In-Depth Insights into Displacement Probes
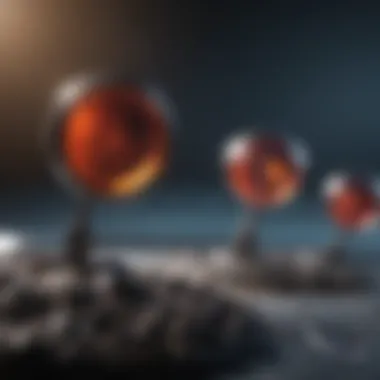
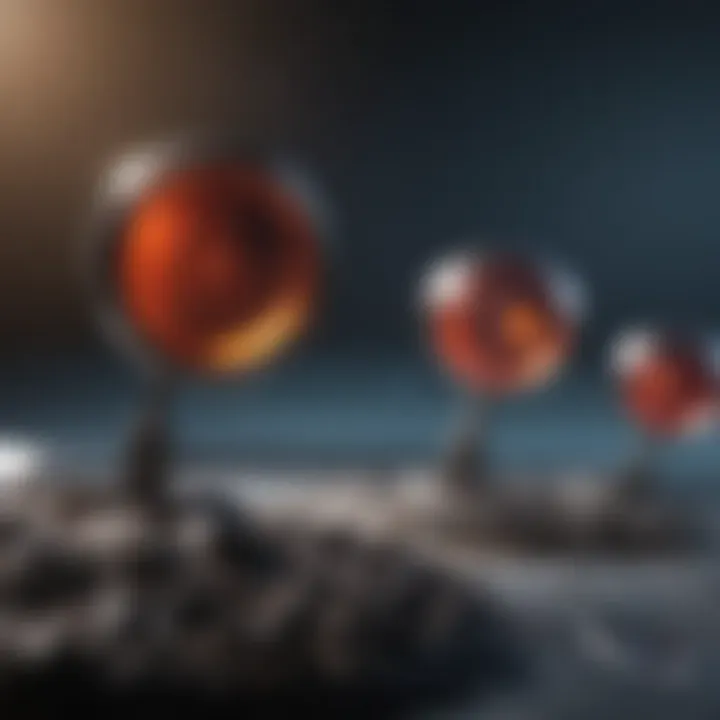
Intro
Displacement probes are crucial devices used in a variety of scientific and industrial applications. They measure the exact position or displacement of an object. Their precision is essential in many fields including engineering, robotics, and manufacturing processes. Understanding how these probes work and their various applications can greatly improve operational efficiency.
In this article, we will dissect the principles behind displacement probes, explore different types, and review case studies highlighting their utility in real-world scenarios. We will also look at the challenges these devices face as technology evolves.
Research Overview
Summary of Key Findings
Displacement probes are fundamental tools in precise measurement tasks. They can be divided into several categories based on their technology, including capacitive, inductive, and optical probes. Each type has unique advantages and limitations. For example:
- Capacitive probes excel in measuring non-contact displacement with high sensitivity.
- Inductive probes are advantageous for environments with high temperatures.
- Optical probes provide quick measurements but can be susceptible to environmental conditions.
Understanding these variations is vital when selecting the appropriate probe for specific tasks.
Research Objectives and Hypotheses
The primary objective of this article is to explore the operational mechanisms and applicability of displacement probes. It aims to elucidate how advancements in technology have contributed to precision measurement. We hypothesize that increased integration of smart technology in displacement probes will enhance their accuracy and broaden their applications across disciplines.
Methodology
Study Design and Approach
This analysis adopts a systematic review of current literature surrounding displacement probes. By gathering data from varied academic resources and technical manuals, we present a comprehensive narrative of the subject matter. Each type of probe is studied based on specifications, functional principles, and practical applications.
Data Collection Techniques
Data was collected through:
- Comprehensive review of peer-reviewed journals
- Technical specifications from manufacturer websites
- In-depth analysis of case studies to understand real-world applications
This data forms the backbone of our exploration, providing a critical context that informs our understanding of displacement probes in modern technology.
Displacement probes stand at the intersection of innovation and measurement science, driving accuracy and efficiency in numerous applications.
Preface to Displacement Probes
Displacement probes are pivotal instruments in various scientific fields, providing precise measurements critical for both research and industrial applications. Their ability to gauge minute changes in position makes them indispensable in engineering systems, manufacturing processes, and advanced research environments. In this section, we will delve into the definition of displacement probes and explore their historical evolution, allowing readers to comprehend why they are essential tools in metrology and engineering.
Definition of Displacement Probes
Displacement probes are sensors designed for measuring the displacement or movement of an object relative to a reference point. They capture both linear and angular displacements, allowing for detailed analysis of physical changes and movements within a system. Typically, these probes convert mechanical motion into an electrical signal that can be processed and analyzed. There are several types of displacement probes, including capacitive, inductive, optical, and mechanical probes, each offering unique advantages and specific applications.
Key Characteristics of Displacement Probes:
- Accuracy: High precision in measurement is crucial.
- Sensitivity: Ability to detect slight changes is essential.
- Durability: Probes must withstand various environmental conditions.
- Response Time: Quick measurement is vital in dynamic applications.
Historical Background
The development of displacement probes stems from the need for accurate measurement tools in engineering and physical sciences. The origins can be traced back over a century, with early versions relying on mechanical devices. Initially, devices such as dial indicators and micrometers were used, which provided limited precision and were subject to human error.
As technology advanced, the introduction of electronic sensors revolutionized displacement measurement. The invention of capacitive and inductive sensors in the mid-20th century marked a significant turning point, allowing for more precise and reliable measurements. Nowadays, displacement probes are integral to modern automation, robotics, and quality control processes, showcasing their evolution from simple mechanical devices to advanced digital measurement tools.
Operational Principles
Understanding the operational principles of displacement probes is crucial. This section sheds light on the foundation of how these devices function and their significance in accurate measurement. Displacement probes are essential in environments where precise positioning and measurement are necessary. Their operational mechanisms contribute significantly to industrial applications, research efforts, and medical technologies. Knowledge of these principles assists users in selecting the appropriate displacement probe for their specific needs.
Basic Working Mechanisms
Displacement probes operate on various principles, each tailored to measure displacement in a unique way. The basic working mechanisms include sensors that detect changes in position or movement. These sensors convert mechanical motion into a measurable signal. Depending on the type of probe, the signal output may vary, making it vital to understand the distinct methods used across different probe designs. The fundamental understanding of these mechanisms is what makes it possible for engineers and scientists to apply the correct technology in their work.
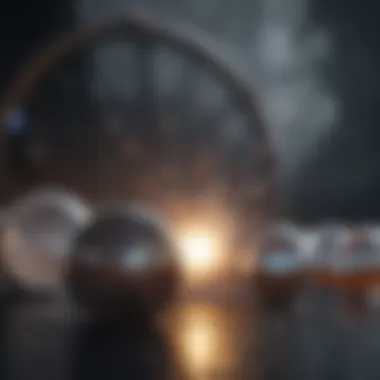
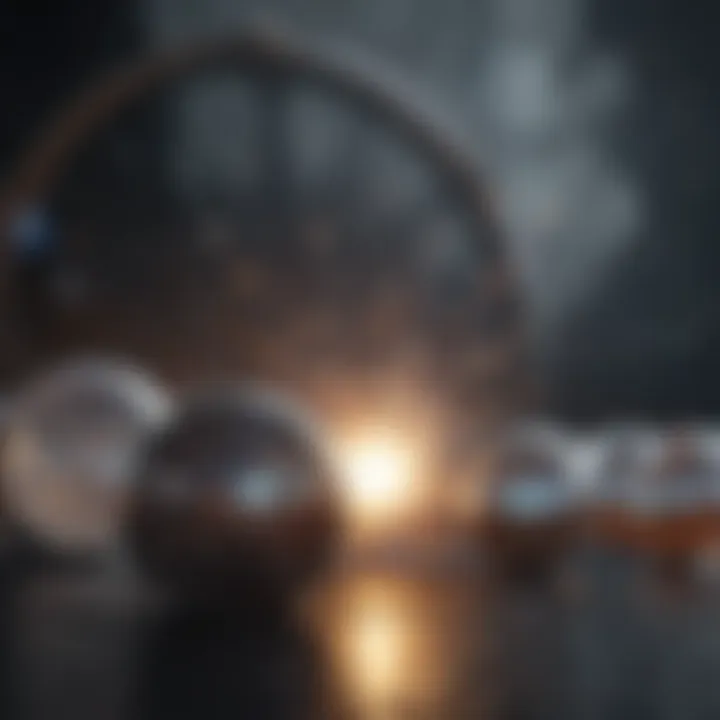
Types of Displacement Probes
Displacement probes come in several types, each with distinct characteristics and applications, which impact their functional effectiveness. The major types include capacitive, inductive, optical, and mechanical probes. Each type has a specific aspect that enhances its use in various applications.
Capacitive Probes
Capacitive probes work by measuring changes in capacitance between sensor plates. This type is known for its ability to detect small displacements with high accuracy. Capacitive probes are often favored for tasks requiring fine measurements in applications like semiconductor manufacturing. Their key characteristic is their sensitivity, which allows for precise detection even with minimal displacement.
However, they can be sensitive to environmental changes, such as temperature fluctuations, which may impact accuracy. Yet, this sensitivity can also be an advantage, as it can detect subtle movements that others might miss, making capacitive probes valuable in environments requiring high precision.
Inductive Probes
Inductive probes function based on electromagnetic principles. They measure displacement by detecting changes in inductance as a target moves. The advantage of inductive probes lies in their robustness and ability to work in harsher environments compared to capacitive probes. They are commonly used in machinery where conditions such as dust, oil, or moisture may be present.
One key characteristic of inductive probes is their non-contact measurement capability, which limits wear and tear on the sensor. However, their sensitivity to metal objects nearby can introduce measurement errors, so careful placement is necessary for accurate readings.
Optical Probes
Optical probes utilize light to measure displacement. By analyzing light reflection or interference patterns, they can ascertain movement with remarkable precision. These probes are particularly beneficial in research settings, where high-resolution measurements are essential. The key strength of optical probes is their ability to measure displacement over substantial distances and with very fine resolutions.
Despite their advantages, optical probes can be affected by external light sources and dust, which may introduce errors. Therefore, controlling the environment around the probe is crucial for achieving accurate results.
Mechanical Probes
Mechanical probes operate through direct contact with the object being measured. They often utilize spring-loaded mechanisms to gauge displacement. Their straightforward design makes them popular in various industries due to their reliability and ease of use. Mechanical probes are particularly useful in situations where high durability is needed, such as in rough manufacturing environments.
The primary limitation of mechanical probes is their wear over time due to contact with surfaces. This characteristic can lead to reduced accuracy as they age. Thus, it is necessary to inspect mechanical probes regularly to maintain their performance and reliability.
"The type of displacement probe selected for a specific application can significantly impact the measurement outcomes and overall project success."
Understanding these operational principles and types is essential for selecting the right displacement probe. Each type offers unique features, advantages, and disadvantages, making it crucial to evaluate application needs thoroughly.
Applications of Displacement Probes
Displacement probes find diverse applications across various sectors. They serve as crucial tools in enhancing precision and facilitating accurate measurement in different settings. Their significance is in their ability to effectively translate small distances into measurable electrical signals, making them essential in many fields. This section explores both industrial and research applications, along with their benefits and considerations.
Industrial Applications
Manufacturing and Assembly
In manufacturing and assembly, displacement probes are essential for ensuring precision during production processes. These probes help in monitoring critical dimensions and alignment, which are vital for maintaining product quality. A key characteristic of this application is the high level of accuracy required. Displacement probes enable manufacturers to achieve this consistency by providing real-time feedback on position and motion. Such precision is a beneficial trait for industries focused on efficiency and quality.
A unique feature of using displacement probes in manufacturing is their capability to automate measurement tasks. This automation significantly reduces human error and labor costs, boosting overall efficiency. However, the initial investment may be a disadvantage for smaller operations. Nevertheless, the return on investment often justifies the technology's use in the long run.
Quality Control
Quality control is another critical area where displacement probes are utilized. These probes assist in detecting deviations from specified tolerances in products. The ability to accurately measure and assess quality leads to enhanced product performance and customer satisfaction. One notable aspect is the probes' capacity to integrate with various quality management systems. This integration streamlines data collection and analysis.
The unique feature of displacement probes in quality control is their non-contact measurement capabilities. Such features prevent damage to delicate parts during inspections. However, the challenge lies in the need for constant calibration to maintain accuracy. Overall, the advantages of improved quality assurance make the use of displacement probes in this area essential.
Research and Development
Experimental Physics
In experimental physics, displacement probes play a crucial role in acquiring accurate data in experiments. Their precision is essential for validating theoretical models and hypotheses. This application is particularly beneficial because it allows researchers to observe minute changes in parameters that may influence outcomes.
A distinct aspect of using displacement probes in this realm is their ability to operate in harsh environments, such as high-vacuum conditions or extreme temperatures. These capabilities allow scientists to push the boundaries of research. However, the complexity of setup and calibration can be challenging, requiring skilled personnel to operate effectively.
Material Science
Material science benefits significantly from displacement probes, especially when studying the properties of different materials. These probes enable researchers to measure mechanical properties, such as strain and deformation, under various conditions. The key characteristic is the ability to provide detailed insights into material performance, crucial for innovation.
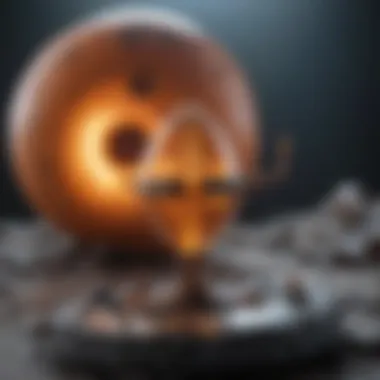
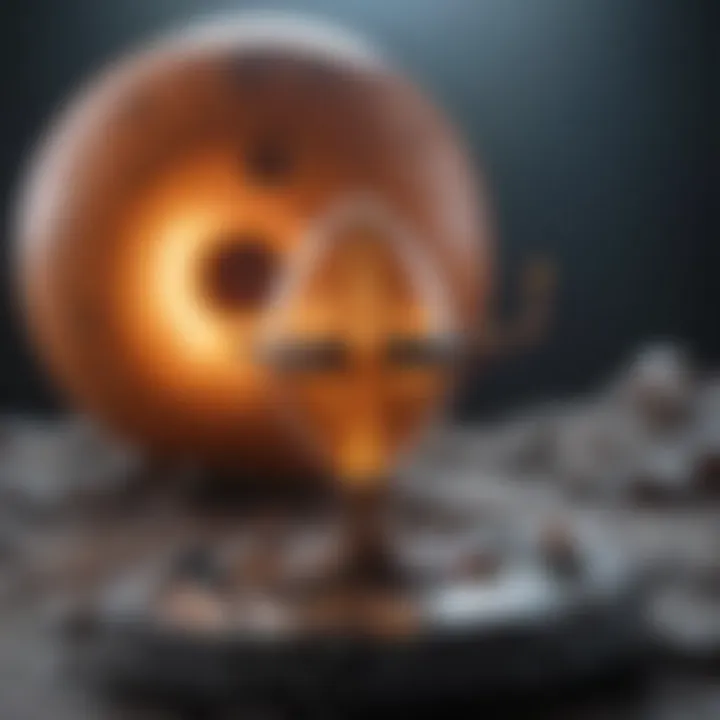
The unique feature of displacement probes in material science is their high sensitivity, allowing for the detection of even slight changes in material behavior. However, the downside can include the cost and complexity of more sophisticated probes. Despite these challenges, the data acquired significantly contribute to advancements in material development.
Medical Applications
Diagnostic Equipment
In the field of medical applications, displacement probes have transformed diagnostic equipment. They are utilized in devices that require precise measurements, such as ultrasound and MRI scanners. A principal reason for their use is their ability to provide real-time data, essential for accurate diagnosis and monitoring.
One unique feature of displacement probes in this context is their non-invasive measurement capabilities. This property enhances patient comfort and safety while ensuring accurate readings. Nonetheless, training and expertise are needed to handle these devices effectively.
Tissue Analysis
Tissue analysis is another significant area where displacement probes are applied. They help in measuring the physical properties of biological tissues, which can be critical in research and clinical settings. A vital characteristic is their role in enabling real-time observation of tissue response under various treatments.
The unique advantage of using displacement probes in tissue analysis lies in their high resolution. This resolution allows for detailed analysis that can influence treatment decisions. However, complex samples can pose challenges in data interpretation. Despite this, the benefits of precise tissue characterization justify the use of these probes in medical settings.
Technological Advancements
Technological advancements are pivotal in the evolution of displacement probes. They enhance measurement accuracy, improve efficiencies, and enable new applications that were not feasible in the past. Understanding these advancements helps contextualize their impact on various fields, including industrial manufacturing, research, and healthcare. As technologies continue to progress, it shapes both the design of the probes and their integration into broader systems.
Recent Innovations in Displacement Probes
Recent innovations in displacement probes focus on increasing precision and reliability. Developments in materials science have led to probes that can withstand harsher environments while maintaining their performance. For example, new coatings protect capacitive probes from wear and contamination. Additionally, the advent of micro-electromechanical systems (MEMS) has paved the way for smaller and more sensitive inductive probes. These MEMS-based designs enable a higher degree of integration with electronic systems, leading to enhanced real-time data collection.
Another noteworthy innovation is the implementation of wireless technology. This allows displacement probes to transmit data without the need for cumbersome wiring. Wireless capabilities not only simplify installation but also facilitate data gathering in remote or difficult-to-access locations.
Integration with Digital Technology
The integration of displacement probes with digital technology represents a significant advancement in measurement science. Modern probes now often feature digital interfacing capabilities. These have made it easier to connect probes to microcontrollers and other processing devices. Consequently, the data collected can be analyzed and visualized in real-time using various software tools, enhancing user experience.
Moreover, advancements in cloud technology allow data from various probes to be stored and processed in centralized systems. This approach supports large-scale data analysis and helps in detecting patterns that may not be obvious in isolated readings. By aligning displacement probes with digital technology, practitioners can achieve greater insight into the systems they are measuring.
"Digital integration transforms the traditional role of displacement probes, shifting from mere measurement tools to integral components of data-driven decision-making processes."
Furthermore, artificial intelligence (AI) applications are beginning to play a role in analyzing data from displacement probes. AI algorithms can process vast amounts of data quickly, identifying anomalies or trends that may indicate an issue or an opportunity for improvement. This capability can lead to timely interventions and optimizations in various processes.
In summary, technological advancements in displacement probes, particularly through recent innovations and digital integrations, not only improve the functionality of these instruments but also expand their application scope significantly. Keeping abreast of these advancements is crucial for students and professionals aiming to leverage the full potential of displacement probes in their respective fields.
Comparative Analysis
The section on comparative analysis holds significant value in the study of displacement probes. Understanding the nuanced differences among various types of displacement probes is crucial for making informed decisions in both scientific research and practical applications. This analysis not only highlights the strengths of each probe type but also allows for the identification of appropriate applications based on specific measurement needs. The ability to compare these devices fosters a critical perspective on their integration into different systems, influencing effectiveness and efficiency.
In this section, we will delve into two main subsections: the advantages of each type of probe and the limitations and challenges inherent in their usage. Each aspect provides deeper insight into why particular probes may be favored in specific situations, while also acknowledging potential drawbacks.
Advantages of Each Type of Probe
Displacement probes come in several types, each possessing unique advantages that cater to a variety of applications:
- Capacitive Probes: These probes excel in high precision settings. Their sensitivity to small changes in capacitance makes them suitable for applications in fields requiring micrometer-level measurements.
- Inductive Probes: Known for their robustness, inductive probes offer durability in harsh environments. They are effective in applications where physical contact is undesirable or could introduce error.
- Optical Probes: Their non-contact nature and high-speed measurement capabilities make optical probes ideal in experiments needing fast responses and minimal interference with the object being measured.
- Mechanical Probes: Often preferred because of their simplicity, mechanical probes are easy to use and can provide direct measurements in a hands-on approach. They tend to be more cost-effective for less complex applications.
Each type possesses distinct advantages that can significantly influence measurement outcomes, highlighting the importance of selecting the appropriate probe for the task.
Limitations and Challenges
Despite the advantages, each type of displacement probe also presents certain limitations and challenges:
- Capacitive Probes: They can be influenced by environmental factors such as humidity and dust, affecting accuracy. Additionally, they require careful calibration and setup to avoid errors.
- Inductive Probes: Their sensitivity to electromagnetic interference may limit their use in certain contexts, particularly near strong magnetic fields.
- Optical Probes: While they have high precision, they can be susceptible to optical noise and require clean environments, making them less practical in settings where dust or particulate matter is present.
- Mechanical Probes: They may introduce wear and tear on components over time, providing less longevity compared to other probe types. Also, their contact nature can affect the very materials they are measuring.
Understanding these limitations is essential for users to make educated choices about the best probe for their measurement tasks, balancing precision with practicality.
A comprehensive evaluation of the advantages and limitations of displacement probes informs their optimal usage, ensuring the selection of appropriate measurement tools for various applications.
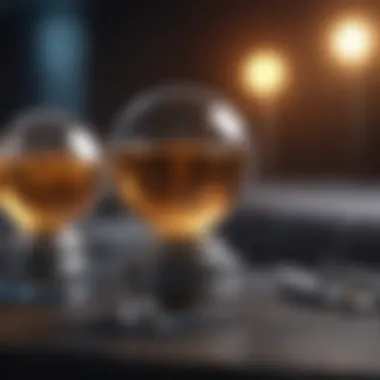
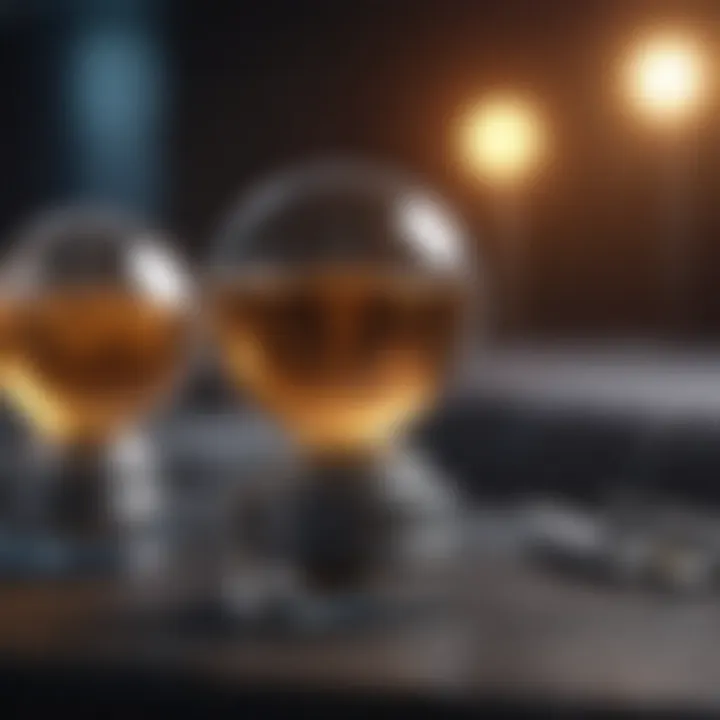
Case Studies
Case studies serve as vital narratives that illuminate real-world applications and outcomes of displacement probes. They highlight how these probes function across various settings, showcasing both successful applications and obstacles encountered during implementation. Through specific examples, readers gain insights into the versatility and challenges of displacement probes, adding depth to theoretical knowledge.
Successful Implementations
In numerous sectors, displacement probes have proven their worth through successful implementations. For instance, in industrial manufacturing, companies like Siemens have integrated capacitive displacement probes for measuring the warping of precision machined components. The data collected aids in adjusting the machining process, ensuring quality control and reducing material waste.
In the realm of medical technology, displacement probes are used in diagnostic equipment to assess tissue elasticity. For example, the use of optical probes in elastography has become common in hospitals. These devices enable clinicians to quantify tissue stiffness, providing critical information for early diagnosis of diseases like liver fibrosis.
Another notable example includes the use of inductive probes in automotive testing. Major automotive manufacturers employ these probes for precise measurements of mechanical components during assembly. This practice not only enhances operational efficiency but also aligns with industry standards for safety and performance.
- Applications in Manufacturing: Siemens uses capacitive probes for quality control.
- Medical Innovations: Optical probes in elastography help in tissue assessment.
- Automotive Testing: Inductive probes standardize measurements in assembly lines.
Failures and Lessons Learned
Failures in the use of displacement probes often yield important lessons that contribute to better practices and technologies. A noted failure occurred during an experiment using optical displacement probes in a research facility. The probes experienced calibration drift, leading to inaccurate data that compromised the results of an important material science study. This incident underscored the need for rigorous calibration protocols and regular maintenance of equipment.
Similarly, in a manufacturing context, a firm faced challenges integrating inductive probes into their process. The testing results were inconsistent due to interference from electromagnetic fields present in the environment. This highlighted the necessity of conducting thorough environmental assessments before installation, ensuring that the chosen technology fits the operational context.
Lessons from these failures can be classified as:
- Calibration Protocols: Regular checks are crucial to maintain accuracy.
- Environmental Assessments: Understanding surroundings can prevent complications.
- Adaptability: Technology must fit the unique challenges of specific industries.
"Failures shed light on the overlooked challenges in system integration; adapting to these insights leads to robust solutions."
In summary, case studies illustrate the importance of real-world applications of displacement probes. They provide valuable insights into both successful implementations and failures, offering lessons that can guide future endeavors in research and industry.
Future Directions
The exploration of future directions in displacement probes is vital for several reasons, particularly the evolution of measurement technology and its application in various fields. As the demand for precision increases in industry, research, and medical applications, developing more advanced displacement probes becomes critical. This section will discuss emerging trends and areas that require further research and innovation.
Emerging Trends in Displacement Probes
In recent years, displacement probes have witnessed several trends shaping their development. Some emerging trends include:
- Miniaturization: Modern applications demand smaller probes without sacrificing precision. This trend allows integration into compact systems, suitable for sensitive environments.
- Smart Probes: The incorporation of smart technology into displacement probes facilitates data collection and transmission. These probes can now integrate with IoT devices, enhancing real-time monitoring capabilities.
- Enhanced Sensitivity: Continuous improvement in sensor technology leads to greater sensitivity, enabling the measurement of smaller displacements. Capacitive and optical probes are at the forefront of this trend, facilitating their application in precision fields like material science.
These trends are propelling the displacement probe field forward, creating opportunities for enhanced measurement processes and innovative applications.
Research Gaps and Opportunities
Despite the advancements, there are significant research gaps in displacement probe technology worth addressing. Areas for further investigation include:
- Integration with Automation Systems: Probes must be optimized for seamless interaction with automated systems. Research can focus on developing protocols and standards to ease integration.
- Novel Materials: Exploring new materials for constructing displacement probes could lead to higher durability and reliability. Research in nanotechnology presents opportunities to create more efficient probes.
- Cross-Disciplinary Applications: Studies exploring how displacement probes can be adapted for use across different disciplines like environmental monitoring and biotechnology can open new avenues for their application.
Addressing these gaps not only will improve the functionality of displacement probes but also will enhance their acceptance and application across various sectors. The future of displacement probes, therefore, relies on sustained research and innovation.
Closure
The conclusion section of this article plays a pivotal role in reiterating the importance of displacement probes and summarizing the key insights presented throughout the text. Displacement probes are essential tools in measuring small movements with great precision. Their significance transcends various fields like manufacturing, research, and healthcare.
Summary of Key Points
In this article, we have covered several critical aspects of displacement probes. These include:
- Definition and Historical Background: Understanding what displacement probes are and their evolution over time.
- Operational Principles: Exploring how they work and the types available, such as capacitive, inductive, optical, and mechanical probes.
- Applications: Examining their roles in industry, research, and medical fields.
- Technological Advancements: Highlighting recent innovations and the integration of digital technology in their functionality.
- Comparative Analysis: Evaluating the advantages and limitations of each probe type.
- Case Studies: Learning from real-world implementations as well as failures.
- Future Directions: Discussing emerging trends and potential opportunities for further research.
This compilation of knowledge underscores the intricate designs and diverse functionalities of displacement probes, serving as fundamental components in precision measurement.
Final Thoughts on Displacement Probes
Displacement probes are more than just measurement devices; they are vital for achieving accuracy and efficiency in numerous applications. As technology evolves, these tools are likely to become even more critical, especially with advancements in digital integration and real-time data analysis. Understanding displacement probes allows professionals and researchers to optimize processes, improve product quality, and conduct innovative research.
The field still possesses significant gaps and opportunities for development. Thus, remaining abreast of the latest advancements will help stakeholders make informed decisions about their applications.
In closing, the exploration of displacement probes is not just about the tools themselves, but the broader implications they hold in enhancing scientific understanding and industrial practices.