CRISPR and iPSCs: Transforming Genetic Engineering
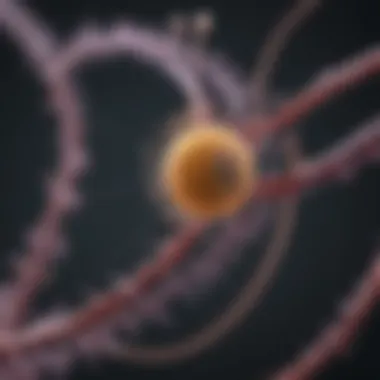
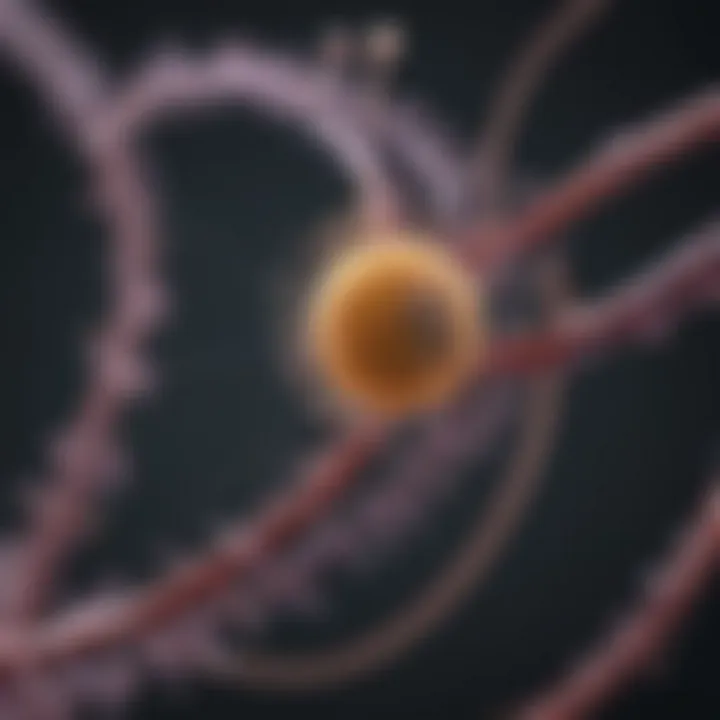
Intro
The fields of genetics and regenerative medicine are undergoing a transformative shift, propelled largely by advancements in technologies like CRISPR and induced pluripotent stem cells (iPSCs). CRISPR, a gene-editing tool derived from bacterial systems, allows for precise modifications in DNA sequences. On the other hand, iPSCs, which are generated from adult somatic cells, possess the ability to differentiate into virtually any cell type in the body. This combination holds immense potential for therapeutic applications, including the potential to treat various diseases, enhance regenerative capabilities, and provide new avenues for research and development.
In this article, we will delve into how these groundbreaking innovations intersect, offering fresh perspectives on genetic engineering and its implications in modern medicine. This narrative will explore critical aspects such as the mechanisms of CRISPR, the methodology of iPSC generation, and their potential applications. Additionally, we will critically assess ethical considerations and challenges that arise in this evolving field. The goal is to shed light on how these technologies are not only altering scientific landscapes but also carrying substantial responsibility for future generations.
Research Overview
Summary of Key Findings
Recent studies demonstrate that the use of CRISPR in conjunction with iPSCs can lead to enhanced specificity in gene editing, reducing off-target effects that have plagued earlier genetic modification approaches. Researchers have established that iPSCs facilitate a more efficient delivery of CRISPR components directly into target cells, resulting in improved editing accuracy.
Research Objectives and Hypotheses
The primary aim of recent investigations includes understanding how CRISPR can enhance the capabilities of iPSCs in tailored therapies. Furthermore, researchers are trying to validate the hypothesis that the combination of these technologies will lead to novel strategies for treating genetic disorders, potentially paving the way for personalized medicine.
Methodology
Study Design and Approach
Researchers typically employ a combination of laboratory experiments and computational models to study the interaction of CRISPR and iPSCs. By utilizing in vitro approaches, they can simulate cellular environments and analyze how these technologies work together.
Data Collection Techniques
Data collection includes genomic sequencing to evaluate the efficacy of CRISPR edits, stem cell differentiation assays to observe how well iPSCs develop into desired cell types, and patient-derived samples that provide context for therapeutic applications.
"Understanding the synergy between CRISPR and iPSCs is not merely a scientific pursuit; it is a necessary step towards the future of healthcare."
In summary, this exploration will provide both academic insights and practical implications of the evolving landscape driven by CRISPR and iPSCs. It aims to contribute to a broader understanding of genetic engineering in contemporary science.
Foreword to CRISPR Technology
The realm of genetic engineering has undergone an immense transformation, largely driven by the emergence of CRISPR technology. This section aims to elucidate the significance of CRISPR as a pivotal element in the landscape of genetic manipulation. CRISPR, which stands for Clustered Regularly Interspaced Short Palindromic Repeats, is a sophisticated tool allowing precise editing of genetic material. The introduction of this technology signifies not only a leap in laboratory techniques but also a paradigm shift in the approach to genetic disorders.
Overview of CRISPR
CRISPR technology was initially discovered as a part of the bacterial immune system. It serves as a defense mechanism against viruses. Essentially, the system is used by bacteria to recognize and cut the DNA of invading pathogens. Researchers have harnessed this natural process, adapting it for genetic engineering in various organisms. The ability to target specific sequences of DNA provides unparalleled specificity, accuracy, and efficiency compared to traditional methods of gene editing, such as zinc finger nucleases and TALENs.
Key Components of CRISPR-Cas Systems
At the heart of CRISPR technology lies the CRISPR-Cas system, which is composed of two integral components: the guide RNA (gRNA) and the Cas protein. The gRNA is designed to be complementary to the target DNA sequence, ensuring that the editing processes are precise. The most commonly used Cas protein is Cas9, which acts as molecular scissors, capable of cutting DNA at specific locations determined by the gRNA. This dual function of identification and cleavage allows for targeted modifications, paving the way for gene knockout, replacement, or insertion.
Applications of CRISPR in Genetic Engineering
The versatility of CRISPR applications spans across various fields in genetic engineering. Some notable applications include:
- Gene Therapy: Correcting mutations in genes responsible for diseases.
- Agricultural Innovations: Developing crops that are resistant to pests or drought conditions.
- Model Organisms: Creating genetically modified organisms for research purposes, facilitating studies on gene function and disease mechanisms.
- Synthetic Biology: Building novel pathways and circuits for biotechnology applications.
The implications of these applications are enormous, with the potential to revolutionize medicine, agriculture, and biotechnology. CRISPR technology not only enhances our ability to manipulate genetic information but also opens avenues for advanced treatments of genetic diseases and sustainable agricultural practices. This section will set the stage for understanding how CRISPR interfaces with induced pluripotent stem cells to create transformative prospects in the field of genetic engineering.
Understanding Induced Pluripotent Stem Cells
Induced pluripotent stem cells (iPSCs) represent a significant breakthrough in regenerative medicine and genetic engineering. Their ability to differentiate into various cell types offers tremendous potential for scientific research and therapeutic applications. This section will elucidate the definition, characteristics, methods of generation, and how iPSCs compare with embryonic stem cells.
Definition and Characteristics of iPSCs
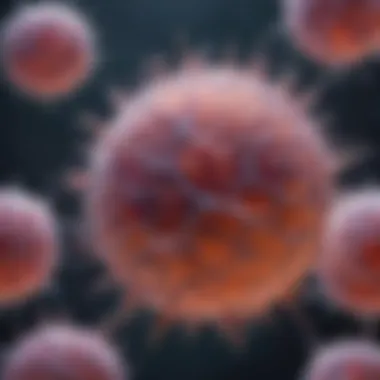
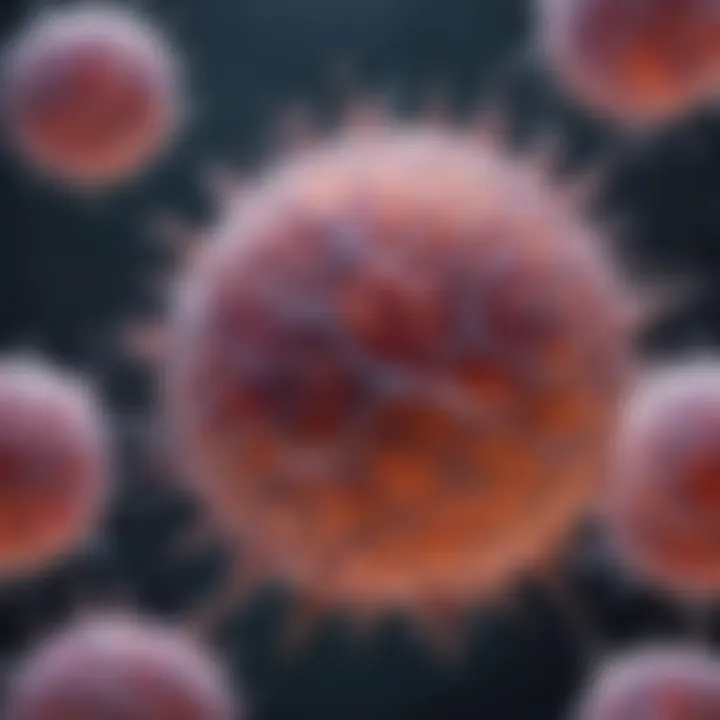
Induced pluripotent stem cells are somatic cells reprogrammed to a pluripotent state. This means they have the potential to develop into any type of cell in the body. The reprogramming process involves introducing specific transcription factors, such as Oct4, Sox2, Klf4, and c-Myc, which facilitate this transformation.
The main characteristics of iPSCs include their ability to self-renew indefinitely while maintaining pluripotency. This quality allows researchers to generate vast amounts of cells for experiments or therapies. Additionally, iPSCs can differentiate into various cell types, including neurons, cardiomyocytes, and pancreatic beta cells. These features make them a promising alternative to embryonic stem cells, which raise ethical concerns.
Methods of iPSC Generation
There are several methods for generating iPSCs. The most common approach is the use of viral vectors. This technique involves introducing the aforementioned transcription factors into the target somatic cells through viral delivery. While effective, the use of viral vectors can raise concerns about insertional mutagenesis and potential cancer risk.
Another method is using non-integrating vectors, which avoid incorporating the reprogramming factors into the host genome. These methods are generally considered safer, though they often have lower efficiency.
Additionally, researchers have developed techniques like cell fusion, where somatic cells are fused with embryonic stem cells. This can also result in iPSC formation. However, it is less commonly used due to complexity and ethical considerations.
Emerging techniques, such as CRISPR-based approaches, offer a more refined method for generating iPSCs. These methods aim to minimize risks associated with traditional techniques by precisely editing the genes involved in reprogramming.
Comparison with Embryonic Stem Cells
The distinction between iPSCs and embryonic stem cells is pivotal for understanding their roles in research and therapy. Embryonic stem cells are derived from blastocysts and possess a naturally high pluripotent capability. However, their use is often controversial due to the ethical implications surrounding the destruction of embryos.
In contrast, iPSCs are generated from adult somatic cells, sidestepping many ethical dilemmas associated with embryonic stem cells. Moreover, iPSCs can be derived from an individual's own cells, reducing the risk of immune rejection when used in therapies.
Despite these advantages, there exist some limitations with iPSCs. For instance, while they may present more manageable ethical considerations, their reprogramming can lead to genetic and epigenetic abnormalities that might affect their functionality. Additionally, some differences in differentiation potential and behavior compared to embryonic stem cells remain.
"Understanding the unique characteristics and generation methods of iPSCs paves the way for their application in regenerative medicine and therapies."
In summary, induced pluripotent stem cells not only enhance our understanding of cellular biology but also open new avenues in medical treatments. Their ability to replicate the characteristics of embryonic stem cells, while addressing ethical concerns, makes them a vital part of contemporary genetic engineering.
The Synergy of CRISPR and iPSCs
The integration of CRISPR technology with induced pluripotent stem cells (iPSCs) represents a transformative shift in genetic engineering. This combination isnβt just about improving already existing technologies. It presents a new paradigm through which researchers can manipulate and examine cellular functions with distinct precision. Understanding this synergy can illuminate pathways for innovative therapeutic strategies and enhanced research methodologies.
Integrating CRISPR with iPSC Technology
Integrating CRISPR with iPSC technology involves applying CRISPRβs gene-editing capabilities to cells that have already been reprogrammed to an embryonic-like state. iPSCs, derived from somatic cells, possess the ability to differentiate into any cell type. This characteristic is crucial for applying CRISPR gene editing, as it allows for targeted genetic modifications that can be observed across various cell lineages. The process typically begins with reprogramming somatic cells, such as skin cells, into iPSCs using specific factors. Once established, researchers can then apply CRISPR techniques to edit the genome of these iPSCs as needed.
The key to this integration lies in the precise targeting capabilities of the CRISPR system. CRISPR-Cas9, for instance, employs a guide RNA to locate specific DNA sequences within the iPSCs. Once identified, the Cas9 enzyme introduces a double-strand break, allowing for targeted modifications, whether it be knocking out genes or inserting new sequences. This method fosters tailored approaches in genetic research and potential therapies.
Advantages of Combining CRISPR and iPSCs
The merger of CRISPR and iPSCs offers several notable advantages:
- Enhanced Precision: CRISPR allows for accurate modifications which can be validated in iPSCs, minimizing off-target effects.
- Versatility: iPSCs can differentiate into various cell types, allowing researchers to study gene function across different tissues.
- Regenerative Potential: Modifying iPSCs with CRISPR could lead to advancements in regenerative medicine, repairing or replacing damaged tissues more effectively.
- Disease Modeling: The combination enables the creation of more accurate disease models by introducing specific mutations associated with certain conditions.
These advantages signify a profound shift in how researchers can approach both basic biological questions and complex therapeutic challenges.
Case Studies and Current Research
Numerous studies have explored the practical applications of combining CRISPR and iPSCs. For example, researchers have successfully used CRISPR to correct genetic mutations in iPSCs from patients with sickle cell disease. In this case, the therapeutic goal is to rectify the underlying genetic defect in the patientβs own cells, enhancing the legitimacy of regenerative approaches.
Additionally, scientists are examining how this technology can be applied in neurodegenerative diseases like Alzheimerβs. By facilitating the editing of iPSCs to reflect disease states, researchers can observe the effects of potential treatments in a controlled environment.
Current research continues to expand the boundaries of these technologies. Investigations are focusing on how modifications can lead to better outcomes in transplantation medicine and novel avenues for gene therapy. Studies are busy actively to establish the full potential of CRISPR-edited iPSCs in clinical settings.
The combination of CRISPR and iPSCs provides unprecedented opportunities to tackle some of the most challenging questions in biotechnology and medicine today.
Therapeutic Applications of CRISPR iPSCs
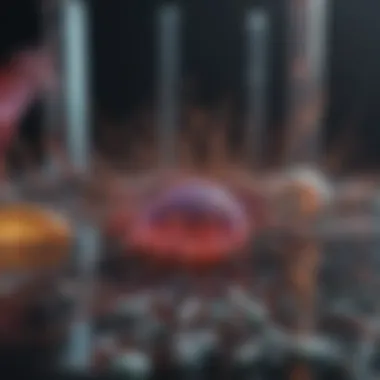
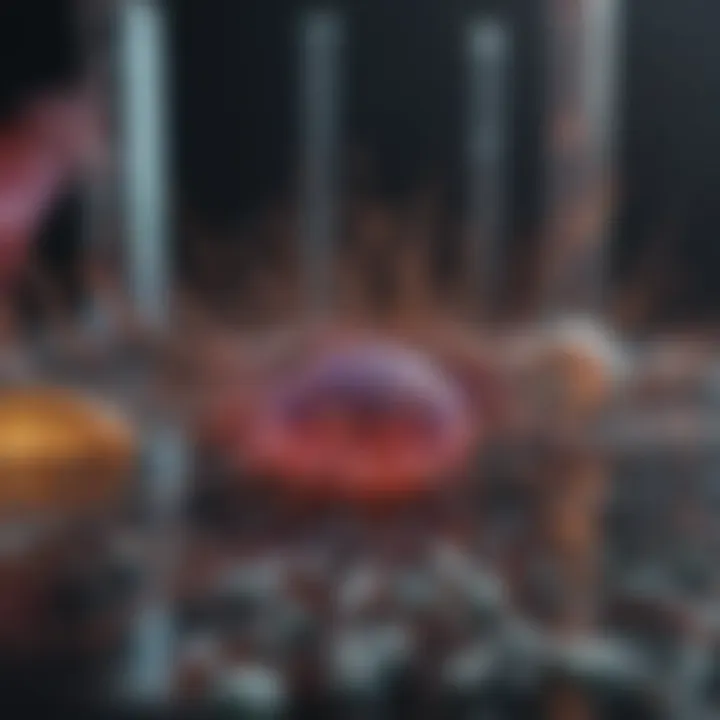
The exploration of therapeutic applications surrounding CRISPR and induced pluripotent stem cells (iPSCs) represents a pivotal aspect of modern biomedical research. These technologies open various pathways for treatment and understanding of numerous diseases. By delving into this topic, we uncover how these methodologies not only advance medicine but also reshape clinical practices.
Regenerative Medicine
Regenerative medicine stands at the forefront of the therapeutic applications of CRISPR iPSCs. By leveraging the unique properties of iPSCs, researchers can generate patient-specific cells for potential regeneration of damaged tissues and organs.
- Tissue Repair: iPSCs can differentiate into various cell types, offering potential for healing injuries or degeneration in tissues, such as heart or nervous tissue.
- Organ Transplantation: The use of patient-derived cells could minimize rejection risks associated with organ transplants.
- Personalized Therapies: Each patientβs cells can be modified to enhance effectiveness, sparking a paradigm shift towards personalized medicine.
However, technical hurdles and ethical considerations must be addressed. Regulatory pathways and long-term viability of these cells require further exploration.
Disease Modeling
Disease modeling using CRISPR and iPSCs represents an innovative approach to studying complex diseases. iPSCs not only provide a platform for studying disease mechanisms but also allow for testing of therapeutic interventions in vitro before they are applied in live subjects.
- Understanding Mechanisms: By inducing specific mutations in iPSCs, researchers can recreate disease conditions. This method is particularly useful in neurodegenerative diseases like Alzheimerβs.
- Drug Screening: iPSCs can be utilized for high-throughput screening of potential drugs, significantly expediting the discovery of effective treatments.
- Patient-specific Models: Using iPSCs derived from patients with specific genetic backgrounds allows for tailored research on individual responses to drugs.
These strategies facilitate a more profound understanding of disease pathology and therapeutic dynamics, potentially leading to precise medical interventions.
Gene Therapy Perspectives
Gene therapy perspectives emerge as one of the most compelling applications in the interplay between CRISPR and iPSCs. By utilizing CRISPR for genetic modifications in iPSCs, researchers can correct mutations at the source before deriving specific cell types for therapy.
- Correction of Genetic Defects: By targeting and editing genes in iPSCs, lifelong genetic diseases such as cystic fibrosis can potentially be treated.
- Safety and Efficacy: Using iPSCs offers an opportunity to assess gene modifications safely in vitro before moving into clinical trials.
- Advancements in Treatment: This approach signifies a stride towards curing genetic disorders rather than merely managing symptoms, which has profound implications for patient care.
The full realization of these therapeutic applications hinges on overcoming current challenges in the fields of ethics, safety, and regulatory measures, but the potential offers considerable hope for future advancements in medicine.
Ethical Considerations
The intersection of CRISPR technology and induced pluripotent stem cells (iPSCs) introduces a wealth of possibilities, but also significant ethical considerations. These include concerns about gene editing, especially related to potential misuse. Furthermore, the techniques involved in creating iPSCs raise questions about the characteristics of human life and the moral status of these cells. Addressing ethical issues is crucial in guiding research while safeguarding public interest.
Ethical Implications of CRISPR Technology
CRISPR technology allows precise editing of genetic material. While this can lead to breakthroughs in medicine, it raises ethical dilemmas. Key implications include the possibility of creating designer babies. The temptation to modify genes for enhanced traits can lead to social inequality. Concerns exist about unintended consequences of gene editing, such as off-target effects, where unintended parts of the genome may be altered. This could have unforeseen ramifications on the health of future generations.
Morality of iPSC Research
Induced pluripotent stem cells provide revolutionary opportunities in medicine. However, the morality of conducting research on these cells involves careful consideration. Since iPSCs are derived from somatic cells, they represent adult tissue that has been reprogrammed. This is distinct from embryonic stem cells, which often face more scrutiny. The moral concerns surrounding iPSC research also include the implications of creating cells that may mimic embryonic development. There is a pressing need for clear ethical guidelines that define the acceptable limits in iPSC research.
Regulatory Frameworks
As CRISPR and iPSC technologies evolve, robust regulatory frameworks must be established. Governance is essential in maintaining ethical standards and ensuring safety. Various countries have implemented different policies regarding gene editing. For example, in the United States, the National Institutes of Health and the Food and Drug Administration oversee research and clinical applications. In Europe, the European Medicines Agency evaluates therapeutic uses. Clear regulations can help prevent misuse and promote responsible scientific inquiry, allowing us to reap the benefits while minimizing risks.
"Navigating the ethical landscape of CRISPR and iPSCs is paramount to fostering innovation responsibly."
Establishing ethical norms in research and applications will facilitate a balanced approach towards genetic technologies.
Challenges and Limitations
The integration of CRISPR technology and induced pluripotent stem cells (iPSCs) offers promising avenues in genetic engineering. However, this frontier comes with its own set of challenges and limitations that are crucial to understanding for both practitioners and researchers in the field. Addressing these obstacles is essential for optimizing therapeutic applications and ensuring the ethical use of these powerful tools in medicine and research.
Technical Challenges in CRISPR Applications
CRISPR technology is not without its technical hurdles. The most significant challenge lies in ensuring precision within gene editing. Off-target effects, where the CRISPR system unintentionally alters unintended genetic sequences, raise concerns about the safety and efficacy of the technology. Furthermore, the delivery mechanisms of CRISPR components into specific cells or tissues also present issues. Traditional viral vectors can sometimes trigger immune responses or integrate into the genome unpredictably.
Moreover, the possible mutations resulting from edited genes can complicate the biological outcomes. For instance, altering a gene that affects cell proliferation inadvertently may lead to tumor formation. Hence, researchers must refine methodologies and carefully choose vector systems to minimize these risks and improve specificity in CRISPR applications.
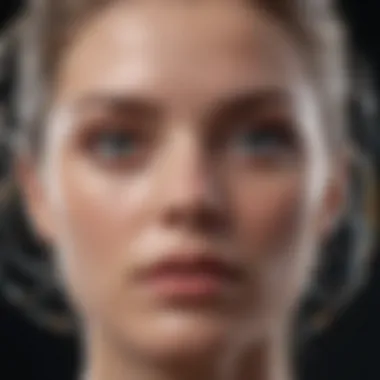
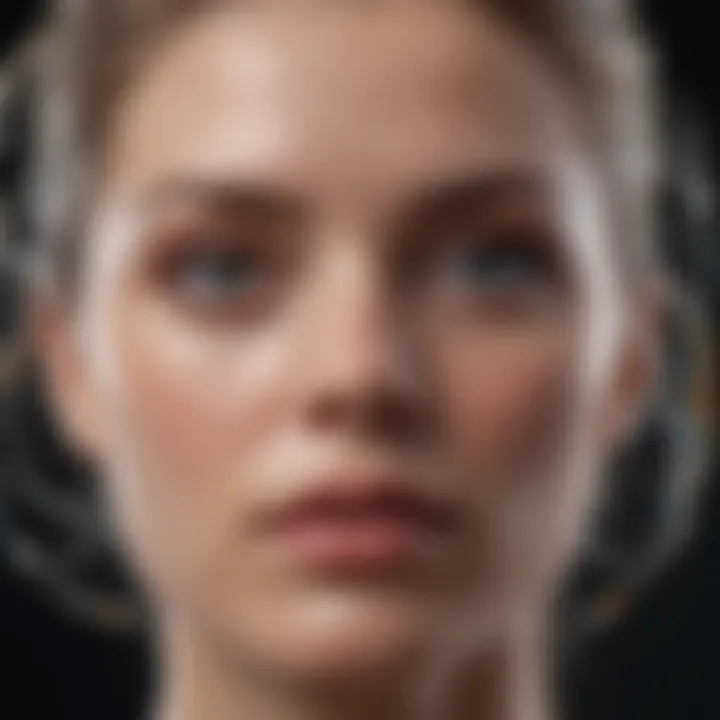
Limitations of iPSC Technology
While iPSCs exhibit remarkable potential, they are not a magical solution for all clinical problems. One of the key limitations is the risk of teratoma formation, which can occur when iPSCs are not fully differentiated before transplantation. This uncontrolled growth poses significant risks when considering therapeutic applications, especially in regenerative medicine.
Additionally, the efficiency of reprogramming somatic cells into iPSCs varies greatly and can influence the quality and functionality of the resulting cells. Variability in gene expression patterns between different iPSC lines also impacts consistency, which can be a critical factor in experimental and clinical settings.
Furthermore, the long-term behavior of iPSCs after transplantation remains poorly understood. Their stability and ability to differentiate into the desired cell types in vivo require deeper investigation to ensure their successful application in therapies.
Future Challenges in the Field
As CRISPR and iPSC technologies advance, they will inevitably face new challenges. One potential area of future difficulty involves regulatory frameworks which often lag behind technological innovations. The lack of clear regulations can hinder the progress of research and clinical trials as scientists navigate ethical considerations related to genetic modifications and stem cell use.
Additionally, public perception of genetic engineering and stem cell research continues to shape funding and regulatory landscapes. Balancing scientific advancement with societal concerns will be vital to progress in the field.
Moreover, interdisciplinary collaboration will play an important role in overcoming these challenges. However, differences in expertise and communication between fields such as molecular biology, ethics, and engineering may complicate efforts to develop comprehensive solutions.
"Navigating the technical and ethical landscape of CRISPR and iPSCs will require continued vigilance and adaptability by researchers and institutions."
In summary, while the potential of CRISPR and iPSCs is vast, it is imperative to address their challenges and limitations to enhance their effectiveness in genetic engineering and therapy.
Future Directions of CRISPR iPSC Research
The future of CRISPR technology combined with induced pluripotent stem cells (iPSCs) holds significant promise for advancing genetic engineering. This section delves into various elements that illuminate the benefits and considerations impacting the research in this area. As advancements continue to emerge, researchers must remain cognizant of the potential ethical implications, regulatory challenges, and the necessity for collaborative approaches.
Emerging Trends in CRISPR Technology
In recent years, several notable trends have surfaced in the CRISPR technology space. The development of base editing is one of them, offering the ability to convert one DNA base into another with remarkable precision. This technique reduces off-target effects and enhances accuracy in genetic manipulations. Another compelling trend is the advent of CRISPR systems that can target RNA instead of DNA. This flexibility expands the utility of gene editing beyond permanent alterations to potential therapeutic interventions for diseases caused by pathogenic RNA.
Moreover, CRISPR systems are being refined for use in therapies that directly target genetic disorders. Research around delivery mechanisms, particularly in vivo methods, is crucial. Researchers are exploring viral vectors and nanoparticle-based systems as effective carriers for CRISPR components. The integration of artificial intelligence into CRISPR design processes also stands out as a transformative element, enabling quicker and more efficient identification of target sites for gene editing.
Potential Developments in iPSC Research
The evolution of iPSC technology is pivotal for the field of regenerative medicine. One potential direction for iPSC research is its application in personalized medicine, allowing cell-based therapies tailored to individual patients. This approach can be transformative in areas such as oncology and rare genetic diseases, where bespoke treatments could greatly enhance efficacy.
Another promising development involves optimizing differentiation protocols to produce specific cell types consistently. This can facilitate advancements in drug discovery and disease modeling. The potential for iPSCs to generate organoids, miniaturized and simplified versions of organs, is also being pursued. These organoids can serve as valuable tools in understanding complex diseases and assessing drug responses.
Interdisciplinary Research Opportunities
Collaboration across disciplines remains a critical aspect of advancing CRISPR and iPSC research. Partnerships between biologists, bioengineers, and computational scientists can catalyze breakthroughs that may not be achievable within isolated silos. For instance, bioinformatics can play a supportive role in analyzing large datasets generated during CRISPR screenings, enabling researchers to identify potential targets more efficiently.
Furthermore, integrating ethical and regulatory perspectives into scientific research is crucial. Interdisciplinary teams must include ethicists to navigate the moral complexities associated with gene editing and stem cell research. Public engagement also represents an important avenue to understand societal perspectives on these technologies.
"As CRISPR technology and iPSCs converge, the need for interdisciplinary collaboration is paramount to navigate the complexities of scientific advancement."
Overall, the exploration of future directions in CRISPR and iPSC research signifies an exciting and transformative phase in genetic engineering, with substantial implications for therapy development and ethical considerations.
End
The culmination of this article emphasizes the profound impact of CRISPR technology and induced pluripotent stem cells (iPSCs) in the realm of genetic engineering. Understanding the synergy between these two revolutionary tools not only illuminates their potential but also underscores the necessity for a thorough grasp of their implications in scientific research and clinical applications.
Recap of CRISPR iPSC Synergy
At the heart of this discussion is the complementary nature of CRISPR and iPSC technologies. CRISPR provides a powerful mechanism for precise gene editing, while iPSCs offer a versatile platform for studying cellular behavior and development. This combination facilitates a new gateway to regenerative medicine. For instance, through the use of CRISPR, researchers can correct genetic disorders at the cellular level, using iPSCs derived from the patient's own somatic cells. This allows for the possibility of personalized therapies that minimize rejection rates and side effects. The regenerative potential is vast, including applications in treating degenerative diseases, facilitating organ regeneration, and advancing personalized medicine.
"The integration of CRISPR with iPSCs presents an unprecedented opportunity to redefine approaches in regenerative medicine and genetic disorders."
Final Thoughts on Research Implications
The future of CRISPR and iPSC research holds promise for transformative changes in therapeutic practices. However, it is vital to remain cognizant of the ethical implications and potential risks associated with these technologies. Researchers must engage with ethical frameworks to ensure responsible usage in human applications. The ongoing development of CRISPR and iPSC methodologies calls for interdisciplinary collaboration, as advancements in bioinformatics, molecular biology, and ethical discourse will shape the path forward. Continuous dialogue among scientists, ethicists, and the public will be crucial in navigating the challenges and opportunities that arise.
In summary, the intersection of CRISPR technology and iPSCs marks a defining moment in genetic engineering. The integration of these innovative approaches has the potential to redefine disease treatment, personalize medicine, and unravel complex biological systems, ultimately enhancing the quality of life for many individuals.