Mechanisms and Applications of CO2 Rockets Explained
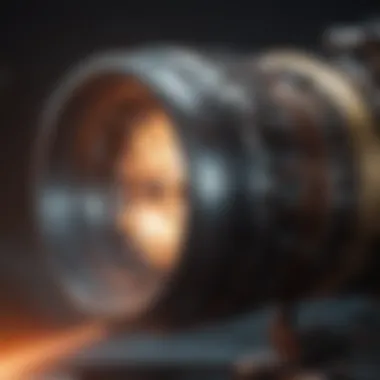
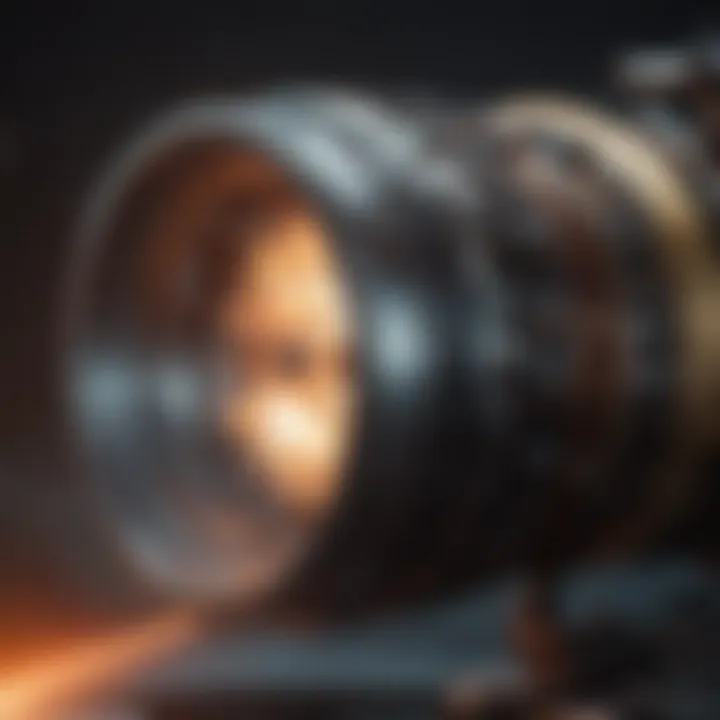
Intro
The concept of rockets powered by carbon dioxide is fascinating, yet it often gets overshadowed by the more glamorous, high-tech propulsion systems associated with space travel. However, CO2 rockets offer an excellent entry point into the world of rocketry, especially for students and educators. They are safe, simple to construct, and can serve a multitude of educational and experimental purposes.
Understanding how CO2 rockets work demands a glimpse into both the science of propulsion and the engineering aspects that underpin their design. This article aims to demystify the mechanics involved in these systems and highlight their real-world applications.
Research Overview
Summary of Key Findings
CO2 rockets generate thrust through the rapid release of carbon dioxide gas. When a pressurized container is ruptured, the gas expands rapidly, propelling the rocket in the opposite direction due to Newton’s third law of motion, which states that for every action, there is an equal and opposite reaction. Key findings in this field show how variations in design and material impact the efficiency and structural integrity of these rockets.
Research Objectives and Hypotheses
The main objectives of exploring CO2 rockets are:
- To analyze the principles of thrust generation via gas expansion.
- To investigate the relationship between construction materials and rocket performance.
- To evaluate the educational impact of CO2 rockets in learning environments.
Hypotheses include:
- The materials used in construction significantly influence the maximum altitude achieved.
- Hands-on experimentation with CO2 rockets enhances comprehension of fundamental physics concepts, such as force, motion, and pressure.
Methodology
Study Design and Approach
A mixed-method approach is employed, integrating both experimental and observational studies. By designing and launching multiple CO2 rocket prototypes, important data can be gathered regarding performance metrics, including height achieved and stability during flight. Observational studies offer insights into how different educational contexts influence learning outcomes.
Data Collection Techniques
Data collection will employ both quantitative and qualitative methods:
- Quantitative Data: Measurements taken from altimeters, pressure gauges, and other instruments during rocket launches will provide numerical data enabling statistical analysis.
- Qualitative Data: Surveys and interviews with participants will be used to gauge comprehension and interest levels regarding rocketry and its associated scientific principles.
Closure
Understanding the mechanisms and applications of CO2 rockets not only sparks interest in rocketry but also fosters deeper engagement with scientific principles. The insights gleaned from experiments and educational activities reveal just how versatile and valuable these systems can be in both theoretical and practical settings.
Intro to CO2 Rockets
The significance of CO2 rockets in the realm of rocketry cannot be forgotten. With growing concerns over environmental issues and the need for efficient propulsion systems, CO2 rockets offer a unique blend of creativity and practicality. This section serves to lay foundational knowledge before diving deeper into the intricacies of their design and application.
Definition and Purpose
To define a CO2 rocket, it’s essential to understand that these are propulsion devices utilizing carbon dioxide as their primary driving force. The main purpose behind using CO2 in rockets is its availability and relative safety compared to other propellants. CO2 rockets harness the rapid expansion of carbon dioxide gas to create thrust.
In practical use, these rockets are often an entry point for students and amateur enthusiasts looking to latch onto basic rocketry principles. They provide a hands-on experience with physics and engineering concepts. Flying a CO2 rocket may seem trivial, but it serves as a powerful educational tool, bridging theoretical knowledge with real-world applications. When initiated, the gas expands, forcing it out of the nozzle and propelling the rocket into the sky.
Historical Context
The history of CO2 rockets is, in many ways, a tale of innovation in the face of simplicity. The use of gas as a propulsion mechanism can be traced back centuries; however, the application of carbon dioxide emerged more prominently in the educational sector since the late 20th century. People realized that utilizing a non-toxic gas like CO2 could simultaneously teach learners about basic rocketry while also emphasizing safety.
Initially, these rockets were seen more in labs and educational environments rather than in commercial forms. This was primarily due to their ease of construction. A typical CO2 rocket could be constructed using lightweight materials, often just plastic bottles and fittings. Schools around the globe took notice, integrating CO2 rocket launches as exciting activity in science curriculums.
"The CO2 rocket acts as a gateway for budding scientists, providing a chance to explore principles of propulsion without the complexities of chemical reactions."
The advancements in design and material application have since propelled CO2 rockets into a wider spectrum of uses—from hands-on educational tools to iterative prototypes standing at the forefront of accessible rocketry. With a mix of historical ingenuity and modern advancements, CO2 rockets continue to capture imaginations in education and research alike.
Fundamental Principles of Rocketry
In the realm of rocket science, understanding the fundamental principles of rocketry is paramount. These foundational concepts not only set the stage for the technical aspects of CO2 rockets but also ensure that the designs are both effective and safe. At its core, rocketry is deeply intertwined with physics, particularly the laws governing motion and energy. Thus, it becomes essential to explore the specific elements that underpin this fascinating field, such as Newton's laws and thrust generation, and to understand their implications for CO2 propulsion systems.
The principles of rocketry offer insight into how and why rockets behave the way they do. They help engineers and researchers evaluate the efficiency, weight, cost, and performance characteristics of their creations. All of this is vital for anyone working with CO2 rockets, whether they are students conducting simple experiments or professionals engaged in cutting-edge research and development.
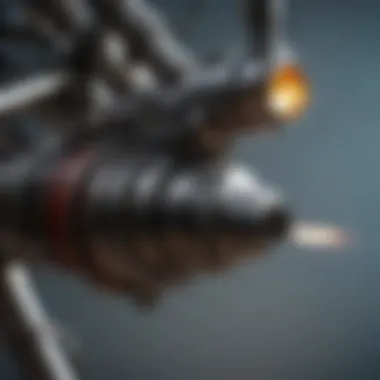
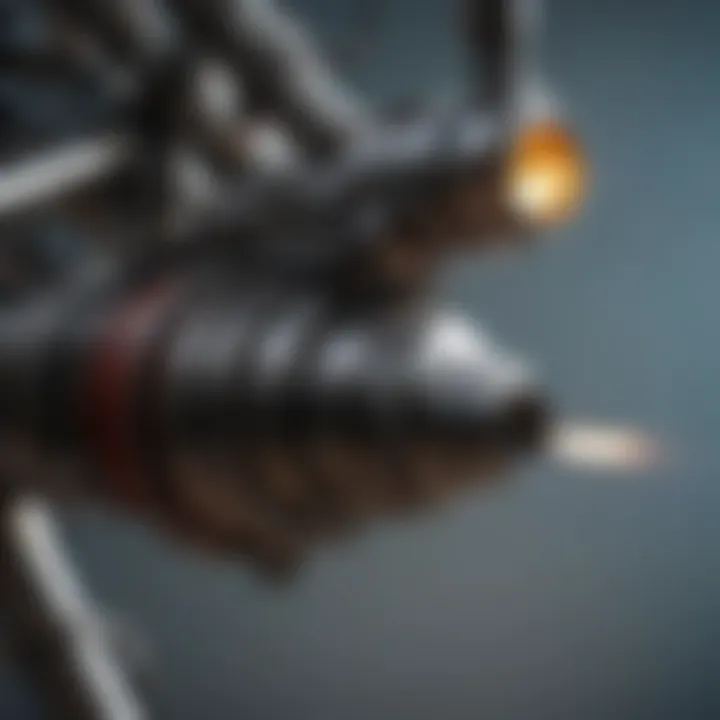
Newton's Third Law of Motion
Newton's Third Law of Motion is a pillar of rocketry: "For every action, there is an equal and opposite reaction." In simpler terms, this means that when a rocket expels gas in one direction, it is propelled in the opposite direction. This principle explains why when carbon dioxide is rapidly released as a propellant, the rocket is driven forward. For CO2 rockets, understanding this law is crucial. The efficiency of propellant expulsion directly impacts the thrust produced, which is essential for achieving lift-off and sustaining flight.
In practical terms, when discussing a CO2 rocket, the rapid release of the gas—often from a soda bottle—produces a force that propels the rocket upward. The design must ensure that the gas is released efficiently, maximizing thrust while minimizing unwanted turbulence or inefficiencies.
"The wonders of rocketry lie in its adherence to the laws of nature, reminding us that every action has its counterpart."
Thrust Generation
Delving into thrust generation, we must first acknowledge that its efficacy directly hinges on the amount and speed of the expelled gases. When we talk about CO2 rockets, we are primarily dealing with pressurized carbon dioxide as a propellant. When the gas is released suddenly through a valve or nozzle, it not only reduces pressure inside the bottle but also generates thrust as the gas rushes out.
Key factors influencing thrust generation include:
- Gas Pressure: Higher pressure leads to faster gas expulsion.
- Nozzle Design: The shape and size of the nozzle determine how effectively the gas can be converted into thrust.
- Mass Flow Rate: This measures how much gas is expelled over a particular time; it's directly related to the thrust produced.
The thrust-to-weight ratio becomes crucial when analyzing a CO2 rocket's flight capabilities. A rocket needs to have sufficient thrust to overcome gravity for a successful launch. If the thrust is less than the weight, then the rocket simply won't rise. Each design consideration—from the materials used to construct the rocket to the velocity of the gas released—plays into this delicate balance.
In summary, the fundamental principles of rocketry are foundational to the operations and applications of CO2 rockets. Understanding Newton's Third Law and effectively managing thrust generation is not only essential for those involved in teaching or researching rocketry but also for engineers and hobbyists alike who are putting these principles into practice.
Chemical Basis of CO2 Propulsion
The significance of understanding the chemical basis of CO2 propulsion cannot be overstated in the context of rocket systems. It is the foundation on which the operational efficiency of CO2 rockets rests, dictating both their performance and applicability. The reactions involved in the formation and utilization of carbon dioxide as a propellant reveal critical insights into how we can maximize thrust while minimizing waste, a concern that looms large in both educational and professional settings.
Formation of Carbon Dioxide
Carbon dioxide formation occurs through various chemical reactions, most notably those involving organic materials and combustion processes. In simple terms, when a carbon-containing fuel undergoes complete combustion in an oxygen-rich environment, carbon dioxide is produced as a primary byproduct. This reaction can be summed up with the following equation:
[\textC + \textO_2 \rightarrow \textCO_2]
Here’s a more practical view: burning substances like sugar, wood, or even certain plastics can yield CO2 among other gases. This particular aspect of CO2 propulsion is crucial for its applications in educational settings, where students can directly observe these processes in action, using safe materials to generate thrust. The beauty of CO2 lies in its abundance and the relative simplicity of the ingredients needed for its creation.
However, it's not just about creating CO2. The rate at which this gas is produced impacts the thrust generated. Thus, understanding and controlling factors like temperature and fuel type can significantly enhance the operational efficiency of CO2 rockets.
Combustion Reactions
When we shift our focus to combustion reactions, it’s essential to acknowledge that they encompass more than just the formation of carbon dioxide. During combustion, chemical bonds within fuel molecules are broken down, releasing energy in the form of heat and kinetic energy. This release is what ultimately propels a rocket skyward. For CO2 rockets, the right blend of fuel and oxidizer not only produces sufficient thrust but also manages the generation of gases effectively, with fewer pollutants compared to traditional chemical rockets.
To delve deeper, let’s look at a generalized combustion reaction involving a hydrocarbon fuel:
[\textC_x\textH_y + z \textO_2 \rightarrow a \textCO_2 + b \textH_2\textO + extEnergy ]
In this equation, we see variables representing the composition of fuels. Consequently, the choices made regarding these fuels will affect the rate of CO2 production during the combustion phase. For example, ethanol, a common alternative fuel, not only produces energy but also generates other products along with CO2, making it vital to calculate the efficiency of its usage accurately.
"Understanding combustion is the key to unlocking the potential of CO2 as a propellant, bridging the gap between theoretical knowledge and practical application."
As we begin to apply these chemical principles in real-world settings, it becomes clear that both the formation of carbon dioxide and the efficiency of combustion reactions play a pivotal role in the propulsion mechanisms of CO2 rockets. The experiments designed around these concepts pave the road for innovative applications in educational environments and beyond, nurturing the minds that will shape future generations of rocketry.
Design Considerations
Design considerations play a pivotal role in the development and performance of CO2 rockets. The intricate balance between materials, structural integrity, and optimal safety must be acknowledged to create a functional and reliable propulsion system. A thoughtfully designed rocket does not just ensure efficiency but also enhances the overall educational and experimental experience for users.
When engaging with CO2 rockets, several specific elements come into play. To begin with, understanding the nature of the materials used in construction is essential, as each component contributes significantly to the rocket's performance, weight, and durability. Moreover, keen attention to structural integrity ensures that the rocket can withstand extreme conditions while maintaining safety for all involved.
Additionally, design considerations can influence not just the immediate success of a launch but also the rocket's adaptability for future modifications or variations in usage. Evaluating weight distribution, thrust-to-weight ratio, and even aesthetics can further refine the project outcomes. Each consideration signifies a step towards professionalism in engineering and scientific literacy, especially for students and educators involved in hands-on learning.
Materials Used in Construction
The choice of materials for CO2 rockets is as eclectic as it is vital. Selecting the right combination not only determines the rocket's weight and aerodynamic efficiency but also its ability to perform under pressure. Commonly used materials include lightweight plastics like polyethylene or polycarbonate, which offer a good strength-to-weight ratio.
Other viable options involve metal components such as aluminum for structural parts that require more durability. However, utilizing cardboard or paper can also be a suitable choice for educational rockets that prioritize cost-effectiveness while still delivering excellent performance in test environments. It is important to recognize the benefits and downsides of each material:
- Weight: Lighter materials typically yield better flight, while heavier components can stabilize the rocket but may hinder vertical ascent.
- Cost: Budget constraints often dictate the choice of materials in educational settings, where more affordable options are favorable.
- Durability: Elements designed to endure multiple launches must incorporate materials known for their resilience and longevity.
- Safety: Materials should be non-toxic, especially for educational uses with younger cohorts.
Structural Integrity and Safety
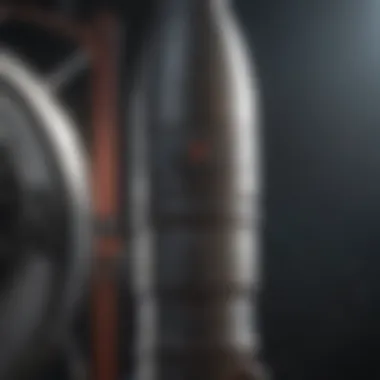
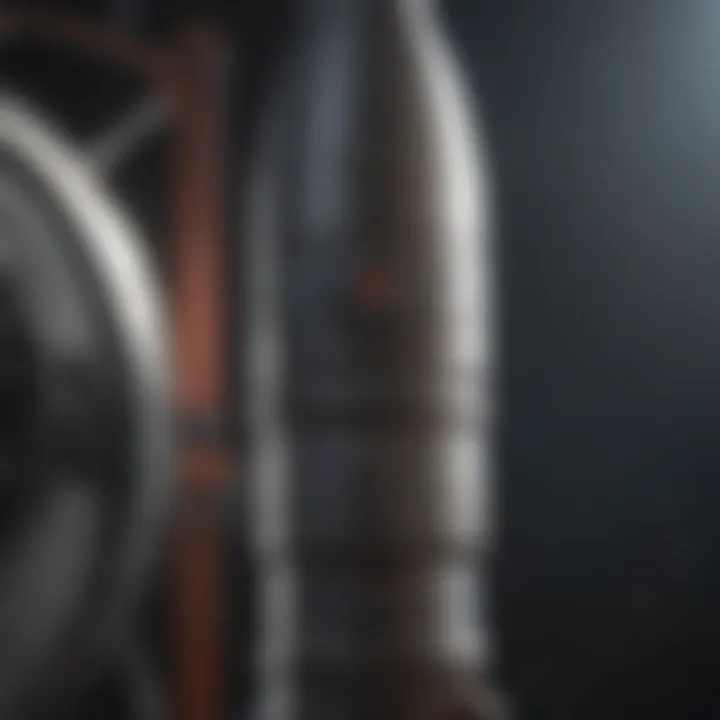
Structural integrity in CO2 rocket design cannot be overstated. It is the backbone of any rocketry project, ensuring that all components withstand the forces exerted during launch, flight, and recovery. Insufficient attention to structural integrity can lead to failures that jeopardize not just the rocket but also the safety of observers and participants.
Key considerations regarding structural integrity include:
- Stress Resistance: The materials selected must handle stresses induced by thrust and aerodynamic forces without deforming.
- Component Connections: Joints and attachments among different rocket parts need to be robust to prevent disassembly mid-flight. Techniques such as using epoxy or screws are effective for this purpose.
- Weight Distribution: An evenly distributed weight contributes to stability, reducing the risk of tumbling or failure during ascent.
- Safety Features: Adding features like shock absorbers can help protect key components during recovery, further ensuring safe operations.
"The accuracy in design translates into reliability in performance. Skimping on these considerations might save time but can lead to costly mistakes in the long run."
Characteristics of CO2 Rockets
Understanding the characteristics of CO2 rockets is crucial as it provides insights into their performance, operational capabilities, and practical uses. When it comes to rocket systems, especially those utilizing carbon dioxide as a propellant, knowing how they behave under various conditions can be pivotal. Two significant characteristics stand out: thrust-to-weight ratio and flight duration and altitude. These elements not only determine a rocket's efficiency but also its effectiveness in meeting specific mission objectives.
Thrust-to-Weight Ratio
The thrust-to-weight ratio (TWR) plays a fundamental role in any rocket's design and operational success. This ratio is measured by dividing the total thrust produced by the rocket engines by the weight of the rocket itself. A TWR greater than one signifies that a rocket can lift off, while a value below one indicates it cannot.
For CO2 rockets, typically constructed from light yet sturdy materials like plastic or metal alloys, optimizing the TWR is essential. The challenge lies in generating sufficient thrust while keeping the overall weight minimal. Factors impacting this ratio may include:
- Engine Design: Different configurations of engine nozzles and combustion chambers can affect thrust production.
- Fuel Type: The purity and containment of carbon dioxide can markedly impact its propulsion efficiency.
- Total Mass: Incorporating lightweight materials aids in lowering weight without compromising structural integrity.
"A CO2 rocket with a favorable thrust-to-weight ratio can achieve significant heights, showcasing the effectiveness of careful design and engineering in rocketry."
Flight Duration and Altitude
Flight duration and altitude are critical indicators of a rocket's performance. They denote how high the rocket can ascend and how long it remains in flight, which directly correlates with the design and operational parameters.
- Flight Duration: This refers to the time from launch until the rocket reaches its apex or begins descent. For CO2 rockets, flight duration can range from a few seconds to a couple of minutes based on several factors:
- Altitude: Altitude is often the primary goal in rocket launches. Elevated altitudes signify successful thrust and effective flight dynamics. Some considerations that influence altitude include:
- The amount of CO2 used and how quickly it is expelled.
- The rocket's aerodynamic design, influencing drag and stability in the air.
- Environmental conditions, such as wind speed and air density.
- State of Propellant: The pressure of CO2 in the rocket impacts how efficiently it can be released, affecting overall ascent.
- Rocket Configuration: A streamlined form can minimize air resistance, allowing for higher flight.
- Launch Angle: The trajectory angle can maximize altitude by balancing gravity and thrust.
In sum, both thrust-to-weight ratio and flight duration with altitude not only characterize CO2 rockets but also dictate their feasibility for educational purposes and experiments. Understanding these concepts helps students, researchers, and professionals alike grasp the intricacies of rocketry and the relevance of CO2 propulsion in practical applications. As science continues to advance, honing these characteristics will pave the way for future innovations in rocket technology.
Applications of CO2 Rockets
The applications of CO2 rockets span across various fields, showcasing their versatility and importance in both educational and research settings. Understanding and utilizing CO2 rockets not only fosters enthusiasm for science but also presents practical solutions to engineering challenges. Their implementation benefits curriculum development, encourages hands-on learning, and supports advancements in aerospace research. By breaking down these applications, we illuminate the significance of CO2 rockets within educational frameworks and scientific exploration.
Educational Use in STEM
In recent years, CO2 rockets have become increasingly popular in STEM education. Educators leverage their simplicity and the fundamental principles of physics they illustrate to engage students. Students learn critical concepts like thrust, aerodynamics, and propulsion while participating in hands-on rocket building and launching activities.
- Hands-on Learning: Building and launching CO2 rockets allows students to experience engineering principles first-hand. They understand theoretical concepts by applying them in practice, bridging the gap between classroom learning and real-world applications.
- Teamwork and Collaboration: Such activities encourage teamwork as students work in groups to plan, construct, and execute their rocket launches. This collaboration fosters communication skills and teaches the value of collective problem-solving.
- Creativity and Innovation: Each student or group brings unique designs to the table, allowing for creative experimentation. This not only cultivates innovation but also highlights the iterative process of engineering—testing, failing, and adapting designs for better performance.
Educators report increased engagement levels when CO2 rockets are part of the curriculum. Students who might otherwise shy away from science find themselves captivated by the thrill of watching their creations take flight.
Research and Development
Beyond educational environments, CO2 rockets hold potential in serious research and development scenarios. Their unique propulsion mechanism provides a safe and controllable means for experimentation, particularly valuable in materials science and propulsion techniques.
- Testing New Materials: Researchers can utilize CO2 rockets to evaluate how different materials perform under varying conditions. By subjecting structural components to the stresses of flight, scientists gain insights essential for improving material resilience in aerospace applications.
- Propulsion System Innovations: Development in propulsion technologies can be tested using CO2 rockets as a proof of concept. Adjustments made to nozzle designs or pressure systems can be investigated more safely in this controlled environment, potentially leading to significant advancements.
- Sustainable Practices: In a world increasingly focused on environmental concerns, CO2 rockets may serve as a model for cleaner propulsion methods. As scientists explore alternatives to traditional propellants, the research conducted with CO2 could pave the way for innovations that lessen environmental impact.
"CO2 rockets exemplify the blend of education and innovation, fulfilling needs in both learning and research while emphasizing hands-on experience and applying theoretical knowledge in practical scenarios."
Experimental Methods of Rocket Testing
Testing methods for CO2 rockets serve a vital role in understanding how these systems operate under various conditions. The experiments help validate theoretical principles, ensuring that designs perform as expected in the real world. By rigorously analyzing the results, educators, students, and researchers can troubleshoot problems, improve rocket performance, and refine future designs. This section will explore the specifics of launch pad setup and data collection techniques, both crucial components of the experimental testing process.
Launch Pad Setup
Establishing a reliable and efficient launch pad is the first step to a successful rocket test. A launch pad must support the weight of the rocket while allowing it to achieve a vertical launch. Proper setup includes several considerations to ensure both safety and functionality:
- Location: The chosen site should be clear of obstacles, ensuring the rocket can ascend without interference.
- Surface Stability: A flat and sturdy surface is essential to prevent any tipping or destabilization as the rocket ignites.
- Support Mechanisms: Rails or platforms can help guide the rocket during takeoff, promoting a straight ascent.
- Safety Protocols: It’s crucial to establish proper distance from the launch site for observers and to have fire extinguishers on hand.
In essence, the launch pad setup must be meticulously planned and executed, taking into account all variables to facilitate a smooth rocket launch. Addressing these elements ensures that tests are both safe and scientifically valid.
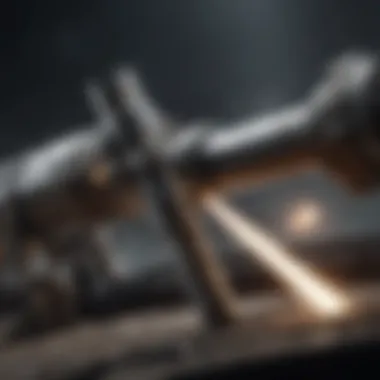
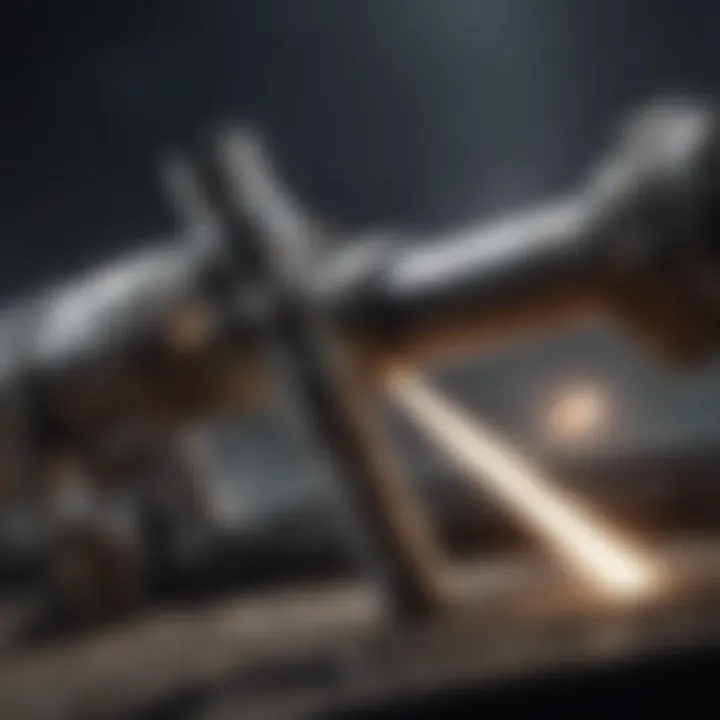
Data Collection Techniques
To accurately assess the performance of CO2 rockets, implementing robust data collection techniques is essential. Gathering the right data can reveal a wealth of information about the rocket’s operation, including speed, altitude, and Thrust-to-Weight Ratio. Some common strategies for data collection include:
- High-speed Cameras: These can capture the rocket's launch and flight profile, providing visual data to analyze.
- Altimeters: Devices that measure the altitude can give precise readings on how high the rocket travels.
- Accelerometers: These sensors collect data on the rocket’s acceleration, helping evaluate thrust efficiency.
- Launch Logs: Keeping detailed records of each test, including launch time, environmental conditions, and any anomalies, facilitates a thorough longitudinal analysis.
"Accurate data is the backbone of effective rocket testing; without it, one is shooting in the dark."
Challenges and Limitations
Understanding the challenges and limitations faced by CO2 rockets is crucial for anyone involved in their study or application. These hurdles not only affect the performance of the rockets but also influence their viability in various settings. By identifying these issues, designers and engineers can work toward improving efficiency and overcoming obstacles that may hinder broader adoption in educational and research contexts.
Efficiency of Propulsion
Propulsion efficiency is a major consideration in the design of CO2 rockets. Unlike traditional rocket fuels, carbon dioxide has unique properties that challenge conventional propulsion methods. The thrust produced by a CO2 rocket is sufficient for educational purposes, but it often falls short when compared to rockets using more advanced propellants. The core of this issue lies in the energy-density of the propellant. CO2 as a propellant does not release as much energy per unit mass when burned or when it transitions from a pressurized state to a gas phase, which limits how high and how fast these rockets can fly.
For example, when we look at the configurations used in various CO2 rocket designs, the thrust-to-weight ratio often needs careful management. If the rocket is too heavy for the thrust produced, it may struggle to lift off. Here are some factors that can impact the efficiency:
- Size and Weight: Heavier rockets require more thrust to reach the same altitude as lighter ones, making careful material selection vital.
- Nozzle Design: The design of the rocket nozzle influences how effectively gas expands and creates thrust. Adjusting the shape can lead to modest gains in performance.
- Operational Pressure: The pressure at which CO2 is stored affects its efficiency. Higher pressure can improve thrust, but safety becomes a bigger concern as the risk of tank rupture increases.
Environmental Considerations
When discussing rockets, the environmental impact is a crucial aspect that cannot be ignored. CO2 rockets, in particular, offer unique insights into environmental considerations. Unlike many traditional rockets that burn hydrocarbons and release harmful byproducts, CO2 rockets primarily release carbon dioxide, which is already present in the atmosphere. However, their operation still carries implications that must be evaluated carefully.
One major consideration is how the production and storage of propellants affect the environment. While using CO2 as a propellant may appear benign, sourcing this gas, especially through fossil fuel processes, can lead to a net increase in greenhouse gases. These factors should motivate researchers to consider alternative, more sustainable methods of sourcing CO2, such as capturing it directly from the atmosphere or using biogenic sources.
Additionally, factors such as noise pollution and safety during launches play a role in determining the feasibility of CO2 rockets for educational and commercial purposes. The noise generated during ignition and launch can be a nuisance, especially in urban areas. Safety regulations must be followed closely, particularly regarding the containment of pressurized gases.
In summary, while CO2 rockets stand at the intersection of education and innovation, they face challenges related to propulsion efficiency and environmental impact.
Understanding these limitations can provide a foundation for future advancements in rocket design that not only optimize performance but also adhere to environmental standards, shaping a more sustainable future in aerospace endeavors.
Future Prospects
The future of CO2 rockets holds a realm of possibilities that can enhance not only educational endeavors but also contribute to significant advances in various fields. As we pivot towards sustainable practices in engineering and science, the potential for CO2 rockets to play a pivotal role in this shift is undeniable. This section will unpack the trends and innovations that may shape the next generation of rocket technology.
Innovative Designs
When it comes to innovative designs in CO2 rockets, the sky is truly the limit. Engineers and scientists are continuously experimenting with new concepts that optimize efficiency. One interesting development is the use of 3D printing technology, which allows for the creation of complex and lightweight rocket structures. This method can lead to reductions in material use without compromising the integrity of the rocket.
Another wave of innovation is seen in the incorporation of recyclable materials. By employing biodegradable or easily recyclable substances, designers can minimize the environmental footprint associated with traditional rocket construction. Optionally, some researchers are looking into hybrid rocket designs, blending CO2 propulsion with other green technologies to achieve better performance metrics.
It is crucial to think about thermal management as well. Using advanced materials that can endure high temperatures and pressure variations will enhance reliability during launches. Likewise, the integration of sensor technology into the design helps track performance indicators in real time, providing invaluable data for improvements.
Potential for Broader Applications
As for broader applications, CO2 rockets have untapped potential that extends far beyond classroom demonstrations and niche research projects. They can be repurposed for environmental monitoring—think about launching small probes to gather air quality data at varying altitudes. The ability to propel these mini rockets at low costs opens doors for large-scale environmental studies that were previously too expensive to consider.
Using CO2 rockets in space exploration is another possibility. While they may not replace chemical propulsion systems entirely, they could serve as auxiliary payload delivery systems, particularly for small satellite deployments. The fact that carbon dioxide is abundant and can be generated from renewable sources adds another level of sustainability.
Industries focused on agriculture can also benefit. Imagine a scenario where CO2 rockets deliver targeted nutrients or bio-pesticides to hard-to-reach areas in farming landscapes. This precision application could greatly enhance efficiency and reduce chemical run-off, supporting both crop yields and environmental health.
"Innovative applications of CO2 rockets may redefine our approach to both education and practical problem-solving across various domains."
In summary, looking ahead, there’s ample room for growth in CO2 rocketry, whether it concerns efficient designs, sustainable materials, or multifaceted applications in different sectors. As research continues to unfold, the future looks promising for this area of rocketry.
Ending
The exploration of CO2 rockets not only sheds light on their operational mechanisms but also emphasizes their significance across various domains. Understanding how CO2 rockets operate helps to bridge theoretical knowledge with practical application. These systems serve as a bridge in educational contexts, sparking student interest in science, technology, engineering, and mathematics.
Summary of Findings
Throughout this article, we have meticulously traced the journey of CO2 rockets from basic design to their real-world implications.
- Basic Principles: By delving into Newton's laws and thrust generation, we established a foundational knowledge of rocketry mechanics.
- Chemical Properties: The significance of carbon dioxide as a propellant has been outlined, showcasing its unique formation processes, which arise from combustion reactions.
- Design and Safety: Material considerations and structural integrity measures were discussed to highlight how engineers ensure reliable performance.
- Applications: We’ve also illuminated their role in education and research, showcasing their unique ability to facilitate hands-on learning experiences.
- Challenges: Practical limitations in efficiency and environmental concerns were acknowledged, making this a balanced discussion.
These findings elucidate not just the working of CO2 rockets but their broader implications in fostering innovation and environmental awareness in rocketry.
Final Thoughts on CO2 Rockets
Looking ahead, the evolution of CO2 rockets may entail groundbreaking innovations that would enhance their design and application. As educators leverage these systems for hands-on experience, students might even fuel their creativity to think beyond existing limitations. The potential of CO2 rockets stretches into the realms of educational outreach and cutting-edge research. Yet, with innovation comes responsibility; we need to consider the environmental challenges that accompany propulsion technologies as we strive to harness the power of CO2.