Cancer Genomics Database: An In-Depth Exploration
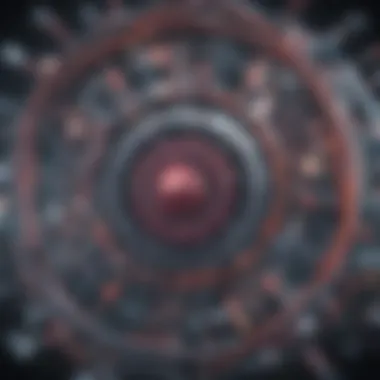
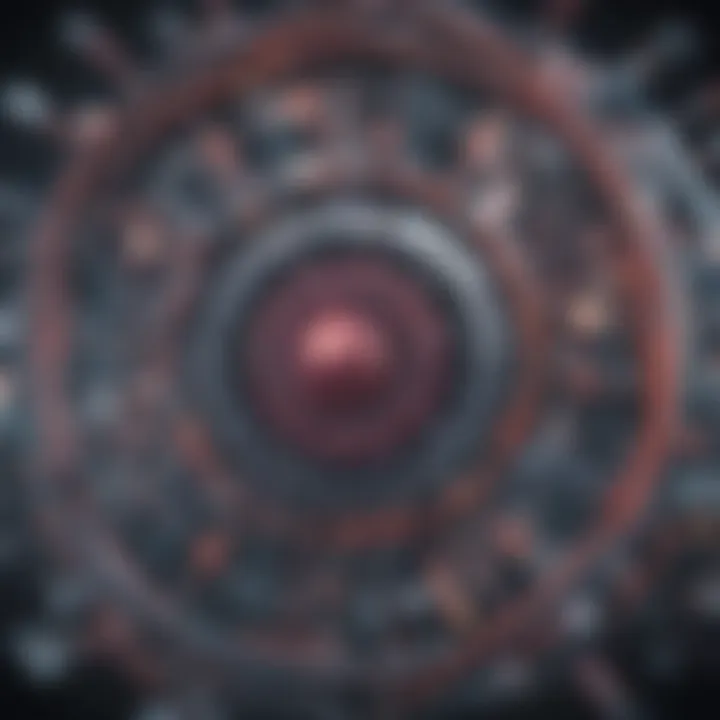
Intro
The field of cancer genomics has grown significantly in recent years. This growth is closely tied to the development of various databases that compile extensive genomic, transcriptomic, and proteomic information. These databases serve as vital resources for researchers and medical professionals aiming to explore the molecular intricacies of cancer. By analyzing the data within these databases, scientists can identify patterns that may lead to new treatment options.
Moreover, cancer genomics databases are instrumental in precision medicine, where treatment approaches are tailored to the genetic makeup of an individual's cancer. The implications of this shift towards personalized treatment strategies are profound, as they promise to improve patient outcomes while minimizing adverse effects.
In this article, we will highlight the importance of understanding these databases. We aim to provide a detailed overview of their structure, the types of data they hold, and the tools employed for data analysis. Additionally, ethical considerations and future directions in cancer genomics databases will be discussed. By breaking down the complexities involved, this article intends to balance clarity with depth, facilitating a thorough understanding for students, researchers, educators, and professionals in the field.
Research Overview
Summary of Key Findings
Cancer genomics databases play a critical role in advancing research by housing vast amounts of data accessible to the scientific community. Key findings from this overview indicate that:
- Significant insights into tumor biology can be derived from mining genomic data.
- Transcriptomic profiles provide information on gene expression that can inform treatment choices.
- Proteomic data helps elucidate the functional aspects of proteins involved in cancer progression.
These findings suggest that comprehensive data integration from various sources can foster more nuanced research inquiries and enable scientists to draw more informed conclusions.
Research Objectives and Hypotheses
The primary objective of this article is to:
- Examine the significance of cancer genomics databases in research.
- Detail the architectural design and data types these databases encompass.
- Discuss the analytical tools used to interpret the data effectively.
Hypotheses guiding this research include:
- The integration of multi-omics data will enhance our understanding of cancer.
- Utilization of these databases will improve precision medicine outcomes by allowing for targeted therapies tailored to genetic profiles.
Methodology
Study Design and Approach
This article uses a literature review approach, drawing on existing research and case studies to illustrate the various aspects of cancer genomics databases. No new empirical data is collected. Instead, emphasis is placed on critical analysis of current literature and interpretation of available information.
Data Collection Techniques
Data for this review was collated from reputable sources, including peer-reviewed journals and recognized databases such as The Cancer Genome Atlas (TCGA) and Genomics Data Commons (GDC). Key methods include:
- Reviewing published studies on database utilization in cancer research.
- Examining case studies that highlight successful outcomes stemming from the analysis of genomic databases.
- Synthesizing findings to draw broader conclusions on the impact of these databases on the field of oncology.
"The future of cancer treatment significantly depends on the advancements in cancer genomics databases and their analysis tools."
Through this structure, the article seeks to provide a thorough understanding of the intricate relationship between cancer genomics databases and modern oncology.
Prolusion to Cancer Genomics
Cancer genomics is a field that focuses on understanding the genetic basis of cancer. The significance of this area cannot be overstated. It provides crucial insights into how genetic variations influence tumor behavior, treatment responses, and disease prognosis. This article serves to highlight the various aspects of cancer genomics databases, emphasizing their role in research, clinical applications, and the future of oncology.
Definition and Scope of Cancer Genomics
Cancer genomics refers to the study of the genetic alterations that occur in cancer cells. This includes the examination of mutations, chromosomal rearrangements, and epigenetic changes. The scope of cancer genomics encompasses various types of data, including genomic, transcriptomic, and proteomic information. These data help researchers and clinicians understand the complexities of cancer biology and pave the way for personalized treatment options. As researchers continue to explore the genome of various cancers, the need for comprehensive databases that store and organize this data has become increasingly essential.
Key points in this area include:
- Identification of genetic markers can aid in early diagnosis.
- Genetic profiling helps in predicting treatment responses.
- Understanding tumor heterogeneity fosters the development of targeted therapies.
Importance of Genomics in Cancer Research
The integration of genomic information into cancer research has transformed our understanding of this disease. Genomics allows for a more nuanced view of cancer by characterizing it as a group of diseases rather than a single entity.
"The insights gained from genomics can lead to breakthroughs in treatment strategies, thereby enhancing patient outcomes."
Some critical aspects of genomics in cancer research include:
- Early Detection: Genomic biomarkers assist in identifying cancers at earlier stages when treatments are often more successful.
- Targeted Therapies: Insights from genomic studies enable the development of drugs that target specific mutations within cancer cells.
- Treatment Customization: By understanding a patient's unique genetic makeup, clinicians can tailor treatment plans that are more effective and less toxic.
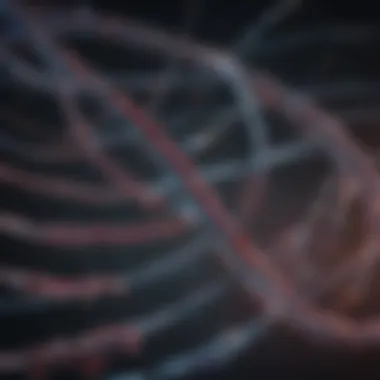
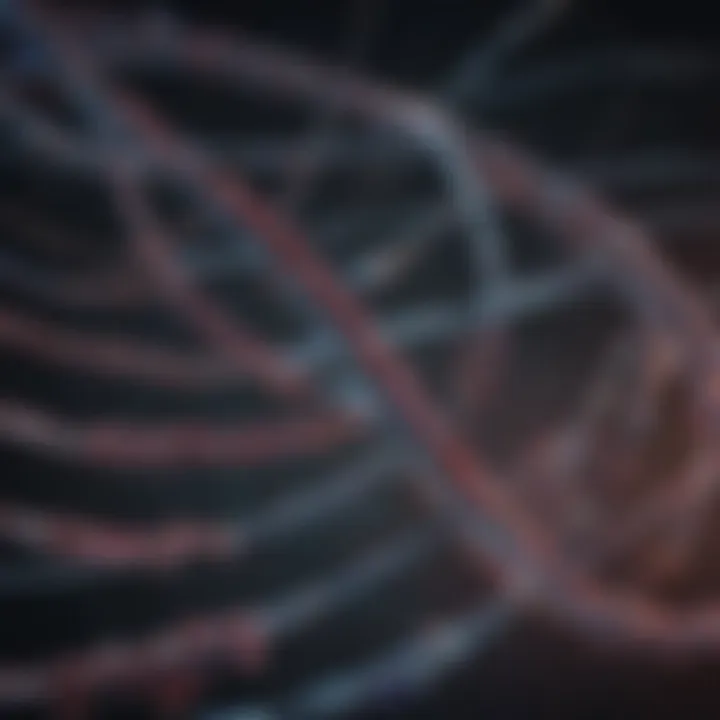
In summary, cancer genomics provides a foundation for advancing research and clinical practices, ensuring that the future of cancer treatment is more precise and informed.
Understanding Cancer Genomics Databases
Cancer genomics databases play a crucial role in the evolving landscape of cancer research and treatment. These databases provide a structured way to store, retrieve, and analyze vast amounts of genomic, transcriptomic, and proteomic data. The significance of understanding these databases derives from their capacity to facilitate discoveries that can lead to advancements in personalized medicine, targeted therapies, and better patient outcomes.
The increasing volume of data generated from various cancer studies requires effective tools for management and analysis. Several key databases, like The Cancer Genome Atlas (TCGA), International Cancer Genomics Consortium (ICGC), and cBioPortal, serve as foundational platforms that researchers and clinicians can rely on. Each of these databases has unique attributes that cater to specific research needs. By familiarizing oneself with their strengths, limitations, and data types, users can make informed decisions about which resource best suits their objectives.
Moreover, the architecture of these databases, encompassing their design principles and technologies, is essential for facilitating efficient data retrieval and integration. A comprehensive understanding of cancer genomics databases ensures that researchers can effectively harness the wealth of information available to drive innovation in cancer diagnosis and treatment.
Overview of Key Databases
TCGA
The Cancer Genome Atlas is one of the most extensive resources available for cancer genomics research. It offers a remarkable collection of genomic data from numerous cancer types. TCGA was established to create a comprehensive, coordinated research initiative to generate and analyze large-scale genomic datasets. Its main strength lies in the rich dataset that it provides, which has already contributed to significant discoveries in cancer biology.
A key characteristic of TCGA is its multi-dimensional approach; it integrates various types of data, including DNA sequencing, RNA sequencing, and epigenomic data. This integration allows for a more holistic understanding of tumor biology when studying the molecular underpinnings of cancer. However, some limitations include the complexity of data processing and the need for expertise in bioinformatics tools to effectively analyze the wealth of information it provides.
ICGC
The International Cancer Genomics Consortium is another premier database in the field of cancer genomics. ICGC focuses on the genomic characterization of different cancer types at a global level. It comprises coordinated efforts from various institutions to collect and analyze cancer-related genomic data. The database is dedicated to providing a platform for sharing research findings to enhance our understanding of cancer genetics.
Its primary feature is the categorization of projects worldwide, allowing researchers to access data that can lead to comparisons across different populations and cancer types. This global perspective is beneficial; however, challenges related to data consistency and integration can arise, especially when comparing heterogeneous datasets.
cBioPortal
cBioPortal is designed for intuitive data visualization and exploration. It aims to facilitate the understanding of complex cancer genomics data through easy-to-use interfaces. cBioPortal offers various tools that allow researchers to investigate mutations, copy number alterations, and clinical outcomes effectively.
The platform stands out due to its focus on interactive data tools, making it suitable for users with varying degrees of bioinformatics expertise. However, while powerful for exploratory analysis, the depth of available datasets may not match that of TCGA or ICGC, which contain more extensive genomic data compilations.
Types of Data Collected
Genomic Data
Genomic data forms the backbone of cancer genomics databases. It includes information regarding the DNA sequence variations that may contribute to cancer progression. The contribution of genomic data to cancer research is substantial, as it helps identify mutations, structural variations, and more. This data type is essential for understanding the genetic basis of cancer and can guide treatment decisions, especially in targeted therapies.
The key characteristic of genomic data is its capacity to provide insights into the heritable nature of cancers, making it a critical aspect for studies on familial cancer syndromes. However, the complexity involved in genomic data analysis demands high-quality sequencing techniques and bioinformatics skills, which can present barriers to effective usage for some researchers.
Transcriptomic Data
Transcriptomic data refers to the analysis of RNA transcripts produced by the genome under specific circumstances. It offers insights into gene expression levels, which can be altered in cancer. By studying transcriptomic data, researchers can identify which genes are upregulated or downregulated in different cancer types, which is vital for understanding tumor behavior.
This data type is essential because gene expression profiles can influence clinical outcomes, allowing for patients’ treatments to be tailored based on their tumor’s unique transcriptomic characteristics. One disadvantage, however, is the variability in RNA quality and quantity between samples, which can affect the robustness of the experimental findings.
Proteomic Data
Proteomic data encompasses the study of proteins produced by the genome, serving as a direct manifestation of gene expression. The importance of proteomic data lies in its ability to provide insight into the functional state of cells and how they may be altered in cancer. It can uncover novel biomarkers and therapeutic targets, aiding drug development and personalized medicine.
A unique feature of proteomic data is its capacity to capture post-translational modifications, which are crucial for understanding protein function and signaling pathways in cancer cells. However, challenges include the complexity of protein interactions and the need for sophisticated analytical techniques, which can limit access to proteomic analysis in some research settings.
Data Retrieval and Management
Effective data retrieval and management are vital for the utility of cancer genomics databases. Organizations must establish robust systems that allow researchers to access, share, and analyze data efficiently. The ability to manage large datasets while ensuring accuracy and integrity is fundamental. Furthermore, the evolution of data management technologies, such as cloud computing and data warehousing, offers new opportunities in the realm of cancer genomics. Reliable data retrieval APIs and user-friendly interfaces are crucial for fostering collaboration among researchers and ensuring that the insights derived from these valuable datasets are accessible and actionable.
Architecture of Cancer Genomics Databases
The architecture of cancer genomics databases is a fundamental aspect that underpins the functionality and accessibility of genomic data. Effective architecture ensures that these databases can handle large volumes of data while maintaining ease of use for researchers. A well-structured database serves not only as a repository but also as a resource that enhances research capabilities and facilitates the sharing of information across various platforms.
Database Structure and Design Principles
The design principles of cancer genomics databases revolve around scalability, flexibility, and user intuitiveness. A robust database structure includes various components such as schemas, tables, and relationships that allow for organized data storage.
- Normalization: This is crucial for reducing data redundancy. Proper normalization creates a structured pathway for data entry, minimizing the chances of inconsistencies.
- Modularity: By building databases in a modular manner, updates and maintenance become less cumbersome. This ensures that changes in one part of the database do not adversely affect its overall function.
- Accessibility: The design should prioritize accessibility for users, especially for non-tech-savvy researchers who need intuitive navigation and functionality.
Technologies Behind Cancer Genomics Databases
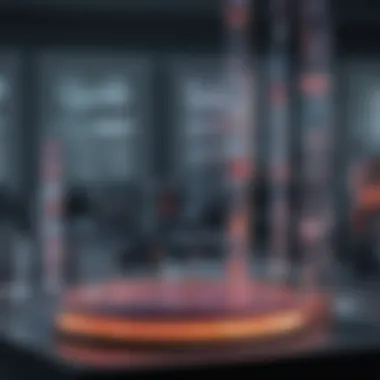
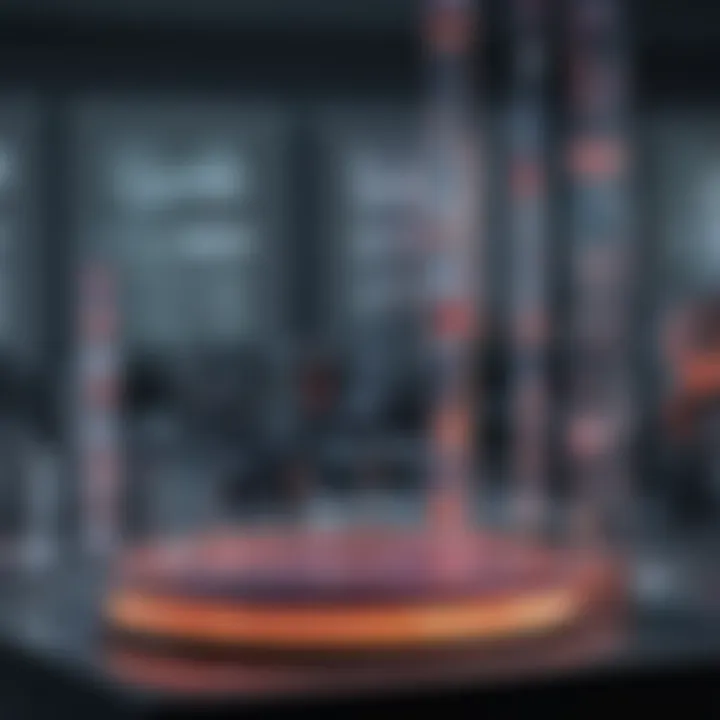
Cancer genomics databases leverage various technologies to support data management and efficiency. Two essential technologies are data warehousing and cloud computing. Each of these technologies offers unique benefits that contribute to the overall success of these databases.
Data Warehousing
Data warehousing is a critical technology in the management of large-scale genomic data. It enables the storage of vast quantities of data from various sources, which is essential for cancer genomics.
Key Characteristic: One of the main characteristics of data warehousing is its ability to consolidate data from multiple origins into a central repository. This integration of data allows researchers to analyze and compare information efficiently.
Benefit: This technology is particularly beneficial as it enhances data integrity and supports complex queries that are necessary for comprehensive analysis in cancer research. The ability to run analytics can lead to discoveries that are pivotal in understanding cancer genomics.
Unique Feature: A notable feature of data warehousing is the support for historical data storage. This allows researchers to track changes over time, which is crucial in the context of studying cancer evolution and patient response to treatments.
Advantages: Among the advantages are improved performance for query execution and simplified data reporting. However, the initial investment in data warehousing can be significant, and maintenance requires skilled personnel to ensure optimal functionality.
Cloud Computing
Cloud computing represents another technology revolutionizing the way cancer genomics databases are structured and maintained. It enables the dynamic storage and analysis of data.
Key Characteristic: The most significant characteristic of cloud computing is its scalability. Researchers can access virtually unlimited storage capacities, which is particularly important given the exponential growth of genomic data.
Benefit: Cloud computing offers a reliable option for collaborative research efforts. Multiple institutions can access the same data sets, facilitating cooperative studies that can lead to accelerated discoveries.
Unique Feature: The flexibility to deploy and scale resources on-demand ensures that data processing capabilities can match the volume of incoming data, reducing bottlenecks in research.
Advantages: The cost-effectiveness of maintaining cloud services compared to traditional infrastructures is noteworthy. Still, issues around data security and compliance with regulations remain concerns that researchers must navigate.
Analytical Tools and Techniques
Analytical tools and techniques are fundamental for extracting valuable insights from cancer genomics databases. The large volume of data generated through various genomic studies requires robust methods to analyze and interpret it. Without these tools, researchers may struggle to derive meaningful conclusions that can impact patient care and treatment strategies.
In the realm of cancer genomics, bioinformatics and statistical methods are indispensable. They empower researchers to make sense of complex datasets, identify patterns, and assess the significance of their findings. By employing these analytical techniques, oncologists and researchers can push the boundaries of cancer research and shape personalized medicine.
Bioinformatics Tools for Data Analysis
Bioinformatics tools play a critical role in the analysis of genomic data. They facilitate the processing, visualization, and interpretation of high-throughput data generated by technologies such as next-generation sequencing. Some key bioinformatics tools include:
- Gene Expression Omnibus (GEO): This repository stores gene expression data and provides tools for analyzing this data, which can reveal insights about cancer progression.
- Galaxy: An open-source platform that enables researchers to develop, run, and share bioinformatics analysis tools, making complex workflows more accessible.
- Bioconductor: A collection of R packages tailored for the analysis of genomic data. It provides statistical methods and visualization tools necessary for understanding large datasets.
These tools illustrate how bioinformatics can help in characterizing tumors, identifying biomarkers, and uncovering pathways critical for cancer development. Moreover, integrating various bioinformatics tools can allow a comprehensive analysis, combining genomic, transcriptomic, and proteomic data in one study.
Statistical Methods in Genomics
Statistical methods are equally important in the analysis of cancer genomics data. They provide a framework for validating hypotheses and ensuring the reproducibility of findings. Several statistical techniques are commonly utilized in genomics:
- Survival Analysis: Techniques such as Kaplan-Meier and Cox regression are widely used to analyze the time until an event occurs, such as disease progression or patient survival. This informs treatment efficacy and patient prognosis.
- Differential Expression Analysis: Methods like DESeq2 or EdgeR are employed to determine which genes are expressed differently between cancerous and non-cancerous tissues. These findings support the discovery of potential therapeutic targets.
- Machine Learning: Algorithms can identify complex patterns in genomic data, aiding in classification tasks or predicting treatment responses. This innovation drives advances in precision medicine, allowing for treatment tailored to individual patient profiles.
Statistical analysis is complemented by visualization techniques, which help present complex data in interpretable formats. Tools such as R and Python libraries provide options for creating plots and charts that capture vital information succinctly.
A thorough understanding of these analytical tools and techniques will provide researchers with the necessary framework to interpret genomic data effectively, enhance collaborative research efforts, and ultimately contribute to better patient outcomes in oncology.
The integration of bioinformatics and statistical methods forms the backbone of modern cancer research. They not only facilitate data analysis but also encourage the validation and reliability of findings. As these technologies continue to evolve, their influence on cancer genomics databases will undoubtedly expand.
Applications of Cancer Genomics Databases
The applications of cancer genomics databases play a critical role in modern oncology. These databases serve as comprehensive repositories of genetic information that can assist in understanding cancer at a molecular level. They bridge gaps between research and clinical practice, facilitating advancements in treatment strategies and improving patient outcomes.
Precision Medicine and Personalized Treatment
Precision medicine aims to tailor treatment based on the unique genetic profile of each patient. Cancer genomics databases are essential in this process as they provide the necessary data to identify specific mutations and variants associated with individual tumors. By analyzing these databases, oncologists can pinpoint which therapies may be most effective for a patient.
Moreover, genomics data helps in predicting responses to drugs, minimizing adverse effects, and developing treatment plans that align with the patient’s cancer characteristics. For instance, targeted therapies can be selected based on a patient’s specific lung cancer mutation, making the treatment more effective and efficient. The integration of data from databases such as The Cancer Genome Atlas (TCGA) greatly enhances the precision medicine approach, leading to better outcomes.
Drug Development and Clinical Trials
Cancer genomics databases are pivotal in the drug development process. By offering a vast amount of genetic data, these databases help researchers understand the underlying biology of different cancer types. New drug candidates can be designed by identifying specific targets derived from genomic alterations in tumors.
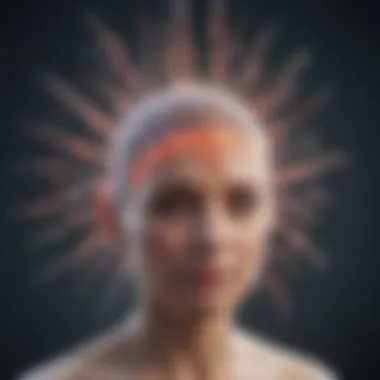
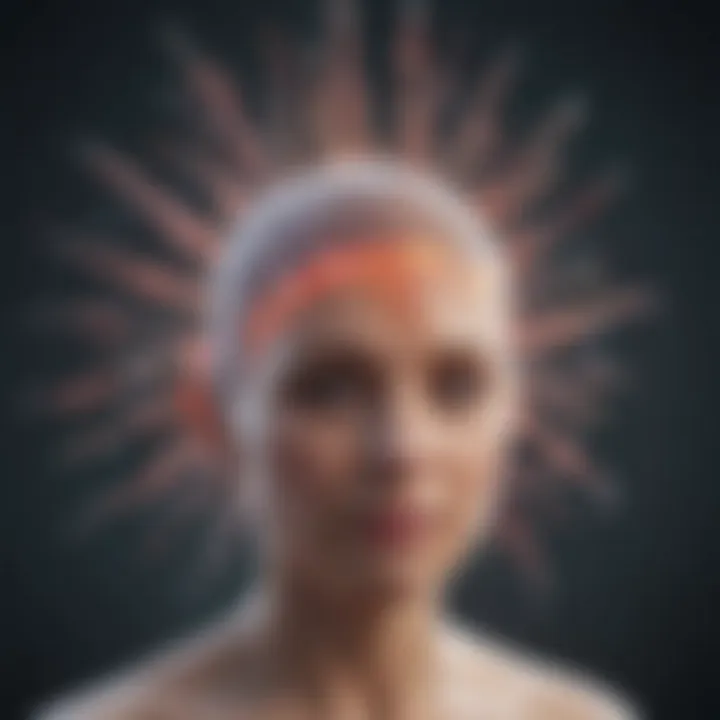
Furthermore, clinical trials increasingly rely on data from these databases to identify suitable patient populations for testing new therapies. For instance, genomic profiling of patients can ensure that only those with relevant mutations are enrolled in trials, increasing the likelihood of success. This focus not only accelerates the development of new drugs but also enhances the safety profile of new therapies, as they are tested on genetically appropriate cohorts.
Disease Progression and Prognostic Indicators
Understanding how cancer progresses is another crucial application of cancer genomics databases. These databases contain information on not just mutations, but also genomic alterations and their association with disease evolution. This can inform prognostic indicators that help predict patient outcomes.
For example, specific genetic markers may signal a higher risk of metastasis or resistance to treatment. By analyzing these markers within the genomic datasets, clinicians can offer more accurate prognoses. Such insights may also inform decisions regarding the intensity of treatment or the need for closer monitoring.
In summary, the applications of cancer genomics databases extend far beyond mere data storage; they are integral to the future of cancer care. They enhance precision medicine, accelerate drug development, and improve understanding of disease dynamics, underscoring the significance of these resources in the clinical landscape.
Ethical Considerations in Cancer Genomics
The rapidly evolving field of cancer genomics presents numerous opportunities for enhancing personalized medicine and advancing cancer research. However, these advancements come with significant ethical considerations that must be thoroughly addressed. Ethical issues in cancer genomics are essential, as they impact both individual patients and the broader research community. Principles such as patient autonomy, data privacy, and the responsible use of genetic information are fundamental to the integrity and progress of oncology research.
The high sensitivity of genomic data elevates the need for stringent ethical standards. Researchers and institutions must ensure that the use of genomic information does not violate patients' rights or lead to discrimination. By prioritizing ethical considerations, researchers can foster trust and collaboration with participants, ultimately enhancing the quality of research and its applicability in clinical settings.
Patient Consent and Privacy Issues
In cancer genomics, obtaining informed consent from patients is of paramount importance. Patients need to fully understand what their participation entails, particularly regarding how their genomic data will be used and shared. The consent process should be comprehensive and transparent, allowing patients to make informed choices about their involvement in studies.
Moreover, privacy issues are critical. Genomic data, due to its uniqueness, can reveal sensitive information about individuals and their families. Researchers must implement rigorous protocols to ensure that identifying information is protected. This includes using anonymization techniques and ensuring that data sharing complies with regulatory standards. Other considerations include:
- Data Ownership: Clarifying who owns the data can prevent future disputes and foster trust among participants.
- Withdrawal Rights: Patients should retain the right to withdraw their data from research at any time, reinforcing their autonomy.
In short, balancing the potential benefits of cancer genomics with the need for ethical stewardship is crucial for maintaining public trust and advancing medical science responsibly.
Data Security Measures
Data security is another vital component in the ethical landscape of cancer genomics. As the volume of genomic data continues to grow, safeguarding this information becomes increasingly challenging but essential. To protect sensitive information, institutions must adopt comprehensive data security measures, including:
- Encryption: Employing strong encryption techniques to protect data both at rest and in transit can significantly reduce the risk of unauthorized access.
- Access Controls: Strict access controls should be established to ensure that only authorized personnel can handle sensitive data.
- Regular Audits: Conducting audits frequently can help identify vulnerabilities and enhance the overall security posture of the data management systems.
Additionally, education and training about data security best practices for all personnel involved in managing genomic data are critical.
"Proper ethical oversight and security measures not only protect individual rights but also enhance the overall legitimacy of cancer genomics research."
Future Directions in Cancer Genomics Databases
The future of cancer genomics databases promises a landscape filled with innovation and enhanced capabilities. These databases are central to modern oncology. They hold vast amounts of data from various sources, and that data is critical for advancements in personalized medicine. Exploring future directions offers insights into potential breakthroughs, challenges, and how these databases will continue to evolve.
Next-Generation Sequencing and Beyond
Next-generation sequencing (NGS) is a game changer in the field of cancer genomics. It offers a level of detail that was previously unattainable with traditional methods. As NGS technology improves, its incorporation into cancer genomics databases will likely lead to more comprehensive datasets.
- Increased Accuracy: NGS is more precise and can identify mutations that may not be noticed with conventional sequencing methods.
- Cost Reduction: As NGS becomes less expensive, more researchers can utilize it, expanding the dataset available for study.
- Rapid Processing: Advances in computational power mean that data generated from NGS can be analyzed quicker, facilitating faster research outputs.
The integration of next-generation sequencing into cancer genomics databases may accelerate the understanding of tumor heterogeneity and lead to more effective treatment strategies.
As researchers continue to harness the power of NGS, databases will need to adapt to store, process, and analyze the influx of genomic data effectively.
Integrating Multi-Omics Approaches
Integrating multi-omics approaches into cancer genomics databases represents another significant direction. This method combines genomics, proteomics, metabolomics, and transcriptomics, providing a systems biology perspective.
The benefits of this integration include:
- Holistic View of Cancer: By analyzing different omics data together, researchers can better understand cancer as a complex system rather than a singular genetic issue.
- Informed Decision-Making: Physicians can make more informed decisions regarding treatment based on a comprehensive overview of patient data.
- Discovery of Biomarkers: Multi-omics can facilitate the identification of novel biomarkers for diagnosis and prognosis, improving patient outcomes.
Looking ahead, integrating multi-omics data into cancer genomics databases poses challenges, such as data heterogeneity and the need for advanced analytical frameworks. Addressing these will be vital for realizing the full potential of this approach in cancer research.
The End
In the realm of cancer research, cancer genomics databases are fundamental. They consolidate vast amounts of information, which helps scientists and clinicians in better understanding the complexities of various cancers. This article has highlighted how such databases contribute not only to research but also to clinical practices.
Summarizing the Contribution of Databases to Cancer Genomics
Cancer genomics databases serve as a crucial linchpin in modern oncology. They offer a wealth of genomic, transcriptomic, and proteomic data across multiple cancer types. The integration of this data facilitates several significant contributions:
- Enhanced Understanding of Cancer Biology: By aggregating information, these databases enable researchers to decode the genetic underpinnings of various cancers. This understanding paves the way for identifying biomarkers that can lead to earlier diagnosis and effective treatments.
- Precision Medicine Development: These databases are indispensable for developing precision medicine strategies. They assist in correlating specific genetic mutations with treatment responses, helping to tailor therapies to individual patients.
- Facilitation of Collaborative Research: Cancer genomics databases create a platform for collaboration among researchers globally. Through shared data, the scientific community can work together to address unanswered questions in cancer treatment.
- Support for Clinical Trials: Cancer genomics databases provide essential data that can help design better clinical trials. They help in identifying suitable patient populations who may benefit from experimental therapies, enhancing the chances of trial success.
"The integration of data in cancer genomics databases drives innovation in both research and treatment options."
The significant advancements in cancer treatment rely heavily on the data stored in these databases. As research progresses, the scope of these databases is likely to expand, offering more precise insights into the genetic makeup of cancers. This ensures that the future of cancer care remains intertwined with genomics, thereby improving outcomes for patients.