understanding of how carbon capture can play a critical role in the fight against global warming. This exploration also extends into climate policy, attributing importance to carbon captureβs potential benefits and challenges in shaping a sustainable environmental future.
Research Overview
Summary of Key Findings
The primary understanding established through recent research reveals the effectiveness of various carbon capture technologies varies significantly. Techniques such as carbon capture and storage (CCS) and negative emission technologies (NETs) show promise in potentially reducing atmospheric CO2 levels when implemented correctly.
Research Objectives and Hypotheses
This section aims to decode how various methodologies impact overall carbon capture efficiency. The hypothesis suggests that while advanced technologies offer improved metrics in capturing carbon, significant challenges, including cost and infrastructure requirements, still hinder widespread adoption.
Methodology
Study Design and Approach
The methodology for exploring carbon capture technologies involves a comprehensive review of existing literature and current practices. This approach ensures a robust understanding of what has been tried, what works, and where gaps still exist in the research.
Data Collection Techniques
Data have been collected from multiple reputable sources, including peer-reviewed journals, environmental databases, and institutional reports. This range of sources provides a well-rounded perspective on the effectiveness, challenges, and future directions in carbon capture technology.
"Understanding carbon capture technology is crucial in navigating our approach to climate change mitigation."
In compiling this information, this article sets the groundwork for a nuanced understanding of how carbon capture technologies can evolve and contribute to sustainable climate solutions. It will elaborate on the significance of carbon capture mechanisms, the existing challenges, and potential implications for policymaking. Readers will come away with a knowledge that transcends the surface, diving deep into a pressing issue impacting our world today.
Foreword to Carbon Capture Science
Carbon capture science is an essential field in the ongoing efforts to mitigate climate change. It encapsulates a variety of mechanisms aimed at reducing carbon dioxide levels in the atmosphere. Understanding this area is crucial for developing effective strategies that combat global warming. The pressing need for carbon mitigation solutions is underscored by the increasing levels of carbon emissions from industrial and everyday human activities.
Efforts in carbon capture not only aim to reduce atmospheric carbon but also have the potential to influence broader environmental policies. These approaches can lead to cleaner air and potentially sustainable practices in various industries. Addressing carbon capture holistically is a priority for researchers, educators, and policymakers alike.
Definition and Importance
Carbon capture refers to a set of technologies that can extract carbon dioxide from sources like power plants and the atmosphere itself. This process is vital for achieving climate targets set by international agreements. By efficiently removing CO2, these technologies can help stabilize global temperatures and potentially reverse some effects of climate change.
The importance of carbon capture lies in its dual role; it not only helps in reducing current emissions but also opens avenues for negative emissions strategies. Negative emissions are methods that can remove more carbon dioxide from the atmosphere than is being emitted. This creates a significant opportunity for mitigation efforts globally, emphasizing the relevance of carbon management practices.
Historical Context
The concept of carbon capture has evolved significantly over the decades. Initially, carbon dioxide was regarded mostly as a pollutant. In the early days of industrialization, little was done to capture or manage these emissions. The first significant efforts began in the 1970s when researchers started exploring ways to separate CO2 from emissions during energy production.
By the 1990s, projects like the Sleipner project in Norway showcased the feasibility of carbon capture and storage. This landmark initiative effectively stored CO2 in geological formations beneath the North Sea. Such early examples have paved the way for further advancements in the field. Now, academic institutions and companies worldwide are investing in research and development to refine methods for capturing and utilizing carbon in transformative ways.
"Understanding the significance of historical context is vital for appreciating the development and future of carbon capture technology."
Through an examination of the progress made, it becomes evident that while challenges remain, the path laid by previous efforts provides valuable insights and frameworks that inform ongoing research and implementations.
Fundamental Principles of Carbon Capture
Understanding the fundamental principles of carbon capture is essential for grasping how this technology can contribute to mitigating climate change. This section discusses the chemical and physical foundations that allow carbon dioxide to be efficiently captured and stored, ensuring a comprehensive overview for students, researchers, educators, and professionals.
Chemical Principles
The chemical principles underlying carbon capture involve various reactions that allow carbon dioxide to be separated from other gases. One prominent mechanism is chemical absorption, where carbon dioxide reacts with a solvent to form a chemical compound. The most common solvents are amines, which are nitrogen-containing organic compounds. When carbon dioxide comes into contact with an amine solution, it forms a carbamate. This reaction is reversible, meaning the captured carbon dioxide can be released when conditions change, allowing for regeneration of the solvent.
Another crucial aspect is sorbent materials, which can capture carbon dioxide through chemical reactions. These materials usually have high surface areas and can be engineered to enhance their affinity for carbon dioxide. Their use in fixed-bed systems or in more advanced frameworks like metal-organic frameworks (MOFs) can significantly improve the efficiency of capture processes.
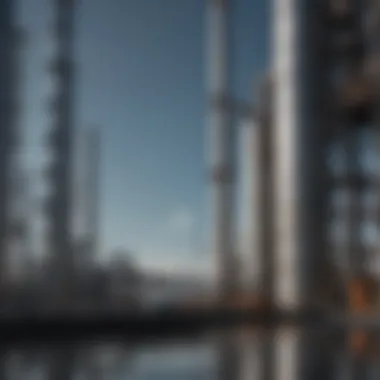
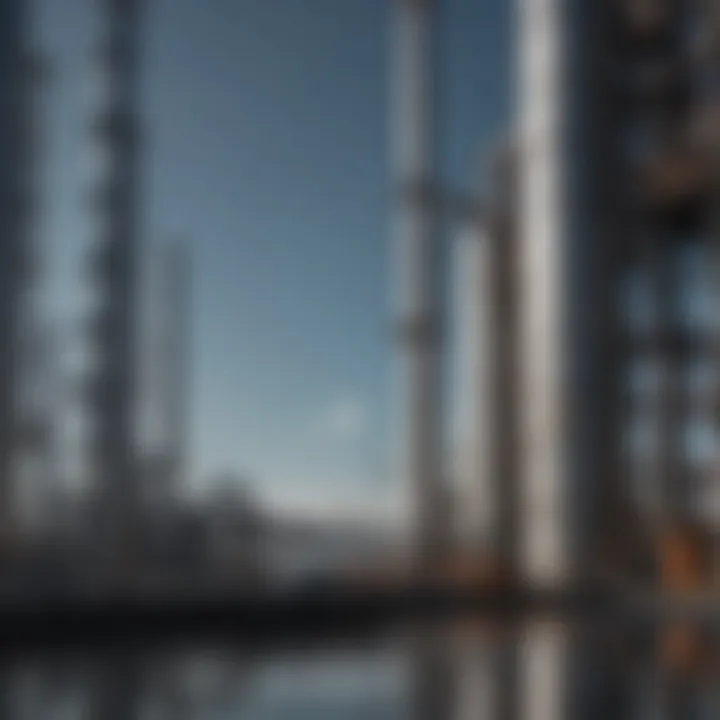
Moreover, the effectiveness of these chemical processes is influenced by various factors, such as temperature, pressure, and the concentrations of reactants. Optimizing these conditions is critical in designing efficient carbon capture systems.
Physical Mechanisms
Physical mechanisms complement chemical processes by utilizing physical interactions to capture carbon dioxide. One primary method is adsorption, where carbon dioxide molecules adhere to the surface of solids, called adsorbents. Materials such as activated carbon, zeolites, and silica gel have been widely studied for their adsorption properties. They can bind carbon dioxide under high pressure, allowing it to be captured from flue gases or ambient air.
Another noteworthy approach is membrane technology, which employs selective permeation to separate carbon dioxide from other gases. Membranes can be made from polymers or ceramics that allow carbon dioxide to pass through while blocking other gases, making them an appealing option for reducing energy consumption compared to traditional methods.
Furthermore, these physical mechanisms may be coupled with other technologies to enhance performance. For instance, integrating adsorption and chemical absorption can lead to innovative dual capture systems that effectively target carbon dioxide across various applications.
"The combination of chemical and physical mechanisms in carbon capture highlights the multi-faceted nature of the challenge. By leveraging the strengths of both approaches, we can develop more integrated and efficient systems."
Carbon Capture Technologies
Carbon capture technologies represent a vital area of study in the quest to reduce atmospheric CO2 levels. These technologies are not just theoretical; they embody practical approaches to combating climate change. By capturing carbon dioxide before it enters the atmosphere or removing it directly from the air, these methods can significantly mitigate the adverse effects of climate change.
Key benefits of carbon capture technologies include:
- Reduction of Greenhouse Gases: They play a central role in lowering the amount of CO2 emitted by power plants and industrial processes.
- Support for Renewable Energy: These technologies can complement renewable energy systems, enabling transitions to more sustainable energy practices.
- Potential for Carbon Utilization: Captured CO2 can be used in various applications, such as enhancing oil recovery or in the production of building materials.
Despite these advantages, there are considerations that need careful evaluation. The initial costs and complexity of installation can be barriers for widespread adoption. There is also a need for comprehensive infrastructure development to support these technologies.
Direct Air Capture
Direct air capture (DAC) systems actively remove CO2 from the atmosphere. This method employs chemical processes to extract carbon dioxide, which can then be stored or utilized. The significance of DAC lies in its potential for large-scale deployment, complementing efforts to reduce emissions at their sources.
The core components of DAC include:
- Air Contactors: These are designed to draw air into the system, where it comes into contact with a chemical solution that binds to CO2.
- Regeneration: Once the solution is saturated with CO2, it undergoes a regeneration process to release the captured gas and produce a concentrated stream.
The promise of DAC is tempered by its current cost structure and energy requirements. Technological advancements are crucial to enhance efficiency and lower expenses.
Carbon Capture from Industrial Sources
Capture techniques have evolved to target industrial emissions. Industries such as cement, steel, and chemical manufacturing are significant contributors to global CO2 emissions. Targeting emissions at their source is a pragmatic approach to carbon reduction.
Processes utilized in this area include:
- Post-Combustion Capture: This method captures CO2 from flue gases after combustion.
- Oxy-Fuel Combustion: In this approach, fuels are burned in an environment with enriched oxygen to create a concentrated stream of CO2.
While capturing CO2 directly from industrial processes is effective, it also presents distinct challenges. There must be ongoing research and development to improve integration within existing infrastructure and to ensure economic viability.
Biological Approaches
Biological methods of carbon capture are less conventional but have gained interest. These techniques use biological systems, such as plants and algae, to sequester carbon naturally. They aid in the reduction of atmospheric CO2 through photosynthesis.
Significant aspects of biological approaches include:
- Afforestation and Reforestation: Planting trees absorbs CO2, acting as natural carbon sinks.
- Soil Management: Proper management practices can increase carbon sequestration in soils.
- Algal Biofuels: Algae can absorb CO2 while producing biomass, which can be converted to energy.
"Effective carbon capture technologies can serve as essential tools in the global strategy to combat climate change."
Understanding the diverse technologies available provides insights into practical steps society can take towards a sustainable future.
Assessment of Carbon Capture Efficacy
The efficacy of carbon capture technologies is central to understanding their potential contribution to mitigating climate change. Effective assessment informs researchers, policymakers, and the public about the real impact these technologies can achieve. This assessment also entails evaluating both short-term effects as well as long-term consequences on the environment and society. Notably, measuring effectiveness and engaging in comparative studies play foundational roles in this comprehensive evaluation.
Measuring Effectiveness
Measuring the effectiveness of carbon capture systems involves a multifaceted approach. Key metrics often focus on the quantity of carbon dioxide captured, energy consumption in the capture process, and the stability of the captured carbon post-process. There are various methodologies to conduct such measurements, including the following:
- Quantitative Analysis: This approach utilizes precise data collection methods to determine how much carbon dioxide is being captured over time. It provides a numerical basis for evaluating success.
- Energy Efficiency: This metric looks at how much energy is expended in capturing a unit of carbon dioxide. A system that captures more CO2 while using less energy is more effective and sustainable.
- Lifecycle Assessment: This is a broader analysis that examines the overall environmental impact of carbon capture technology, from material extraction through operation to eventual disposal.
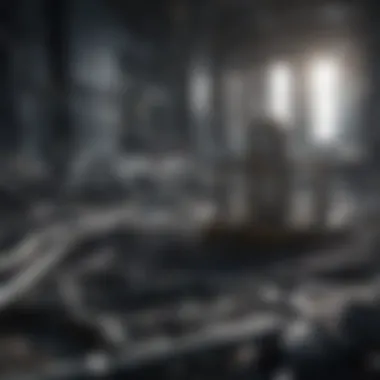
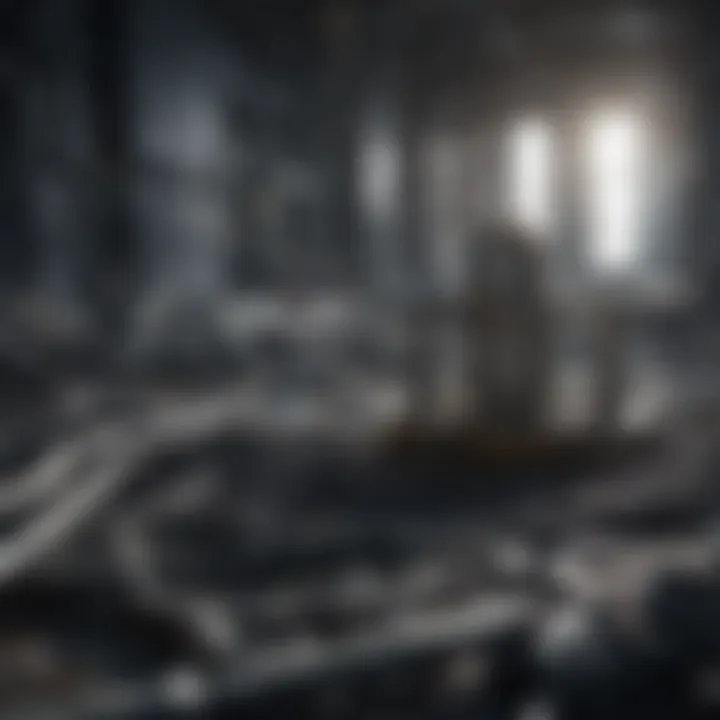
These approaches allow for a comprehensive understanding of how various systems operate in real-world conditions, creating a clearer picture of their effectiveness. Moreover, it provides insight into how improvements can be made in existing technologies.
Comparative Studies
Comparative studies are essential for assessing the relative efficacy of different carbon capture technologies. These studies aim to analyze and compare the performance of varying methodologies, enabling researchers and policymakers to identify the most promising options available. Some aspects considered in these studies include:
- Cost-Effectiveness: Comparing the economic viability of various carbon capture technologies involves analyzing installation, operational, and maintenance costs against their carbon capture capacity.
- Scalability: This aspect examines how easily a technology can be scaled up to meet the demands of larger facilities or industrial applications. Technologies that can be modified for different scales are often favored.
- Environmental Impact: Comparing how each technology affects the environment gives insights into potential drawbacks, including resource consumption and ecological disturbance.
"Comparative studies provide a crucial perspective on developing more efficient and effective carbon management strategies."
Research in this area can help direct funding and resources towards the most promising technologies, ensuring a strategic approach to tackling carbon emissions and climate change. Furthermore, it facilitates collaboration among researchers and industries, leading to more informed decisions.
The assessment of carbon capture efficacy thus stands as a critical endeavor. By systematically measuring effectiveness and engaging in comparative studies, we can navigate the complexities of carbon capture technologies and strengthen our efforts towards achieving climate goals.
Challenges in Carbon Capture Implementation
The journey towards effective carbon capture technology is fraught with numerous challenges that can hinder progress. These challenges span across technological, economic, and social dimensions. Addressing these obstacles is crucial to ensure that carbon capture can be implemented on a large scale. Each of these areas presents unique considerations and impacts the overall efficacy of carbon capture systems.
Technological Barriers
Technological barriers represent a significant hurdle in the advancement of carbon capture. Many existing methods for capturing carbon dioxide require advanced materials and processes that are not yet fully developed for commercial use. For example, the efficiency of sorbents and solvents varies greatly. These materials must optimize the absorption of CO2 while minimizing energy use and operational costs.
Moreover, the design of capture devices, like those used in direct air capture, often faces limitations in scalability. The conversion of captured carbon into useful products also remains a nascent field. Research shows that while some methods, such as mineralization, are promising, they often require additional investment in infrastructure and technology development.
In addition, reliability and durability of capture systems are paramount. Frequent maintenance and the need for upgrades can significantly impact long-term investment attractiveness. Addressing these technical challenges is essential to create efficient and reliable carbon capture technology that can be integrated into current industrial systems without excessive costs.
Economic Considerations
Economic considerations play a critical role in the successful implementation of carbon capture technologies. High capital and operational expenses are significant barriers for many organizations. According to recent studies, costs related to capturing carbon range widely, influenced by factors such as technology type and location.
The lack of strong economic incentives further complicates matters. Many industries are not compelled financially to invest in carbon capture; thus, without supportive policies or substantial carbon pricing, there is little motivation to adopt these technologies. Government support and subsidies can alter the financial landscape, making investments in carbon capture more appealing.
Furthermore, the return on investment is uncertain. This uncertainty can deter potential investors, especially in a volatile economic environment. To encourage wider adoption, it is crucial to develop robust financial models that can predict the long-term benefits of carbon capture, alongside government policies aimed at enhancing economic feasibility.
Public Perception
Public perception also heavily influences carbon capture implementation. There is often resistance from communities regarding new technologies, particularly those associated with industrial projects. Misconceptions about the safety and efficacy of carbon capture can lead to skepticism and opposition.
Engaging with the public is essential for fostering acceptance. Transparency about the benefits and risks associated with carbon capture can help build trust. Initiatives that demonstrate success stories can encourage a more favorable view. Moreover, involving local communities in decision-making processes can make them feel part of the solution.
"Public support is an essential ingredient for any large-scale implementation of technology. Without it, efforts can stall, regardless of the scientific merits."
The challenges of technological barriers, economic considerations, and public perception are significant. Yet, they are not insurmountable. By addressing these obstacles with targeted strategies, the potential of carbon capture can be unlocked, leading to substantial environmental benefits.
Environmental Implications of Carbon Capture
The environmental implications of carbon capture are a crucial thematic element in understanding the wider impacts of this technology. As global efforts to combat climate change intensify, it is increasingly important to assess not just the efficacy of carbon capture systems, but also their ecological consequences. This section will examine how carbon capture can affect ecosystems, the potential for carbon sequestration, and the broader environmental considerations that accompany the implementation of these technologies.
Impact on Ecosystems
The deployment of carbon capture technologies can have mixed effects on ecosystems. On one hand, by reducing the amount of carbon dioxide in the atmosphere, these innovations may contribute to lessening the greenhouse effect. This can lead to moderated climate change impacts, including reduced extreme weather events and altered species habitats.
However, the implementation of large-scale carbon capture projects can disrupt local ecosystems. For instance, the construction of capture facilities might lead to habitat loss for various species. Furthermore, if the carbon is stored underground, there are risks associated with leakage, which could affect groundwater and soil health. Thus, protecting ecosystem integrity while developing carbon capture systems is paramount.
Potential benefits to ecosystems include:
- Enhanced Biodiversity: By mitigating climate change, carbon capture may promote conditions for diverse species to thrive.
- Soil Restoration: Some carbon capture technologies can be integrated with soil management practices that enhance soil health.
Conversely, challenges include:
- Habitat Disruption: Land used for carbon capture infrastructure may displace native flora and fauna.
- Chemical Contamination: Risk exists if captured carbon interacts with geological formations, potentially leading to contamination of surrounding environments.
"Understanding the dual role of carbon capture is fundamental. While its goals are aimed at climate mitigation, the paths to achieve these goals may unwittingly threaten ecological equilibria."
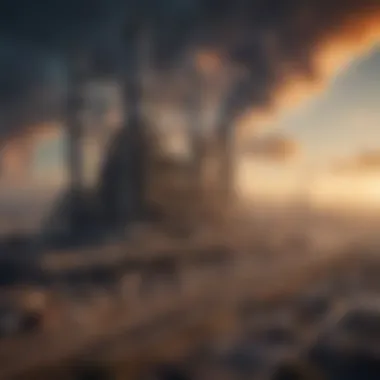
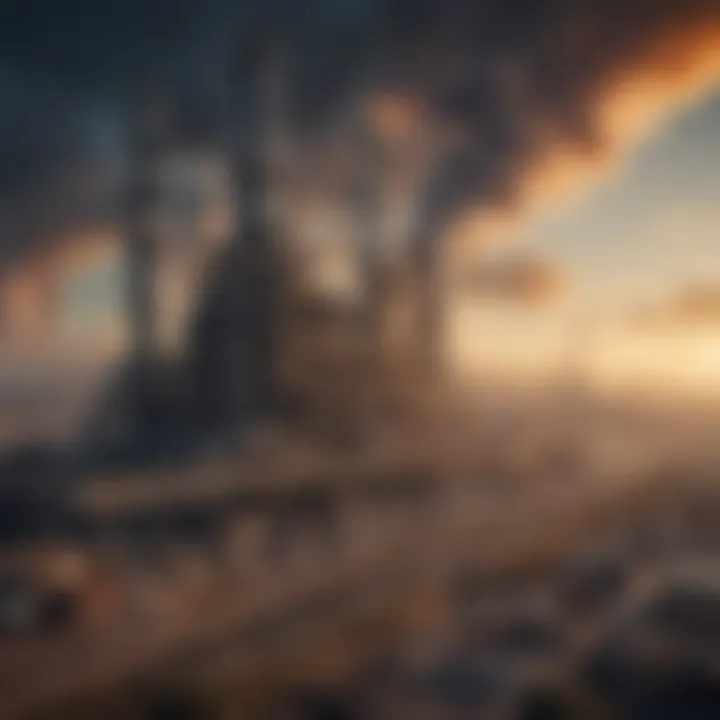
Carbon Sequestration Potential
Carbon sequestration refers to the long-term storage of carbon dioxide or other forms of carbon to mitigate or defer climate change. The potential for this process hinges on several factors, including the technology employed, the geological characteristics of storage sites, and the regulatory environment.
Key aspects of carbon sequestration potential include:
- Geological Sequestration: This method injects carbon dioxide into deep geological formations. Studies indicate that certain formations may store significant quantities of CO2 securely.
- Ocean Sequestration: Research explores the oceanβs capability to absorb excess atmospheric carbon. However, implications for marine ecosystems must be carefully assessed.
- Biological Sequestration: Enhancing carbon storage through soil and biomass can support agricultural practices, but long-term monitoring is necessary to ensure effectiveness.
The broader implications of carbon sequestration are profound. Achieving efficient carbon capture and effective storage could transform the environment by reducing atmospheric carbon levels, fostering climate resilience, and contributing to environmental restoration. Yet, the success of these efforts relies heavily on a scientific understanding of ecosystems and geological formations.
In summary, the environmental implications of carbon capture are complex. They embody a balance of potential benefits and risks. As we refine and deploy these technologies, the importance of holistic assessments cannot be overstated.
Carbon Capture and Climate Policy
Understanding the relationship between carbon capture and climate policy is crucial in addressing the growing concern of climate change. Carbon capture technology plays a significant role in helping nations meet their emissions reduction goals laid out in international agreements such as the Paris Accord. This section discusses the multifaceted benefits of carbon capture, the intricate considerations involved, and its essential place within climate policy frameworks.
Role in Mitigating Climate Change
The primary aim of carbon capture technologies is to reduce the concentration of carbon dioxide in the atmosphere. This is particularly important given the alarming rise in global temperatures linked to greenhouse gas emissions.
- Emission Reduction: Carbon capture processes can mitigate emissions from both fossil fuel combustion and industrial sources. By capturing CO2 before it reaches the atmosphere, this technology directly contributes to reducing the greenhouse gas burden.
- Sustainable Energy Transition: As the energy sector shifts towards renewable sources, carbon capture can manage the emissions from periods where fossil fuels may still be needed. This creates a transitional phase where carbon capture becomes a viable solution until fully renewable systems can achieve energy demands without emissions.
- Enhanced Carbon Sequestration: Captured carbon can be used in enhanced oil recovery or permanently stored underground, preventing it from re-entering the atmosphere. This creates dual benefits by both using resources and sequestering harmful carbon emissions.
The integration of carbon capture is not without challenges. However, its role as a solution is gaining recognition as part of comprehensive climate strategies that include emissions reductions, renewable energy expansion, and sustainable practices.
Policy Frameworks
To optimize the implementation of carbon capture technologies, robust policy frameworks are essential. Governments need to establish clear regulations, funding mechanisms, and incentives that support technological advancements and address public concerns. Key elements of effective policy frameworks include:
- Regulatory Support: Policies must clarify operational guidelines for carbon capture projects, ensuring compliance with environmental standards while promoting innovation.
- Financial Incentives: Providing subsidies, tax credits, and grants can lower the economic burden associated with developing and installing carbon capture technology. For instance, the 45Q tax credit in the United States incentivizes the capture and sequestration of CO2, illustrating a successful model of economic policy aiding technology deployment.
- Research and Development: Investment in R&D is critical for enhancing existing technologies and creating new methods. Collaborative efforts between governments, universities, and private sectors can accelerate breakthroughs that reduce costs and improve efficiency of carbon capture processes.
"Policy frameworks can significantly influence the adoption and effectiveness of carbon capture technologies, paving the way for a sustainable future."
Future Directions in Carbon Capture Research
The field of carbon capture science is evolving rapidly. It is crucial for the ongoing fight against climate change. Future directions in research may lead to innovative solutions that can enhance existing methods of capturing carbon dioxide. Understanding these directions is important for students, researchers, and policymakers alike.
Innovative Technologies
New technologies are at the forefront of advancing carbon capture methods. Innovations such as advanced solvents, solid sorbents, and membrane technologies are gaining traction. These technologies aim to increase efficiency and reduce costs associated with carbon capture processes.
One promising area is the development of biodegradable sorbents. These materials can potentially improve land use while capturing more carbon. Moreover, integrating artificial intelligence can help optimize the carbon capture process by predicting conditions that maximize efficiency.
Additionally, the exploration of carbon utilization pathways is essential. For example, converting captured carbon into valuable products such as fuels, chemicals, or construction materials can create economic incentives for capture technologies.
Collaborative Research Trends
Successful carbon capture research hinges on collaboration across disciplines and sectors. Universities, governmental agencies, and private companies are forming partnerships to pool resources and expertise. This collaborative approach can accelerate knowledge transfer and innovation.
Furthermore, international collaboration is equally vital. Climate change is a global issue that requires shared strategies and solutions. Research networks and consortia like the Carbon Capture Coalition are fostering cooperation among diverse stakeholders. These networks facilitate data sharing and joint studies that strengthen the overall body of knowledge in carbon capture.
"Collaboration is key to advancing carbon capture technologies and addressing climate challenges effectively."
By investing in these future directions, we can shape an effective response to climate change. Understanding the importance of innovative technologies and collaborative research trends allows all stakeholders to contribute meaningfully to a sustainable future.
The End
The conclusion serves as a critical element in understanding the implications and overall significance of carbon capture science. Throughout this article, we have explored various dimensions of carbon capture, from the fundamental principles to the challenges and future prospects. This reflection not only consolidates the key points discussed but also highlights the necessity of continued research and collaboration in this field.
A summary of the relevant findings allows researchers and policymakers to pinpoint effective strategies. The exploration of technologies such as Direct Air Capture, industrial carbon capture, and innovative biological methods showcases diverse approaches to CO2 removal. Each method comes with its advantages and challenges, making it essential to evaluate them thoroughly, as their effectiveness varies based on specific contexts.
CBDMoreover, understanding public perception and addressing the economic considerations can significantly affect the adoption of these technologies. The challenges are not merely technical but encompass societal acceptance and investment viability. Thus, grasping the intersection between technology and policy is vital for making informed decisions.
In essence, the conclusion also serves as a call for action. For agents in academia and industry, the results point toward a need for innovative thought leadership and policy engagement. The journey of carbon capture science is pivotal in combating climate change. Thus, a coherent strategy involving multiple stakeholders is imperative for its success.
Summary of Key Points
- This article has navigated through the complex nature of carbon capture science, explaining its mechanisms and the challenges faced.
- Key technologies explored include Direct Air Capture and various biological approaches, underlining their effectiveness.
- The connection between technological advancement and economic implications was emphasized, indicating a need for robust policy frameworks.
Call to Action for Researchers and Policymakers
- Embrace collaborative research initiatives that focus on innovative carbon capture technologies to enhance effectiveness.
- Advocate for public engagement, ensuring that societal views influence policy frameworks positively.
- Prioritize funding towards scalable solutions in carbon capture, enabling timely responses to climate change challenges.